Author: Robert Walker, contact email robert@robertinventor.com
Is NASA’s novel biosafety reasoning for Mars samples safe enough? How to play to NASA's strengths and keep Earth's biosphere totally safe with more science return, at less cost, and with a better fit for NASA's long-term objectives
[MID EDIT]
[MID EDIT - mainly minor edits now but occasionally still make major changes - also some cites to add / check / move to the end]]
[in process of reducing repetition as much as possible - with brief summaries and links to other sections when the same material has to be touched on in a new context in the book]
Last modified:
Citation list at end
The option to move the citation list to the end is unselected for new visitors to the page, as it lets you click straight through to the linked paper from the cite and slightly reduces page load time.
Test citation: (Sagan, 1973, The Cosmic Connection - an Extraterrestrial Perspective)
Note. This was originally planned as a single paper but is now book length. I may use this as a basis for a shorter paper or papers to submit to journals. This web page is designed to be easy to navigate so you can quickly navigate to any section of interest using the menu, skip to next, and search features.
BOOK: To submit to academic publishers - NOT YET PEER REVIEWED and need to start public and general scientific dialogue on these issues early before it can be published
This book has NOT yet been sent for peer review. The plan is to first notify NASA and ask for comments as advised by the CEQ, as a courtesy, as an attempt at bridge building, and to give them an opportunity to reply if they wish. It will likely be some months more before I submit it to peer review as it covers a wide range of disciplines and I plan to spend some time contacting the experts I cited in those various topic areas to ask if I have summarized their work properly and for feedback if they are interested to comment. This might well lead to revisions and additions. Then as a first time author writing my first work on astrobiology I expect it to take some months to years to get it published.
However time is not on our side, unless NASA is correct in its novel non-peer-reviewed biosafety plans that a biosafety level 4 facility is already adequate to contain any conceivable alien life from Mars. In particular according to all the peer-reviewed literature on the topic, work on a more elaborate Sample Receiving facility should have started already to be ready by 2033 and the timeline is tight to finish it in the 2030s.
If Carl Sagan and experts who worked on the Apollo Lunar Receiving facility were correct that we are not able to contain alien life after a lab leak in a lab run by human technicians, it is not certain that we can return unsterilized samples to Earth. Also, some decisions may need to be made in the next few years if the outcome of a review of NASA's plans is that the design of the spacecraft it sends to Mars in the late 2020s need to be changed.
See below:
The planetary protection experts say this dialogue and airing of the issues should have started long ago, as soon as NASA begun on serious planning over a decade ago. So it is a matter of doing what I can from my side to help get the process going. This research effort can't succeed without that broad acceptance.
See below:
While stressing the urgency for a decision from the point of view of science return, cost, and NASA policy, it is important in all our public communications to explain clearly
- we are NOT talking about a high risk mission
- Earth will be kept safe.
This is about potential low risk but a low risk of large-scale harm. Margaret Race's house analogy of a smoke detector to help protect against house fires is a wonderful tool here for public communication. We all know that house fires are very low risk, yet we also all understand the importance to take precautions to protect our houses against them.
See below:
Also we have many precautions in place to protect Earth's biosphere in the form of legal protection for invasive species, etc. In addition, the general public care deeply about the safety of our biosphere and have far more awareness of the importance of protecting it than in the time of the Apollo missions in the 1960s.
NASA currently relies on its novel non-peer-reviewed conclusions to say that it already fulfills those obligations:
- Why NASA can't ensure negligible public impacts from alien microbes using standard biosafety level 4 procedures: how a biosafety lab differs in requirements from a Mars Receiving Facility
- NASA's novel deduction that there would be NO significant ecological impacts, based only on its assessment that samples are UNLIKELY to pose a risk of significant ecological impact
If a review of NASA plans overturns these conclusions, this incurs many obligations on both NASA and ESA to protect Earth's biosphere.
- An impact statement that recognizes a low risk potential for large scale harm to the environment and public health has to consider impacts on the Great Lakes, or oceans, or invasive species, and many executive orders are triggered
- Obligations for ESA countries based on the conclusion of the ESF study from 2012 on low risk of large-scale harm
So, I won't wait for publication to share this draft book. Early in 2024, and depending on responses from NASA and experts I contact early on, I plan to encourage sharing of this preprint and move to gradual publicity of this draft book to draw attention to these issues. I think it is possible this book may get significant attention at that stage. The aim is to help start the process of public and general scientific engagement in discussion of NASA's novel biosafety plans as much as I can from my side.
You can get a pretty good idea of this draft book, if interested by reading from the abstract through to the 12 pitfalls and the roadmap in 16 steps, a total of about 16,350 words or nearly 55 minutes at 300 wpm.
Or for a shorter intro, the short guide here gives a glimpse of the book and where it's headed:
Abstract (299 words)
START ABSTRACT
Some low-risk scenarios of martian microbes may have large-scale effects on human health or the biosphere. The problem is, NASA shut down its planetary protection office for a Mars sample return in 2017, losing its global leadership in this field.
NASA’s current biosafety plan relies on a novel argument for harmlessness of martian life: that it can get here better protected in a martian meteorite than a sample tube. However, dust or dirt can never get here, and any martian microbes may not survive ejection or transit between planets. Also, in some scenarios, Mars is habitable and has martian microbes; life from Mars can spread on Earth; and martian life can harm humans, or our biosphere.
NASA plans a Biosafety Level 4 facility to contain its samples in 2033. However, the ESF’s 2012 size requirements to contain ultramicrobacteria are beyond capabilities of best available air filter technology.
Any lab with human technicians needs quarantine for lab leaks. But this can’t keep out diseases with lifelong symptomless carriers, harmful fungi or mirror life.
We can sterilize Perseverance's samples, with virtually no geological effect by applying the same dose of ionizing radiation they likely already accumulated on the Martian surface, in a shorter time. We can enhance astrobiological value by adding bonus samples of salts, dust, dirt, atmosphere and a pebble, collected in clean containers.
We can keep Earth 100% safe (no realistic risk) by studying unsterilized samples in a satellite resembling NASA’s 2016 proposed Europa in situ life detection lander, above GEO.
We can keep Mars clean until we know if it is safe to land humans, by using telerobotic explorers with modern components that withstand an hour of sterilizing heat at 300°C before deployment. The martian moons are a challenging intermediate goal, to study Mars via telepresence from orbit.
END ABSTRACT
Hover mouse over left margin for floating table of contents and other options for this online preprint
For this online paper, I use a short form of citation inlined like this (Hand et al., 2017, Report of the Europa Lander Science Definition Team :
xi). This makes it easier to click through to the cite when reading online, without losing
your place in the page. It gives the author, date, and a direct hyperlink to the online paper and
adds the page number, table, or figure number, etc., as appropriate
It is easy to convert these short form citations to the long form in any citation format you prefer
(e.g. with the google scholar button),
Hover your mouse over the left margin of the page to see a floating table of contents of all the section titles.
The skip to next / back links give another way to go through the paper quickly. You can read the title of each section then read on to find more or click next. The top level next lets you skip through the top lively headers like reading an abstract of the paper.
For my background see Supplementary information section under:
Headers in dark blue are hyperlinked to themselves - this lets you copy / past them into other pages or emails to link to them. You can use the "copy header as link with minimal styling" to copy just the link itself, not the header and without text colour or the text size. This is useful if you want to link to it from another page or an email etc. Use Ctrl + click for no styling (though browsers often add their own styling as well as programs you paste the link into). The minimal styling sets the linked text to underline with a dark blue color. This only works for headers that I have added anchors to and linked to themselves - will do that to them all when finished but if the button shows or the header is coloured dark blue it's okay.
Search this page
Search this page for (enter text to find)
Search for complete word only (if only one word entered):
Search for exact phrase
[search ignores punctuation and to avoid very long search results, search term must be at least three letters]]
This book is designed to be widely accessible
I have written this book to be as accessible to everyone as I can – theologians, philosophers, lawyers, politicians, decision makers, the general public and autistic people. I do this by careful use of vocabulary and grammatical structures, but never with loss of precision in the meaning of the text. I wish this choice to survive through to the final version of this book or any papers if possible.
Examples:
- I use the most widely accessible vocabulary available to convey the desired meaning
- I replace technical by non-technical terms when it can be done with no loss of precision
- I use non-scientific terms, non-technical terms generally, and non-mathematical language if it is available with the same precision.
Example of using non-technical terms when there is no loss of precision:
- One million instead of 106
Where there is no ordinary language equivalent, I explain the term in ordinary language as far as is possible when it is first introduced. I may later use a shorter definition of the same term as a reminder.
I also make the book maximally accessible to neurodivergent such as autistic people. The most obvious changes are that sentences and clauses tend to be shorter and more self contained than in most academic papers. The pastel background shade is for this reason too, and the use of text on images and bolding of some key words and sentences in quotes. These also tend to help with many neurotypical readers so it's a "win win" situation. For details see Supplementary information under Making this book maximally accessible to neurodivergent people such as autistic people
This book is written mainly in British English but many of the quotes are in American English and I use some American conventions. As an example, in particular, British authors usually use the plural for organizations for instance I would normally use "they" to refer to things NASA do but in the USA it is normal to use "it" to refer to things NASA does. I use the American convention here to make it more accessible to American readers. Of course if this book is published by an academic publisher, these matters would be sorted out according to the publisher's manual of style.
Introduction
NASA has achieved extraordinary things in both robotic and human exploration. For many decades, NASA was the only space agency able to explore Mars, and even today nearly all our discoveries are due to its robotic explorers and satellites. It is also the first agency globally to prepare an Environmental Impact Statement for a Mars Sample Return. We can expect other space agencies and private space to model their own plans on NASA's work.
However NASA lost its in-house excellence on planetary protection for a Mars sample return when it closed down its planetary protection office in 2017 (Voosen, 2017, With planetary protection office up for grabs, scientists rail against limits to Mars exploration), and has never been an agency responsible for oversight of biosafety or public health. It also closed down its interagency panel in 2006 (SSB, 2018, Review and Assessment of Planetary Protection … : 26 - 27). and so its plans are developed independently of the CDC, DoA or other agencies that could have advised it on biosafety measures.
To develop a credible biosafety plan, it's essential for NASA to maintain scientific integrity, and to comply with NEPA requirements for its Programmatic Environmental Impact Statement (PEIS) (NASA, 2023. Mars Sample Return final PEIS). One of those requirements is that the disciplines of the preparers should be appropriate to the assessment they are required to make (§ 1502.6).
When NASA closed its planetary protection office, it lost its only expert on the planetary protection literature for a Mars sample return mission. When NASA closed down the interagency panel, it also lost direct access to expertise on how to contain a large-scale risk to human health or to protect the environment from invasive species, or to design, build and operate a biosafety laboratory.
NASA has never been an agency responsible for running a biosafety lab or containing a pandemic or containing invasive species. This mission plan is intended to prevent any possibility of a future pandemic in circumstances that would be challenging for the CDC or NIH. It needs plans to contain not only terrestrial pathogens but a pathogens based on any conceivable alien biology. Similarly it needs to contain invasive microbes based on any alien biology, in a way that tries to avoid any assumptions based on our limited knowledge of only one genesis of life.
It would be no surprise to find issues in such an ambitious plan. Any issues we find don't reflect on individual competence of NASA engineers and scientists or mission planners or administers at any level. Rather they are likely to be more systemic, due to an agency with excellence in space missions trying to use its engineering and scientific excellence to devise a biosafety plan when this is not its natural area of competence. We will identify some systemic issues that go back to the time of Apollo in this article.
Carl Sagan was enthusiastic about space exploration and would have loved to be involved in the first human mission to Mars. However he also valued Earth's biosphere and its inhabitants greatly. He said we shouldn't take even a small risk of large scale effects on human health from any life they might bring back from Mars, the same issue as for a Mars sample return.
Carl Sagan put it like this:
Text on graphic: Carl Sagan (pioneer in planetary protection - first paper in 1960)
[his first paper on planetary protection is (Sagan, 1960, Biological contamination of the Moon)]“I, myself, would love to be involved in the first manned expedition to Mars. But an exhaustive program of unmanned biological exploration of Mars is necessary first.
“The likelihood that such pathogens exist is probably small, but we cannot take even a small risk with a billion lives.”
(Sagan, 1973, The Cosmic Connection - an Extraterrestrial Perspective)
[I provide text captions for the graphics in this open letter for visually impaired readers]
In that photo Sagan is standing in front of a model of the Viking lander, which remains the gold standard for the most carefully sterilized mission ever sent into space.
On a topic like this it is inevitable that readers will speculate about the author's perspective and motivations. I am with Sagan on this. I am as keen on astronauts in space as anyone, have been since I was a kid, but it has to be safe. Safe for Earth's inhabitants (humans and all the other creatures we share this Earth with) and for our biosphere.
All the modern major peer reviewed studies on a Mars sample return would concur with Carl Sagan's assessment of risk in his book published in 1973. They refer to a risk of large-scale harm to human health and the environment although experts consider the risk to be likely very low (Ammann et al., 2012, Mars Sample Return backward contamination–Strategic advice and requirements : 20, 24, 58) (SSB, 2009, Assessment of planetary protection requirements for Mars sample return missions : 48)
See below:
If you have already read the next two sections which I link to at the top of the page::
- Guide to this book: issues and solutions
- Time is not on our side according to the peer reviewed literature - unless NASA is correct in its novel non-peer-reviewed biosafety plans
you may want to skip to the section after them:
Guide to this book: issues and solutions
For an overview of the structure of this book hover the mouse over the left margin of the page to see the table of contents.
You can get a good first idea of this book by reading from the abstract through to
That's a total of about 16350 words or nearly 55 minutes at 300 wpm.
This short guide is intended as a first glimpse at where this book is headed with useful links to some of the main sections.
Why NASA no longer has world experts on planetary protection for a Mars sample return mission:
Four novel findings in NASA's PEIS which are also commonly believed to be valid and so might be used in similar statements by other agencies:
- How NASA's central finding that any harmful life can get here better protected in meteorites is rebutted by BOTH of its main cites for it
- NASA's uninhabitable Mars finding rebutted by its own cite, and by NASA's own plans to test for life in its samples
- NASA's Biological Safety Report's finding of near zero risk that martian life can harm us rebutted by soil pathogens like tetanus and aspergillus fumigatus not adapted to any terrestrial host
- NASA's Biological Safety Report's finding that any martian microbe would not be viable on Earth rebutted by the only plausible Mars analogue in its cite: Planococcus Halocryophilus
Rummel et al. first rebutted all four of these findings in 2000, and NASA's PEIS supplied no new information to change the conclusions, indeed showed no awareness of previous rebuttals:
Two novel conclusions:
- Why NASA can't ensure negligible public impacts from alien microbes using standard biosafety level 4 procedures: how a biosafety lab differs in requirements from a Mars Receiving Facility
- NASA's novel deduction that there would be NO significant ecological impacts, based only on its assessment that samples are UNLIKELY to pose a risk of significant ecological impact
For the effect of all this on the limited scope of the PEIS from the outset:
- NASA's novel conclusion that any effects would be local to the Utah Test and Training Range, and not significant
- Impact of limited scope for tier 1: NASA only publicised its PEIS and scoping meetings in newspapers local to the Utah Test and Training Range and Kennedy Space Center
Consensus of all the studies of a likely low risk but of large scale and protracted effects:
Smoke detector analogy. The analogy of a house fire is an excellent way to communicate the idea of a likely low risk but a low risk of potentially major effects requiring adequate and sensible precautions
NASA itself says in its responses to public comments that that large-scale, even unprecedented harm is a possible outcome of the mission which it has to prevent:
This may seem to contradict NASA's own finding that any impacts would not be significant. The reason is its novel deduction we already mentioned which is not standard risk assurance reasoning and is not cited to anyone else making that deduction but is NASA's own non peer reviewed reasoning in the PEIS itself:
Need for peer review of NASA's novel findings and novel conclusions:
For the need for early public engagement emphasized in all the main studies on a Mars sample return mission:
And current near impossibility of any engagement:
For a summary of the public responses to NASA's PEIS see:
Issues with sample containment which NASA deferred to tier 2, which should be for matters not ripe for discussion at tier 1 and not needing public responses:
- Why NASA can't rely on HEPA filters for the ESF recommendation to contain 100% of ultramicrobacteria
Two illustrative scenarios to show why lab leaks are such an insoluble question for alien life if we don't know what it is.
Why NASA can't rely on standard biosafety level 4 procedures to contain the samples and why it is not appropriate to defer such considerations to tier 2.
A solution to these containment issues proposed in this book:
- Life detection with miniature instruments in a teleoperated satellite above GEO: an alternative that plays to NASA's strengths, modelled on its proposed in situ Europa life detection lander
- This proposed alternative submitted to NASA under NEPA uses a centrifuge for Mars gravity in an excellent Mars simulation chamber, which also means instruments can operate under Mars gravity
- “Earth Entry Vehicle” can be converted into an “Above GEO Insertion Vehicle” by replacing aeroshell with extra fuel for its ion thruster and returned to a safe orbit tens of thousands of kilometers above GEO - or perhaps to the Earth Sun L2 point like the James Webb telescope
- Modern miniature life detection instruments that could fly to above GEO, or to Mars
- A small step for NASA, a giant leap for planetary protection: the case for a miniature telerobotic lab above GEO
- How a miniature life detection lab above GEO can overcome the technological uncertainties of a fully telerobotic biosafety lab on Earth, at significantly lower cost, with a significantly higher technological readiness level and more robust biocontainment
Need for bonus samples of interest to astrobiology collected in 100% clean containers added to the NASA Sample Retrieval Lander for first steps in any meaningful searches for past life, or indeed present day life unless surprisingly abundant in Jezero crater.
Longer term vision of a way to achieve NASA's end goal throughout the solar system in a more robust fashion that works no matter what we find on Mars and also lets us explore Mars rapidly by telepresence with telerobotic avatars while keeping Mars's sensitive "special regions" if any safe from being overrun with terrestrial microbes:
- A more resilient way to achieve NASA's end goal of humans throughout the solar system with an intermediate goal to explore Mars from orbit, with In Situ Resource Utilization (ISRU) on its moons
- How to do a rapid 100% heat sterilized unmanned biological exploration of Mars using a simpler version of NASA's Venus HOTTech
Supplementary Information section which includes material that is too specialist to be included in the main body of the book.
Summary of some of the NEPA legal issues with this PEIS (Programmatic Environmental Impact Statement):
This book does NOT recommend anyone takes out legal action against NASA on these issues.
Instead it recommends NASA withdraws the PEIS and starts again:
- withdraws this PEIS to fix all the NEPA issues it identifies,
- that any new plans are based on peer reviewed science and
- that it follows the NEPA processes carefully next time since the requirements are there for a reason.
This section focused on the NEPA requirements for a PEIS is intended as constructive criticism to ensure a future better PEIS done properly under NEPA. It concludes that there are reasonable alternatives to consider that have potential to resolve all these issue with more science return and potentially at less cost.
If the plan is not withdrawn, this book recommends urgent peer review of NASA's novel findings and conclusions in the PEIS by a body of high reputation such as the National Academy of Sciences in the USA or the European Science Foundation. This review is urgent because of the risk of high costs and time delays for the mission if the review isn't done until the 2030s.
A thorough academically sound review can be expected to REVISE all of these novel deductions and conclusions in NASA's current PEIS:
- four novel findings ❌
- two novel conclusions ❌
- novel risk assurance deduction of no significant ecological impact from a low risk of large-scale and protracted ecological impact ❌
Also these novel decisions
- that any environmental effects would be restricted to the neighbourhood of the landing sites in the sands of the Utah Test and Training Range ❌
- that the public only needs to be consulted locally to the UTTR and in the Kennedy Space Center ❌
- that samples can be contained with standard Biosafety level 4 protocols❌
- that design of a Mars Receiving Facility is not ripe for discussion until tier 2. ❌
- that ESF requirement to contain 100% of particles at 0.05 microns and upwards didn't need to be mentioned because HEPA filters fulfill this requirement already ❌
- that lab leaks can be contained with standard biosafety level 4 protocols ❌
- that life detection can only be done in terrestrial labs ❌
- to prescreen all proposals that look for life in situ or in orbit as non substantive ❌
- that unsterilized samples must be tested for safety ❌
[no safety testing needed for samples studied in orbit or on Mars or sterilized before they reach Earth's biosphere]
See: If all samples returned to Earth are sterilized the main biosafety challenge for NASA is to ensure the level of sterilization is adequate in an open, transparent and interdisciplinary way - that safety testing can only be done on Earth in terrestrial labs ❌
[no testing can ever ensure safety of the Perseverance samples for ultra low concentrations of martian life; there is no substitute for Sagan's exhaustive program of unmanned astrobiology on Mars]
See: Why the COSPAR Sample Safety Assessment Framework can't be used to prove safety of unsterilized Perseverance samples
[❌ indicates not expected to survive peer review]
This book concludes that such a review is inevitable at some point, if NASA continues with its current plans. A decision to do such a review early would greatly benefit the science return.
This book concludes that such a review is most urgently needed for ESA and NASA's preparations for the Mars Ascent Vehicle, the Earth Return Orbiter and the Sample Retrieval Lander, due to be launched in the late 2020s, as the design of these spacecraft and their objectives may well be changed in a peer reviewed biosafety plan for Earth's biosphere. An independent review has already concluded that the earliest Launch Readiness Dates are in 2030 for both of these (Figueroa et al., 2023. Mars Sample Return (MSR) Independent Review Board-2 Final Report : 16).
As for returning the samples to a terrestrial lab: if this book is correct in its identification of its many extra challenges, it is a tight timetable to have it ready by 2040, if such a solution is possible at all with near future technology. It may still be possible to return the samples to the miniature life detection lab above GEO by the early 2030s if the design of the spacecraft can be adapted to this new approach quickly enough.
This book also concludes that this is a pivotal decision for our civilization. Based on technology we only developed in the last 5 years or so we now have the option to choose to protect the biospheres of both Mars and Earth 100% until we know better:
Conclusions:
Time is not on our side according to the peer reviewed literature - unless NASA is correct in its novel non-peer-reviewed biosafety plans
Based on the conclusions in this book we can see that it is important to resolve these issues quickly. According to the sample return studies, and this book supports and concurs with this conclusion:
- If NASA's biosafety plans don't stand up to scrutiny by the broader scientific community and the general public, this research effort cannot succeed, as simple as that.
See below: Broad acceptance by scientists and general public is essential
NASA depends on novel reasoning for their decision to postpone all considerations of biosafety containment measures for a Mars Sample Receiving facility to tier 2, after the process of public engagement with its plans.
Repeating some links from the previous section :
Two novel conclusions:
- Why NASA can't ensure negligible public impacts from alien microbes using standard biosafety level 4 procedures: how a biosafety lab differs in requirements from a Mars Receiving Facility
- NASA's novel deduction that there would be NO significant ecological impacts, based only on its assessment that samples are UNLIKELY to pose a risk of significant ecological impact
For the effect of all this on the limited scope of the PEIS from the outset:
- NASA's novel conclusion that any effects would be local to the Utah Test and Training Range, and not significant
- Impact of limited scope for tier 1: NASA only publicised its PEIS and scoping meetings in newspapers local to the Utah Test and Training Range and Kennedy Space Center
- If NASA's non-peer-reviewed conclusion is correct: that a normal biosafety level 4 facility is already adequate to contain any form of alien life and that we don't even need a large-scale facility but only a lab that uses some elements of such a facility because the samples will quickly be shown to be safe to release unsterilized.
- we do have plenty of time. Though NASA have never built such a facility, the NIH often has and know how to do it and it only takes a few years even to build a complete large-scale biosafety level 4 facility
For NASA's sketch of how they will do it see: NASA's 2022 sketch for a Sample Receiving Facility: assumes samples are rapidly shown safe to remove unsterilized and leaves considerations of biocontainment for tier 2, outside expertise of MSPG2 members - as for all its studies prepared in 2022
For issues with their projection that samples will be proven safe to release unsterilized within months after a preliminary biosafety screening in the lab:
See below: Why the COSPAR Sample Safety Assessment Framework can't be used to prove safety of unsterilized Perseverance samples - If ALL the published peer reviewed Mars Sample Receiving Facility studies are correct: that we need a novel facility that would challenge the NIH to build, and that we need broad public and general scientific engagement at the earliest stage for success of this research effort
- it is already too late for the facility to be ready by 2033, planning should have started a decade ago and the facility can't realistically be ready before 2035 if this process starts today, with delays likely
See: Timetable tight to complete a sample return to a ground based lab before the 2040s; while ESA and NASA can achieve considerable cost savings for their sample retrieval spacecraft launched this decade with an early decision to return them to a life detection lab above GEO
However we need to add to this timetable as NASA should have done a new Mars Sample Return planetary protection study before starting work on its biosafety plans, the one from 2009 is over 14 years out of date now. It should also have done a new size limit and level of reassurance update of the European Science Foundation study from 2012.
The ESF study also set a requirement to contain 100% of ultramicrobacteria and a review of the literature hasn't turned up any designs for air filters that can fulfill this requirement. It is not a normal requirement for a lab and as far as I can tell from the published literature, it has only been demonstrated in fragile experimental filters in water under high pressure using silver doped carbon nanotubes.
See below: Why NASA can't rely on HEPA filters for the ESF recommendation to contain 100% of ultramicrobacteria
- Allowing a year for updating the requirements and likely several years to develop novel technology if it is required with the updated level of assurance and size limit:
- the facility is likely not going to be ready until the late 2030s or the 2040s
Finally
- If Carl Sagan was right that there is no way to protect Earth's biosphere from a lab leak of alien microbes with unknown capabilities after it contaminates human technicians (an issue that was never solved for the Apollo Lunar Receiving Facility):
- there may be no way with existing or near future technology to return unsterilized samples to Earth
See below: Even the most well prepared labs can have lab leaks - Sagan's insoluble "vexing question" of the quarantine period for a novel alien pathogen
For a couple of illustrative scenarios of potentially harmful alien life that would be impossible to keep out of Earth's biosphere after a lab leak, to help focus attention on this:
See below: Illustrative scenarios for low risk but large-scale harm: a novel fungus similar to Aspergillus fumigatus and life evolved from mirror organics - This issue was also raised by William Agger an infectious disease microbiologist who commented on NASA's plans under NEPA, Once experts in infectious diseases, invasive species and epidemiology get involved in the debate, this seems likely to be the main issue they raise repeatedly with NASA's plans.
See: Example of another substantial issue in urgent need of public dialogue: limitations of relying on studies for previous biosafety labs for human diseases and animal diseases for samples from Mars
If we can't contain alien life in a human run laboratory on Earth
- this might be solvable with a fully automated telerobotic facility of a novel design never built before but would be very expensive especially as it would need end of lifetime sterilization in the case where it turns out to have been contaminated with sterilization resistant soil pathogens or mirror life with potential dormancy periods of millennia or more.
- adds considerably to the timeline
See: Sketch for a future ground facility that is future proof to achieve no appreciable risk even for mirror life -
otherwise we need to return samples to a life detection lab in orbit around Earth, or elsewhere such as on the moon or we need to send equipment to study unsterilized samples on Mars
- the technology to do this already exists based on NASA studies for a life detection lab as far away as Europa
- since this changes NASA and ESA requirements for spacecraft to send to Mars in the late 2020s, this is something we need to know at the earliest stage, preferably already in 2024
See: Timetable tight to complete a sample return to a ground based lab before the 2040s; while ESA and NASA can achieve considerable cost savings for their sample retrieval spacecraft launched this decade with an early decision to return them to a life detection lab above GEO
If we need bonus samples for a meaningful start on astrobiological study of Mars, one of the main objectives of this mission, this also needs to be decided in the very near future since it affects design of the spacecraft to send to Mars. See:
NASA has stepped back from its former world leadership in planetary protection
The NASA Viking landers in the 1970s are still the "gold standard" in planetary protection, an unprecedented level of protection and impressive for the technology we had at the time. NASA funded much of the planetary protection research from 1997 to 2017 when it closed down its planetary protection office.
NASA's first and second planetary protection officers, though no longer working for NASA continue to do planetary protection research to this day.
However NSA itself have stepped back from their former world leading position in the field of planetary protection. See:
This book suggests that with a change of direction which has less overall cost and greater science return than NASA's current plans. The suggested change in direction also helps to enable NASA's end goal for space exploration, to support human exploration and settlement throughout the solar system. In this way the paper maps out a way for NASA can return to its world leading role on planetary protection within a program of vigorous human exploration of our solar system.
Smoke detector analogy for the low risk of large-scale harm to human health and Earth's biosphere
Dr Margaret Race's analogy of a smoke detector summarizes the consensus of the sample return studies perfectly. She was a biologist working on planetary protection and Mars sample return for the SETI Institute (now retired) and specialist in environmental impact analysis. She is also the author for a paper for COSPAR on the legal implications for a Mars sample return.
She used the analogy of smoke detectors and fire-extinguishers in response to non-peer-reviewed op. ed. by the space colonization enthusiast and leader of the Mars Society Robert Zubrin (Zubrin, 2000. Contamination From Mars: No Threat). She is responding to his suggestion that we drop all planetary protection for samples returned from Mars:
If he were an architect, would he suggest designing buildings without smoke detectors or fire extinguishers?
(Rummel et al., 2000, Opinion: No Threat? No Way : 5)
We take great care to keep our houses safe from house fires. The risk of a house fire is low but very significant. In the same way all the major studies are in agreement: we do need to pay careful attention to the methods we use to protect Earth's biosphere and inhabitants from a likely low risk of large-scale harm to human health and the environment.
Hand installing smoke detector labeled “NASA” and wooden ceiling of a house labeled“Earth”
(EnergySmart Academy, 2014, Smoke detector graphic)
Using Margaret Race’s analogy, what these studies say is that space agencies need to install a smoke detector but one that is exceptionally important despite the low risk, because Earth’s biosphere is a “house” with billions of people in it and numerous other lifeforms in it.
We shouldn't forget the other creatures potentially affected by a Mars sample return in the worst case scenarios, for instance using recent estimates:
- 50 billion birds, see (Callaghan, 2021, Global abundance estimates for 9,700 bird species)
- 3 trillion trees, see (Crowther et al., 2015, Mapping tree density at a global scale)
- There may be quadrillions of bristlemouths, a tiny but very abundant fish in the sea. (Woodshole Oceanography institute, 2019, Bristlemouth dominance: How do we know?)
- Vast numbers of insects, it's hard to find reliable estimates of the numbers of individuals.
This is how the infectious disease specialist and clinical microbiologist Dr William Agger (USNews, n.d., Dr. William A. Agger MD)put it in his comment on the draft PEIS.
William Agger: Yes, we are still here, but the Earth’s geologic periods are replete with massive extinctions which changed the course of biologic history. Is it not possible that some, if not all, of the massive extinction events may have been related to panspermic meteoric showers?
Even if only one was the result of a Martian microbe finally surviving through interplanetary space to cause a massive change in the web of life on Earth, that alone should disqualify NASA from adding this risk to the ongoing human-caused mass extinction.
... In short, it’s a scientific gamble with a small upside against a catastrophic downside for the living people on the Earth and to the only biosphere they will survive on. Finally, I am not opposed to looking for life on Mars. If found, it should be left on Mars and studied there.
(Agger, 2022, Comment Submitted by William Agger)
This is a point that the National Academy of Sciences / Space Studies Board also made in its reports on several occasions. They don't given an example of any particular mass extinction but the Great Oxygenation Event would seem to be one candidate that could have been caused by life from Mars if photosynthesis happened to develop there first then get to Earth, see below:
We need a "smoke detector" for them too.
All major planetary protection studies warn we have to contain martian life to protect against a likely low risk for large scale harmful effects on human health, or on Earth's entire biosphere
The European Science Foundation in 2012 said the samples should be contained as if they were risk group 4 (high risk of individual and community spread) until they are better understood
While, based on assumptions, some aspects of the release of unsterilised Mars material can be framed in some way, with such a level of uncertainty, unknown (and therefore unexpected) consequences driven by unknown mechanisms are conceivable and by definition are hardly manageable and predictable.
…
Following the same principle [as is used for a novel unknown pathogen], a priori assignment of a Mars sample to Risk Group 4 appears to be the best measure.
(Ammann et al., 2012, Mars Sample Return backward contamination–Strategic advice and requirements : 24)
NASA's second planetary protection officer Cassie Conley who is a world expert on planetary protection for a Mars sample return mission with many papers on the topic, similarly said
that means we are going to contain the samples as if they were the most hazardous Earth organisms that we know about, Ebola virus.”
at (NASA, 2013, Video: Cassie Conley on Safe Guards Against Contamination : 1:02)
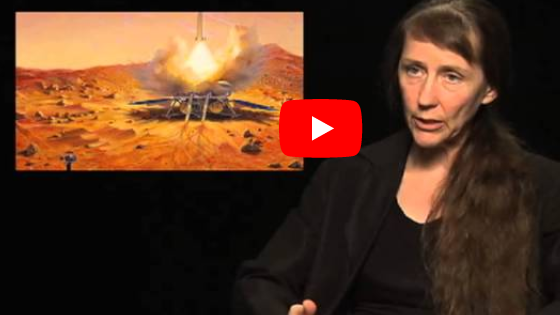
Video: Cassie Conley on Safe Guards Against Contamination
On environmental effects, the ESF report concurred with earlier reports that the risk appears to be low but is not demonstrably zero.
The Study Group also concurs with another conclusion from the NRC reports (1997, 2009) that the potential for large-scale effects on the Earth’s biosphere by a returned Mars life form appears to be low, but is not demonstrably zero.
(Ammann et al., 2012, Mars Sample Return backward contamination–Strategic advice and requirements 20)
There is no mention in the PEIS of the possibility of a risk of large scale harm to human health or large scale changes to Earth's biosphere. This fails the US legal NEPA requirement for agencies to discuss all major points of view
At appropriate points in the draft statement, the agency shall discuss all major points of view on the environmental impacts of the alternatives including the proposed action.
§ 1502.9
See: Fails NEPA requirement to discuss major points of view by not mentioning the view of a low risk of large-scale harm in the Supplementary information
For all the NEPA issues see : ⸽ How NASA's current PEIS fails many central NEPA requirements again in the Supplementary information
This book will draw attention to many failings of fundamental legal NEPA requirements. It does NOT recommend that anyone takes out legal action against NASA on these issues. Rather the reason for pointing out when the PEIS fails NEPA requirements is that they are there for a reason, to protect Earth's biosphere in this case.
The book recommends that NASA withdraws this PEIS to fix all the NEPA issues it identifies and to find another way ahead. It recommends that any new plans are based on peer reviewed science rather than novel findings and conclusions only found in the PEIS. It provides one such solution in detail, and so is constructive criticism of NASA's current plans. The hope is that this book will stimulate interest in looking more closely at these plans and lead to a request for peer review from the Space Studies Board or the European Science Foundation or both.
Illustrative scenarios for low risk but large-scale harm: a novel fungus similar to Aspergillus fumigatus and life evolved from mirror organics
We know what a house fire looks like, so we take safety measures to prevent this low risk. However, the studies lack examples to show why we need to prevent large-scale harm from martian life.
I found only one worked public health example in the 2009 study, an extremophile in hydrothermal vents that evolved virulence genes used by its close cousins to harm humans (SSB, 2009, Assessment of planetary protection requirements for Mars sample return missions : 48)..I found no examples for environmental effects, for instance, the ESF just says it is not possible to definitively assess how a Mars organism would interact with the Earth's biosphere. (Ammann et al., 2012, Mars Sample Return backward contamination–Strategic advice and requirements : 24)
This makes it rather like builders of a house debating about how to protect it from fires when they have no examples of any house that has ever gone on fire. All we have are unrealistic sci-fi scenarios
- the crystalline lifeform of the Andromeda strain (Bookrags, 2023, Andromeda Strain plot summary)
- "Life", a 2017 movie about Mars samples in which a microbe implausibly grows into a multi-cellular intelligent being (Boyle, 2017, ‘Life’ horror movie sparks reality check on procedures for studying Mars samples)..
Neither of these is even remotely plausible.
I worked on several scientifically based illustrative scenarios to help inform public debate and decision making.More concrete scenarios can help focus attention on the need to take precautions and on particular issues one might not think of so easily reasoning in the abstract.
I have selected two of those for the main focused in this book. One of them is an example to illustrate large-scale harm to human health and the other is a similar scenario for the environment.
For human health we can use a Mars analogue of Aspergillus fumigatus. This fungus is harmless to most of the world population, but it causes millions of cases of asthma a year, hundreds of thousands of cases of a serious progressive lung condition (Denning et al., 2013, Global burden of allergic bronchopulmonary aspergillosis with asthma and its complication chronic pulmonary aspergillosis in adults), and tens of thousands of deaths in immunocompromised people every year (Brown et al., 2015, Hidden killers: human fungal infections : table 1).
What makes this fungus a particularly good analogue for a pathogen from Mars is that
- it is not adapted to a infectious lifestyle in any organism (McCormick et al., 2010, Aspergillus fumigatus: contours of an opportunistic human pathogen).
- it is able to grow in human lungs due to adaptations to harsh environments (Paulussen et al., 2017, Ecology of aspergillosis: insights into the pathogenic potency of Aspergillus fumigatus and some other Aspergillus species : table 1, table 2 and section: Biophysical capabilities and ecophysiology of pathogenic Aspergillus species).
- When we look at those adaptations in detail, we find a martian fungus would be expected to evolve similar adaptations to the stressful environments on Mars (e.g. chemical barriers, corrosive chemicals to degrade organics, fast hydration, fast revival when warmed from cold conditions, resilient to both low oxygen and oxidative stress, rapid uptake of nutrients when available using rapidly growing hyphae etc. The closely related a. niger is also a facultative anaerobe and the martian surface has higher levels of oxidative stress from the perchlorates than most terrestrial microenvironments.
- The worst case here is that if it is a species from a novel fungal genus, our immune system would likely have no response to protect us as we don't have a broad spectrum response to fungi (their cell walls look too like our own cells), or our immune system might overreact with an allergic reaction. Either could be quite serious for us.
We could find native fungi on Mars as black fungi do well in Mars simulation chambers. One of these is an occasional pathogen of humans,.Exophiala jeanselmei MA 2853 (Zakharova et al., 2014. Protein patterns of black fungi under simulated Mars-like conditions). Its close relatives are sometimes fatal.(Wu et al., 2015, Cutaneous Phaeohyphomycosis of the Right Hand Caused by Exophiala jeanselmei:, 2022 : table 2).
I cover this scenario in detail here:
For large-scale harm to the environment we can use life evolved from mirror organics. Everything flipped as in a mirror, DNA spirals the other way and so on. It should work just like normal life but flipped.My analogue is a mirror life analogue of the blue-green algae Chroococcidiopsis, which does well in Mars simulation chambers and could live almost anywhere on Mars with access to habitable water or possibly just the humidity of the air condensing in micropores in salt deposits, and which could radiate and spread to almost any ecosystem or microecosystem. on Earth if it established a foothold here.
Mirror life is one form of exobiology everyone agrees is possible, and indeed synthetic biologists plan to try to create it in the laboratory by flipping the components of a cell of terrestrial life step by step, though it may take a decade or more. Everyone agrees the end result should be a functioning organism, though there are technical difficulties involved in the process of flipping a cell component by component like that. They have looked at the scenario of mirror life spreading through our biosphere, to motivate taking great care with any synthetic experiments involving mirror life (Bohannon, 2010, Mirror-image cells could transform science-or kill us all).
Synthetic biologists design in multiple different dependencies into their engineered life to prevent them replicating in the wild. In this way they achieve a level of containment far higher than for a conventional biosafety laboratory. It is essentially impossible for their synthetic life to escape, with a safety goal similar to what in this book we are calling informally "100% safe" (Torres et al., 2016, Synthetic biology approaches to biological containment: preemptively tackling potential risks)
We will see mirror life is also one possibility for Martian life. However this time we can't engineer the life to be safe for Earth's biosphere. If Mars has mirror life it evolved in a second genesis just as our life did, but it evolved from the mirror forms of the organics terrestrial life evolved from.
Experts agree it's not impossible that we return independently evolved life from a second genesis on Mars. NASA's iMOST team put it like this in their discussion of what forms of life we could return in the Perseverance samples:
“We cannot predict with any accuracy life's form and characteristics, whether it would be viable …, or whether it shares a common ancestor with life on Earth.”
(Beaty et al., 2017. iMOST : 88)
To return mirror life in the Perseverance samples, many things need to fall into place. There may be only a very low likelihood for Mars to have independently evolved life. Then we need it to be evolved from mirror rather than ordinary organics (some say that's a 50- 50 chance). It also needs to survive to the present, to be returned in the samples, and to be able to live on Earth.
We are not at present able to assess probabilities for any of these prerequisites for mirror life in the samples. It may well be a very low likelihood possibility, but it is scientifically credible, it is not science fantasy, and we can’t at present say it is impossible.
I introduce the two scenarios here briefly then cover the mirror life scenario first in more detail followed by the fungus scenario already mentioned:
As well as these major effects of large-scale harm, we shouldn't ignore the potential for "minor" effects that still may impact on our economy and incur costs of billions or even trillions of dollars over time. There are many such examples one can think of, but as a representative example I added a third scenario, already in the literature, of life that can survive in freezers, leading to major economic issues as freezers would have to be designed to operate at lower temperatures to prevent contamination of food by martian microbes. The economic costs could easily be greater than the space budget.
See below:
Lab leak responses can benefit from concrete scenarios like novel fungi or mirror life, which may help focus attention once more on Sagan's "Vexing question" of the latency period
Concrete scenarios like this can encourage decision makers to focus on important issues in more detail. For instance, a lab operated by humans needs precautions in place for a lab leak. The Lunar Receiving Laboratory for Apollo used quarantine to help protect Earth's biosphere but it was an imperfect compromise solution. We can use these two scenarios to look at issues with that approach.
Human quarantine is not likely to stop mirror life or other life that may be harmless in the human microbiome and cause problems only as it spreads through ecosystems. It also couldn't stop a fungus resembling Aspergillus fumigatus, a common fungus even in the ISS (Knox et al., 2016, Characterization of Aspergillus fumigatus Isolates from Air and Surfaces of the International Space Station). The ISS gets new astronaut visitors only every few months and has a two week health stabilization program, essentially quarantine, before any of the astronauts leave Earth (NASA, n.d., Health Stabilization Program). But that can't keep out a fungus that can live in the human microbiome and is harmless to young healthy astronauts. Similarly it would be impossible to guarantee to keep a martian analogue of Aspergillus contained after a lab leak that contaminates human technicians with any quarantine period however long.
I have found no discussion in the recent Mars sample return literature of this "Vexing question" in Carl Sagan's words. He mentioned Leprosy which has a latency period of two decades as we now know (WHO, 2019, Leprosy, Key facts). It is even more acute for diseases with lifelong symptomless spreaders like typhoid, or as happens for immunocompromised patients with COVID (Abbasi, J., 2021. Researchers tie severe immunosuppression to chronic COVID-19 and virus variants). It is the same situation for fungi which are harmless for most people but deadly for some. This issue was discussed a fair bit in the Apollo era and in modern historical discussions of quarantine issues for Apollo.
The only recent paper I've found that discusses responses to a lab leak with modern technology is Pugel et al. They however have no resolution for the issue of a quarantine period. They expect Federal agencies to issue hospitals with updated guidance during an outbreak of a pathogen and expect the guidelines to change. I cover this paper with quotes from it below in:
They mention several issues that need to be looked at such as a likely delay in developing diagnostic tests for a novel pathogen of alien biology and potential issues in adapting sterilization practices in hospitals to novel biology (Pugel et al., 2020. Restricted and Uncontained: Health Considerations in the Event of Loss of Containment During the Restricted Earth Return of Extraterrestrial Samples : 9).
They don't discuss fungi, microbes harmful to wildlife or ecosystems or the likes of mirror life. This issue will need to be looked at closely to devise safety measures for unsterilized samples from Mars, if they are ever returned to a Mars Receiving Laboratory on Earth (or indeed to one staffed by humans in space). Judging by the extensive interagency discussions of this topic for Apollo, this quarantine issue is one of the things experts in biosafety would want clarified before signing off on a biosafety plan.
Another issue which scientists wrestled with at the time of the Apollo missions is, what do we do if a technician in quarantine is seriously ill? We can't leave them in quarantine to die, but that is the very situation where quarantine is most needed if it is a martian pathogen that afflicts them. We look at these issues below in:
NASA never found a resolution of this vexing question. Rather, they simply settled on a compromise that seemed good to them. According to Richard Bryan Erb, manager of the Lunar Receiving laboratory from 1969 to 1970 (Carroll, 2019, The Early History of Canadian Planetary Exploration). the Apollo quarantine procedures were just intended to try to stop a pathogen with a short incubation period of less than 3 weeks, to ensure any novel pathogen spreads slowly enough so that we can prepare countermeasures. Even then, they had no real ethically sound solution for the situation of a fast acting pathogen that requires technicians to be treated in hospital (Butler, 1999, Edited Oral History Transcript). In the interview he says:
Erb: It'll be interesting to go through this again as we tackle Mars samples return, - but now with, I think, a much greater likelihood of life forms from Mars."
(Butler, 1999, Edited Oral History Transcript).
See:
We do have options to do good science with no risk to Earth or effectively 100% protection (no realistic risk)
Unlike a house fire this is a risk we can prevent altogether. I mention this because in the PEIS NASA replied to proposals to use extra precautions to keep Earth safe with extra precautions (including mine) by answering:
No outcome in science and engineering processes can be predicted with 100% certainty.
(NASA, 2023, Mars Sample Return FINAL PEIS : B-55)
My proposals which triggered that reply involved sterilizing any samples BEFORE they contact Earth's biosphere. So to avoid confusion, I'd like to clarify what is meant by 100% safe in this book.
First, if we never return samples from Mars they can never harm Earth's biosphere. With the incredible miniaturization of technology for biological sciences, it is now feasible to search Mars for life remotely on Mars itself using modern miniaturized life detection instruments, with a thoroughness well beyond the capabilities of previous decades.
Similarly we can return unsterilized samples to a satellite in a safe orbit within easy reach of Earth and study them there. If we never return any sample to Earth and only send materials in one direction from Earth to the satellite, there can be no risk to Earth.
Both these options have zero risk for Earth.
We can also use sterilization to make the risk as low as we like, to the point it is not realistic as a possibility.
100 million years of surface ionizing radiation would reduce any DNA to strands around 30 bases long. If 100 million years of ionizing radiation isn't considered to be enough, a billion years of ionizing radiation which still would have little effect on the geology for samples that have been exposed for 3 billion years, and would reduce any molecule similar to DNA to fragments only a few bases long.
For details see:
This is the approach for the option to return a sample to above GEO which I proposed in my final comment on the NASA PEIS and also cover in this book to show that it is possible to do good science without taking even a tiny risk with a billion lives.
- Life detection with miniature instruments in a teleoperated satellite above GEO: an alternative that plays to NASA's strengths, modelled on its proposed in situ Europa life detection lander
- This proposed alternative submitted to NASA under NEPA uses a centrifuge for Mars gravity in an excellent Mars simulation chamber, which also means instruments can operate under Mars gravity
- Modern miniature life detection instruments that could fly to above GEO, or to Mars
In this book I use "100% safe" to refer to no realistic risk, a level of risk that though mathematically calculable is ordinarily considered impossible.
Depending how we do the sterilization, suppose we use a cobalt source. It is mathematically possible that by chance none of the cobalt atoms in gamma ray source decay, and so they don't emit any gamma rays for the duration of the sterilization process, for several hours. This is not however a realistic possibility when the source is emitting thousands of gamma rays per second through the natural unstoppable process of radioactive decay.
Apart from that it is a matter of the sterilizing dose needed, and whether extraterrestrial life could be of some unusual structure, for instance perhaps sheet-like, like prions, rather than 1D and so more resilient to ionizing radiation.
This is a matter that would be worked on internationally to decide on a level of sterilization that everyone agrees is adequate to sterilize any conceivable biology we might return from Mars. The experts tell us we can sterilize any life based on covalent bonds by using doses high enough to sever the bonds that hold the molecules together.
See:
I will use "100% safe" or "No realistic risk" in this book as described here. It refers to a risk that can be made as low as we like it to be by selecting a level of sterilization adequate, and which can be made so low that it wouldn't normally be considered a realistic risk at all.
It isn't possible to calculate probabilities in the classical sense for an unknown biology. But if, for instance if any DNA is broken up into fragments of 30 bases maximum, it is next to impossible that even unfamiliar life could survive that and still be viable, unless its informational biopolymer is somewhat two dimensional like prions. If that is considered possible, a higher dose may be needed. We will find that even ten times that dose, reducing it to fragments only a few bases long, would still be equivalent to just one billion years of surface ionizing radiation, and most geological investigations would be unaffected.
Broad acceptance by scientists and general public is essential for this research effort to succeed
This is how John Rummel, NASA’s first planetary protection officer, put it in 2002:
“Broad acceptance at both lay public and scientific levels is essential to the overall success of this research effort.”
(Rummel et al., 2002, A draft test protocol for detecting possible biohazards in martian samples returned to Earth : 99)
Mars sample return studies emphasize the need to involve the public early on, not just in the USA, but globally, for instance, using fora open to representatives from all countries. They say this is necessary because negative impacts could affect countries beyond the ones involved directly in the mission.
This is from the most recent ESF study in 2012 which also says civil society generally, and the scientific community should be involved in risk governance as soon as possible.
RECOMMENDATION 3
Potential risks from an MSR are characterised by their complexity, uncertainty and ambiguity, …, civil society, the key stakeholders, the scientific community and relevant agencies’ staff should be involved in the process of risk governance as soon as possible.
, transparent communication covering the accountability, the benefits, the risks and the uncertainties related to an MSR is crucial … Tools to effectively interact with individual groups should be developed (e.g. a risk map).
RECOMMENDATION 4
Potential negative consequences resulting from an unintended release could be borne by a larger set of countries than those involved in the programme… mechanisms and fora dedicated to ethical and social issues of the risks and benefits raised by an MSR are set up at the international level and are open to representatives of all countries
(Ammann et al., 2012, Mars Sample Return backward contamination–Strategic advice and requirements : 59)
This was written in 2012, over a decade ago, yet this wasn't done. either in the USA or ESA.
The interagency panel had at least four members knowledgeable in one or more of the fields of bioethics, law, public attitudes and the communication of science, the Earth’s environment, or related fields, by its charter (SpaceRef editor, 2001, Planetary Protection Advisory Committee Charter)
However NASA lost that expertise when they closed down the panel in 2007 See below:
There will be intense public scrutiny of NASA’s plans as it gets nearer to the date the samples return to Earth. This is why, if NASA still doesn’t have a functioning smoke detector, in this analogy NASA will have to fix this before 2033.
The Mars sample return studies all say part of this process is to set up mechanisms and involve the public as soon as possible.
It is also a NEPA requirement when selecting the preparers for the PEIS to use an interdisciplinary approach that will ensure integrated use of the social sciences.
Agencies shall prepare environmental impact statements using an interdisciplinary approach that will ensure the integrated use of the natural and social sciences and the environmental design arts (section 102(2)(A) of NEPA).
§ 1507.2
This would seem to indicate a need for NASA to comply with the recommendations from all the major Mars sample return studies to involve the public early on.
From Mars Sample Return studies it is clear the selection of the disciplines of the preparers should reflect the wider social and ethical issues involved in this mission. The selection of disciplines should also reflect the need for effective communication with the public and to involve the public in matters of risk governance early on in the process. This is covered in more detail below:
NASA's four novel findings in the PEIS and two novel conclusions of negligible health risk and that environmental effects would not be significant
NASA restricted the scope of the potentially affected environment from the outset to the Utah Test and Training Range which is where the container containing the samples lands in the USA in NASA's plans. NASA also did this PEIS in two tiers. Any consideration of sample handling in the Mars Receiving Facility and how to respond to lab leaks is left to tier 2 which is intended for effects that are not ripe for discussion at tier 1.
This is based on NASA's two new conclusions central to the PEIS, that:
- any effects on the environment would not be significant (NASA, 2023. Mars Sample Return final PEIS : 3-16), and localized to the Utah Test and Training Range,
- any effects on human health would be negligible. (NASA, 2023. Mars Sample Return final PEIS : 3-14)
When asked for a source for the first of these conclusions, that any environmental effects would not be significant, NASA responded that the source is the PEIS itself. See below:
This reasoning is not actually valid. To see why, the risk of a house fire is very low but that does not support a judgement that house fires are not significant. I cover this in my book under:
NASA separately confirmed the PEIS is not peer reviewed, with no requirement under NEPA for it to be peer reviewed. See:
In my literature search I have found no peer reviewed paper on a Mars sample return that derives either of NASA's two main conclusions
NASA relies on four new findings to derive these two conclusions:
- any harmful life can get here better protected in meteorites (NASA, 2023. Mars Sample Return final PEIS : 3–3),
- Mars has been uninhabitable for millions of years (N 3. Mars Sample Return final PEIS : 1–6)
- martian microbes would have near zero chance to harm humans because of the lack of similar hosts on Mars (Craven et al., 2021. Biological safety : 6)
- any martian life would be unable to survive on Earth (Craven et al., 2021. Biological safety : 6)
NASA provides no previous cites for these findings either. No mentions of anyone who has used them before in the planetary protection literature.
My literature survey only found similar findings in a non peer reviewed op ed (Zubrin, 2000. Contamination From Mars: No Threat) rebutted in the next edition of the Planetary Report with NASA's first planetary protection officer as lead author (Rummel et al., 2000. Opinion: No Threat? No Way : 4 - 7).
These are covered in detail in this book under:
These are the four rebuttals:
- How NASA's central finding that any harmful life can get here better protected in meteorites is rebutted by BOTH of its main cites for it
- NASA's uninhabitable Mars finding rebutted by its own cite, and by NASA's own plans to test for life in its samples
- NASA's Biological Safety Report's finding of near zero risk that martian life can harm us rebutted by soil pathogens like tetanus and aspergillus fumigatus not adapted to any terrestrial host
- NASA's Biological Safety Report's finding that any martian microbe would not be viable on Earth rebutted by the only plausible Mars analogue in its cite: Planococcus Halocryophilus
The most important of these four arguments for NASA's conclusions in this PEIS is the first one, that any harmful life can get here better protected in meteorites,
NASA:
“The natural delivery of Mars materials [i.e. martian meteorites on Earth] can provide better protection and faster transit than the current MSR [Mars Sample Return] mission concept
…
First, potential Mars microbes would be expected to survive ejection forces and pressure (National Academies of Sciences, Engineering, and Medicine and the European Science Foundation 2019), …”
(NASA, 2023. Mars Sample Return final PEIS : 3–3)
This argument is rebutted on page 5 of NASA's main National Academy of Sciences cite for the quoted sentence:
- Martian material present in a Phobos/Deimos sample would have undergone several physical sterilization processes (e.g., excavation by impact, collision with Phobos, and exposure to radiation), before it is actually sampled. Material collected on the surface of Mars will not have undergone such processes.
- MSR material might come from sites that mechanically cannot survive ejection from Mars and thus any putative life-forms would de facto not be able to survive impact ejection and transport to space. Such mechanical limitations do NOT apply for material collected on
MarsPhobos/Deimos.Therefore, the committee finds that the content of this report and, specifically, the recommendations presented in it do NOT apply to future sample return missions from Mars itself.
(SSB, 2019, Planetary protection classification of sample return missions from the martian moons : 5)
[Corrects obvious typo, Mars to Phobos - it is covered in more depth in their cite without the typo on page 45. In this passage, examples of sites that mechanically cannot survive ejection from Mars would include ice, dust, dirt and salts on the surface of Mars]
It is rebutted in more detail on page 45 of this cite (SSB, 2019, Planetary protection classification of sample return missions from the martian moons : 45). However, the reader of the PEIS is never alerted to this discrepancy or any other view on the matter; only NASA's own views. In their responses to the public comments and in the PEIS itself, NASA's team seem unaware of this discrepancy. This suggests it may need external peer review.
I didn't spot the rebuttal in the 2019 cite in time for the NEPA comments (but NASA should have read their own cite of course). However I did alert NASA to the similar rebuttal of the Mars meteorite argument by the National Academy of Sciences / Space Studies Board in 2009, NASA even cited my comment where I alert hem to this rebuttal.
NASA doesn't seem to have noticed that my comment alerted it to a rebuttal of the sentence in the PEIS in their own cites, and didn't correct the text in the final PEIS to alert the reader to this discrepancy between the text and the cites attached to it.
Robert Walker: NASA fail to adequately consider the risks from life that can’t get to Earth on meteorites 2009, the National Research Council examined the possibility of life transferred on meteorites said the risk is significantly greater in a sample return mission - and said they can’t rule out the possibility of large scale effects in the past due to life from Mars – NASA’s EIS instead claims microbes will survive transfer from Mars to Earth more easily in a meteorite than in a sample return mission but their sources don’t back this up
[NASA don't seem to notice the highlighted text and instead only respond to the start of the sentence]
NASA: Additionally, the PEIS details and references on pages 3-3 to 3-4 information on the unlikely risks from “life that can’t get to Earth on meteorites.”
[those pages 3-3 to 3-4 are the pages that outline the Mars meteorite argument that my comment tried to draw their attention to as having a major issue with it]
(NASA, 2023. Mars Sample Return final PEIS : B-59 - B-60)
In the text highlighted in red, I am referring to this quote from the National Research Council study:
Section “Martian meteorites, Large-Scale effects and Planetary Protection” The potential hazards posed for Earth by viable organisms surviving in samples [are] significantly greater with a Mars sample return than if the same organisms were brought to Earth via impact-mediated ejection from Mars
…
… Thus it is not appropriate to argue that the existence of martian meteorites on Earth negate the need to treat as potentially hazardous any samples returned from Mars by robotic spacecraft.
(SSB, 2009, Assessment of planetary protection requirements for Mars sample return missions : 47 - 48)
If NASA's result was true, it would be a major new finding in the literature on a Mars sample return and overturn the NRC findings. However, NASA's reasoning in this PEIS would never pass peer review.
More details here:
We see that NASA's PEIS is the outlier here.
NASA's Mars meteorite argument IS valid for samples returned from Phobos because any martian life there has ALREADY been ejected from Mars to get there, and so has to be from materials that can be ejected and withstand ejection, and it also has to survive the impact sterilization of hitting the surface of Phobos at kilometers per second. We cover this later in this book::
What is novel in this PEIS is that NASA apply this same Mars meteorite argument to samples returned from the surface of Mars.
NASA's four arguments build up to NASA's two conclusions as already mentioned. First, a conclusion that any environmental effects would not be significant.
The relatively low probability of an inadvertent reentry combined with the assessment that samples are unlikely to pose a risk of significant ecological impact or other significant harmful effects support the judgement that the potential environmental impacts would not be significant.
(NASA, 2023. Mars Sample Return final PEIS : 3-16)
This reasoning is not actually valid. To see why, the risk of a house fire is very low but that does not support a judgement that house fires are not significant. See below:
Secondly, a conclusion that any public health risks from lab leaks from the Mars Receiving Facility are negligible
… the risk to the public [from lab leaks] is negligible
(NASA, 2023. Mars Sample Return final PEIS : 3-14)
See below:
As someone who had been working on a paper on planetary protection for NASA's mission for some years, I was very familiar with NASA's previous plans. The use of this argument and the other three arguments was by far the most striking difference between the plans in the PEIS and NASA's previous plans, or the recommendations in the Mars sample return studies.
As we saw above, major peer reviewed studies by the NRC and the ESF present a consensus view of a low risk of large-scale harm to human health and the environment (Ammann et al., 2012, Mars Sample Return backward contamination–Strategic advice and requirements 20, 24, 58) (SSB, 2009, Assessment of planetary protection requirements for Mars sample return missions : 48). NASA/'s second planetary protection officer Cassie Conley says the samples will be contained as if they were the most hazardous Earth organisms known in an official NASA YouTube video. See above:
NASA's conclusions from this argument and others and its confidence in the validity of its conclusions had major effects on the PEIS. First as mentioned, they restricted the "affected location " to the Utah Test and Training Range.
These conclusions also led to NASA adopting the two tier approach mentioned at the start of this section. With their conclusion that any public health risks are negligible they postpone all discussion of risks of lab leaks from the Mars Receiving Facility to tier 2, which would mean a lesser and localized level of environmental risk. It also lead to them restricting the scope of any potential environmental effects in tier 1, the one the PEIS covers, to the Utah Test and Training Range.
See below:
This also lead to NASA's decision that its scoping meetings and request for public comments in the first round of public comments and the review of the Draft PEIS in the second round of public comments are matters only of relevance to people local to the Utah Test and Training Range. As a consequence of this conclusion, they only advertised in local newspapers not nation-wide or globally.
Because of this, NASA's scoping process and its draft PEIS didn't have extensive public scrutiny. They were only looked at by locals and by anyone who heard about it through the grapevine,
in my case I heard about the draft PEIS and the first round scoping process because a number of people knew of my interest in the topic and that I was working on a paper on NASA's very mission. I heard about it by chance, from an online friend. Public comments were open globally, in my case commenting from the UK. But the scoping process and the PEIS were only publicised in the vicinity of the Utah Test and Training Range and the Kennedy Space Center.
This low key approach with limited publicity simplifies things for NASA in these early stages. But this lack of attention by the public, including scientists, at a national or global level can lead to issues later on. It means NASA's plans have had much less scrutiny and much less feedback for them to learn from than if they had publicized it nationally or globally.
The main concerns raised in the public comments on NASA's PEIS were similar to Sagan's concerns, a risk of large-scale or even unprecedented impacts on public health or the biosphere. This is one example of many of the comments:
Richard Spotts: I am extremely concerned that this proposed action could potentially contaminate native life forms on Mars and/or bring back alien virus, bacteria, or other life forms from Mars to Earth.
I understand that there are planetary protection protocols. However, Murphy's Law says that if something horrible could happen, it eventually may indeed occur. History is filled with examples where Acts of God and/or human arrogance caused otherwise unforeseen disasters.…
The Earth is already dealing with increasingly serious problems from invasive or alien species being transported to new locations, and viruses mutating and causing deadly pandemics. We have not been able to solve many of these problems.
What happens if a Mars life form escapes containment and, without evolving in Earth's ecosystems, spreads uncontrollably and devastates Earth's species including us humans? There might be no way to reverse or even mitigate for that devastation.
I support scientific research when it is safe and in the public interest. However, I oppose research when there is no absolute guarantee of safety and when the risks outweigh the potential benefits.
(Spotts, 2022, Comment Submitted by Richard Spotts)
Chester Everline, a co-author of NASA's handbook on probabilistic risk assurance (NASA, 2011, Probabilistic risk assessment procedures guide for NASA managers and practitioners) who commented on the last day of public comments put it like this:
Chester Everline: Given our lack of scientific insight into possible life on Mars, relics of life we may return from Mars, or simply organic substances from Mars that could interact with certain life forms on Earth, how can we possibly assert with confidence that MSR [Mars Sample Return] poses an acceptable risk to Earth's biosphere, … NASA, 2023. Mars Sample Return final PEIS : B38)
Chester Everline continues:
Does NASA intend to impose a threshold for acceptable risk (i.e., a value above which the mission is considered too risky to proceed)?
A possible consequence of unsuccessful containment is an ecological catastrophe. Although such an occurrence is unlikely, NASA should at least be clear regarding what level of risk it is willing to assume (for the biosphere of the entire planet)
NASA responded in the same way Chester Everline, to Richard Spotts, and to myself referring us all back to this response to Logan Greger.(NASA, 2023. Mars Sample Return final PEIS : B38)
This is their answer to all of us, no matter how serious the potential consequences mentioned. In this case Greger was concerned about mass extinction or that humans might have to live in biomes for the rest of time
Greger: Are you certain that in any way, this mission won’t end with the total annihilation of the entire planet, or force us to live in biomes for the rest of time?
NASA: As discussed in Section 3.2 of the PEIS, the exact nature of the Mars sample constituents regarding biosignatures and potential biological activity is currently unknown.
The PEIS cites several sources supporting the position that contamination of Earth by Martian microorganisms is extremely unlikely to pose a risk of significant harmful effects. However, the risk cannot be demonstrated to be zero(NASA, 2023. Mars Sample Return final PEIS : B-43)
NASA go on to say in reply to Greger that with current data any analysis of potential impacts would involve substantial speculation so the focus is on sample containment.(NASA, 2023. Mars Sample Return final PEIS : B-43)
NASA could more skillfully have reassured Greger that the risk is likely ultra low like a house fire and that such an event would unfold most likely over decades or centuries and maybe millennia rather than happen instantly. It wouldn't resolve the question, but it would give the general public a more accurate idea of what is meant by low risk here.
I provide direct links to all the comments submitted in the final round of public comments with a brief summary of the level of concern for each one here:
I expand on this and discuss why if NASA continue to respond in this way it is not likely to be an effective way to reassure the public of the safety of the mission below in:
I talk about the importance of engaging in extensive open dialogue with the public and scientists of other disciplines globally at an early stage, as stressed in all the sample return studies here:
And the current near impossibility of dialogue here:
Pitfalls of NASA's approach and how an alternative vision can build in resilience to future discoveries about Martian biology
NASA's PEIS is an example many other agencies and private space companies will follow, unless it is withdrawn or challenged. It has global importance as a precedent. From now on any paper on planetary protection for a Mars sample return has to discuss it.
This book draws out many pitfalls of relying on a PEIS based on novel arguments rebutted in their main cites, and of devising a biosafety plan for Earth without the expertise in the research on Mars sample return missions of their former planetary protection officers.
To protect Earth this book suggests NASA explores alternatives where samples that may contain living martian microbes are studied outside of Earth's biosphere, with the suggestion of a miniature lab above GEO.
To protect both Earth and Mars (from clashes between the biospheres in both directions if Mars has a biosphere), this book suggests NASA could make their intermediate goal humans in orbit around Mars, exploring the surface rapidly via low latency telepresence and using the resources of the martian moons. This suggested approach of an intermediate goal in Mars orbit is resilient to future discoveries.It also avoids the risk of multi-billion dollar stranded assets on Mars.
- If we discover that it is biologically safe to land humans on Mars, the orbital base and the many assets on the surface would be of great value for humans on the surface.
- If we find that Mars has a biosphere which can never mix safely with the terrestrial biosphere, the bases in orbit around Mars have potential to be the hub of a colony exploring the surface of a biologically different terrestrial planet form orbit as well as a waypoint to the asteroid belt and beyond.
A base on the Martian moons or in orbit around Mars is also far more relevant to human exploration of the rest of the solar system than NASA's current goal of a base on the martian surface with unique challenges such as the martian dust storms, perchlorates and the difficulties involved in landing large payloads on a planet with a thin atmosphere and several; times the gravity of the Moon. Mastering those challenges is only really of relevance to Mars while mastering the challenges of living on Phobos is the key that can unlock ISRU for most of the rest of the solar system.
The next two sections list 12 potential pitfalls if NASA continue with their current plans, then a roadmap for this book in 16 steps.
Twelve potential future pitfalls for NASA's current plans
There are many ways NASA's current mission plan can fail. We will see we can build in resilience to these pitfalls with alternatives such as the miniature life detection lab above GEO.
- Legal challenges under NEPA - 49 out of 63 public comments were opposed to NASA's plans in the second round and Environmental Impact Statements are often challenged. See below: Most public comments share Sagan's priority that NASA can't take even a small risk of large-scale harm
- The plan is for ESA to deliver the samples to the USA. This makes ESA a party to the project, so ESA countries need to consider obligations under the Biodiversity Convention on control of invasive species which they all ratified. They also need to consider various other international and domestic laws. They have domestic legislation similar to NEPA which would apply because of the potential low risk of global public health or ecological effects from their actions even though they are returning the samples to the USA. This also brings with it obligations under the Espoo convention which most belong to, to consult with other parties of the convention that could be impacted outside their national borders. In this case all 45 parties to the Espoo convention are potentially affected in the low risk worst case. See below: Obligations for ESA countries based on the conclusion of the ESF study from 2012 on low risk of large-scale harm
- NASA depends on ESA for the Sample Retrieval Lander and the Earth Return Orbiter. ESA has paid
little attention to planetary protection issues for this mission so far. ESA's main planetary
protection page hasn't yet been updated to mention their participation in the Mars sample return
mission. ESA removed their former planetary protection officer, replaced by a new official which has
a wide range of responsibilities including space debris, nuclear safety and so on. As public
interest grows, discussion in ESA countries may lead to ESA paying more attention to planetary
protection for this mission. See below:ESA seem to have no independent public discussion yet of planetary protection for their participation in NASA sample return mission
ESA might commission an update of their own 2012 ESF study (Ammann et al., 2012, Mars Sample Return backward contamination–Strategic advice and requirements : 24) focusing on the level of assurance and size limit as recommended by the one in 2012 and on the feasibility of satisfying those requirements with a BSL-4, and the feasibility of developing the necessary technology if not. They may then ask NASA for a change of plan before they can continue with the mission.
See below: Why NASA should have commissioned a review of the size limit and level of assurance first, as recommended by the 2012 ESF study - Presidential directive NSC-25 requires "a review of large scale effects that could be reasonably expected to result in allegations of major or protracted effects" The head of NASA should recommend such a review even if the agency feels confident such allegations are false (White House, 1977, Presidential Directive NSC-25: Scientific or Technological Experiments with Possible Large-Scale Adverse Environmental Effects and Launch of Nuclear Systems into Space). The process starts when the head of the agency calls their proposals to the attention of the Director of the Office of Science and Technology. The Director then consults with the Chairman of the Council on Environmental Quality. Together they review the proposals and the supporting materials and can order further studies if they don't feel the potential allegations have been addressed adequately. This directive would seem to apply since all the major peer reviewed studies say there is a likely low risk of large-scale harm to human health and the environment even though the PEIS argues that there is no significant risk.
See below: Potential opportunity for NASA to officially withdraw their final PEIS and seek independent peer review, or to recommend it for independent assessment under presidential directive NSC-25
and the Supplementary information section under:
By the presidential directive NSC-25 (possible large-scale adverse environmental effects), NASA should draw their PEIS to the attention of the Director of OSTP which can lead to the chair of the CEQ ordering further studies - Issues may be raised as a result of press briefings in 2033, if the mission survives all previous
challenges. This seems especially likely if NASA continues to rely on the four novel findings in the
PEIS for their biosafety plan. Many in the general
public find the argument implausible that if there is life on Mars it can get here better
protected in a meteorite than in the sample tubes,
See below: How NASA's central finding that any harmful life can get here better protected in meteorites is rebutted by BOTH of its main cites for it
The other three novel findings that NASA uses in this PEIS to support their biosafety plan also are not ones the general public are likely to find plausible.- If NASA continues to assure the public that existing credible evidence says Mars has been uninhabitable for millions of years, we can also expect their scientists in a separate press briefing to say they will test the returned samples for respiration, metabolism and to see if anything can grow from them, This could impact on the credibility of NASA's biosafety plan, as the two statements contradict each other. See below: NASA's uninhabitable Mars finding rebutted by its own cite, and by NASA's own plans to test for life in its samples .
- If NASA continues to say any health effects would be negligible because Mars microbes wouldn't be adapted to human hosts, health professionals can be expected to challenge the presenters with many examples of microbes not adapted to an infections lifestyle in any organism such as tetanus and Aspergillus fumigatus NASA's Biological Safety Report's finding of near zero risk that martian life can harm us rebutted by soil pathogens like tetanus and aspergillus fumigatus not adapted to any terrestrial host
- If NASA continues to say any life from Mars would not be viable on Earth, scientists outside NASA are likely to mention the many examples of terrestrial Mars analogues that do well in Mars simulation chambers. If NASA continue to use the same evidence to support their findings as they used for this PEIS, scientist are likely to look at their cite and find that the only Mars analogue extremophile in it grows up to human blood temperature. See below: NASA's Biological Safety Report's finding that any martian microbe would not be viable on Earth rebutted by the only plausible Mars analogue in its cite: Planococcus Halocryophilus
- if NASA uses their final argument to deduce from a low risk of significant environmental effects to a conclusion that environmental effects would not be significant, experts on risk assurance would ask NASA for clarification in the same way Chester Everline did for this PEIS as this is not standard probabilistic risk assurance. It's like deducing from a low risk of a house fire that a house fire would not be significant. See below: NASA's novel deduction that there would be NO significant ecological impacts, based only on its assessment that samples are UNLIKELY to pose a risk of significant ecological impact
NASA would also be certain to get many questions from the public concerned about a risk of unprecedented harm to human health or the environment, similar to the several dozen such comments they got on this not very well publicised PEIS. See Most public comments share Sagan's priority that NASA can't take even a small risk of large-scale harm .- A reporter might ask the same question Logan Greger did ":Are you certain that in any way, this mission won’t end with the total annihilation of the entire planet, or force us to live in biomes for the rest of time?"
Suppose NASA answers as in the final PEIS:
NASA's standard response to public comments raising concerns of unprecedented levels of global harm from this mission, even concerns about mass extinction: "the risk cannot be demonstrated to be zero" jars with their conclusion in the main body of the statement that any environmental risk would not be significant and any public health impact negligible
NASA's team clearly believe this to be satisfactory answer to Greger. But the general public may not find such answers acceptable. This might to lead to a public credibility gap between this statement and NASA's statement that any environmental effects would not be significant.
After press briefings in the 2030s with responses similar to NASA's official responses in the PEIS, the president may appoint an independent commission to check its plans. This could lead to a requirement on NASA not to return the samples until the commission has completed its investigation and until NASA have addressed any issues raised by this investigation. The worst case for NASA here is a decision that the samples have to fly past Earth and can't do the final course maneuver to land on Earth.
Then, depending on how NASA handles the situation and how the public respond to it, this could also lead to loss of trust in NASA. There may also be a risk of infodemic, conspiracy theories and viral claims that NASA is lying and covering up something. Such claims happen anyway, especially about asteroids, or claims that NASA astronauts never went to the Moon, or that NASA has a secret base on Mars, but there is a potential for this to be amplified in a situation of intense public scrutiny of NASA's plans for returning these samples.
We have seen how infodemic can lead to a lack of confidence in science, for instance with vaccine hesitancy for COVID vaccines. Pandemic planning before the COVID pandemic did consider the potential for infodemic but the amount of disinformation and misinformation was far more than expected leading to the need to develop the new discipline of infodemiology to study and respond to it similarly to epidemiology for diseases (Zielinski, 2021, Infodemics and infodemiology: a short history, a long future)
In the worst case scenarios here, issues of public trust could make it hard for NASA to return even thoroughly sterilized samples from the diverted mission.
If NASA's plan survives pitfalls 1 to 5, unsterilized samples are returned to Earth. The risk of harm likely remains very low as experts all say it is about averting a low risk of large-scale harm. Most likely there is no life in the samples as they are returned from a region with low likelihood of habitats and from a mission that is not looking for microhabitats or life in Jezero crater. Or if there is life in the samples the first microbes returned most likely are easily contained or unable to spread on Earth or harmless or beneficial.
However this would not prove that all future samples returned from Mars will be harmless.
- A professional astrobiologist would not be able to use the unsterilized samples to say it is safe for humans to go to Jezero crater or to say that there is no martian life in the Perseverance samples. That's because it is not a life detection mission, is not looking in places likely to find life even in Jezero crater, and all samples would go to hold and critical review due to the 8.1 ppb of terrestrial contamination until more information can be found with in situ searches on Mars. It would not be possible for an astrobiologist to prove that the terrestrial contamination it returns is not martian in origin. See: No possibility of a genetic inventory of terrestrial contamination: over 1000 genera had too few reads to identify, with enough organics to mask thousands of ultramicrobacteria from Mars
- Supposing humans go to Mars anyway, it is hard to land multi-ton payloads gently on Mars requiring either a parachute or ballute far larger than any tested so far which may tangle or rip. This may be the safest approach but it would take a lot of preliminary research with large ballutes in the upper Earth atmosphere. Alternatively in SpaceX's plans their rocket uses aerobraking well below the height of the southern uplands and then has to tip over from near horizontal to vertical using its thrusters, with only hundreds of meters of clearance, and may crash. If this happens, it likely starts the anthropocene on Mars with debris spread over thousands of square kilometers as for the Space Shuttle Columbia crash, with fragments of biofilms later spread in the dust storms. See: Exploring Mars from orbit avoids the dust and risk of a Columbia type crash on Mars
- Supposing humans land on Mars safely; the main dialogue in astrobiological journals is about how to do
as much astrobiology as possible before humans get there. The assumption on both sides of this
argument is that the anthropocene starts when humans get there, i.e. that Mars from
then on will be contaminated with terrestrial life in a way that can't be reversed. If there are habitats for terrestrial life on Mars, it will eventually spread throughout the planet. So this is a plausible future scenario according to all those currently discussing the topic in peer
reviewed papers.
See: Astrobiologists debate how much they can do before humans irreversibly introduce terrestrial life to Mars
There is a dissenting voice here, Chris McKay
See: Chris McKay's view that human missions to Mars can be biologically reversible and any terrestrial contamination should be reversed if we find a second genesis on Mars
However the many knowledge gaps identified by the SSB review mean we can't decide between these two views at present. See:
Life in the uninhabitable - the Space Study Board's thought-provoking review of SR-SAG2 commissioned by NASA and ESA a year later - If we get as far as 8, humans on Mars, without any of the previous steps stopping the mission we still don't know that Mars is safe for humans or Earth. Also, the level of terrestrial contamination from a human landing will make it almost impossible to find any rare native life that might still survive, if Mars ever had life, to assess whether it is safe or to study it. We will only be able to spot native life easily if it is distinctive and remains reasonably abundant after the start of the anthropocene.
- If present day Mars had only uninhabited habitats before the anthropocene, as some astrobiologists think likely, then by 8, humans on Mars,
humanity has also lost its opportunity to study a terrestrial planet in the habitable zone in its
prebiotic or early biotic state before introduction or evolution of life or the first steps of early life. We have lost that
opportunity not only for our generation and for our civilization, but for all future civilizations in our solar system. The nearest
Mars analogue planet is sure to be at least light years away, the distance to the nearest stars.
See: Mars’ potential uninhabited habitats as precious resources, the only nearby analogues of early life, prebiotic or abiotic chemistry on a terrestrial planet, vulnerable to contamination by terrestrial life - Colonists may find that the anthropocene has led to biofouling of underground reservoirs of water,
and they may also find that consortia of terrestrial microbes have turned subsurface aquifers into
calcite (cement) as two of the foreseeable consequences of terrestrial contamination mentioned in
the planetary protection literature. It may be possible to avoid such issues in a carefully planned rather than accidental anthropocene.
See: How introducing microbes in the wrong order in an accidental anthropocene could harm human prospects on Mars - If the plan is to attempt terraforming Mars or to try ecopoesis, to return Mars to a state
suitable for native life, the new terrestrial microbes of the martian anthropocene may predate on
the beneficial microbes we attempt to introduce. They may also compete with them or in other ways make the terraforming or
ecopoesis project impossible. For instance introduced life may eat or compete with algae we attempt to use
to convert CO2 to oxygen or methanogens that we may introduce to try to use a methane boost to speed
up warming of Mars, or any nitrogen liberating microbes if we find large stocks of nitrates on Mars for
the nitrogen which we'd otherwise have to source from comets. The accidentally introduced microbes
may rapidly fix CO2 to carbonates (for instance in diatom cell walls), convert nitrogen
gas to nitrates, and convert methane to various complex organics in anoxic conditions at source
before it can reach the atmosphere (as happens to most methane liberated below ground level in peat
bogs that form after melting permafrost) or oxidize any methane rapidly to carbon dioxide and water
(as happens to most methane liberated below ground level in dry soil in melting permafrost).
There is considerable uncertainty about how the terrestrial permafrost will respond to global warming, not because of issues with the physics, but issues with predicting how the permafrost would respond biologically. There is so much uncertainty that current models can't predict conclusively whether the melting permafrost will be a carbon source or a sink for the atmosphere (IPCC, 2019, Special Report on the Ocean and Cryosphere in a Changing Climate : section 3.4.3.1.1, Figure 3.11). There may be similar issues with modelling the effects of melting of the permafrost on Mars once we introduce terrestrial biology to the plant.
We have never done anything like this, introduce terrestrial life for the first time to another habitable planet. The Biosphere 2 experiment is the closest we have, not very close, and it ran into unexpected problems with loss of oxygen because of unpredicted reactions involving the concrete (Severinhaus et al., 1994, Oxygen loss in Biosphere 2). We may want to test our ideas for ways to introduce microbial life to Mars first in smaller space habitats before doing them on Mars itself. For instance the order in which the various species of microbes are introduced to Mars may change the outcome in major ways. It may matter if you introduce the photosynthetic life first, or the methanogens or methanotrophs or nitrogen fixers or denitrifiers (nitrogen liberators). It may also depend on the phages and fungal microbial pathogens you introduce with those microbes, which may well change the eventual outcome in significant ways. There may not be just one future Mars but many potential futures depending on which microbes, bacteriophages and fungal parasites we introduce in which order.
There is also a risk that introduced life on Mars may evolve novel capabilities as it rapidly radiates throughout Mars in the novel extreme conditions. These might then lead to similar issues to the issues with returning life from an inhabited Mars with a distant past common ancestor. Again a planned process may be able to reduce and manage these risks.
For some more details of these potential issues see blow : How introducing microbes in the wrong order in an accidental anthropocene could harm human prospects on Mars
The aim of this book is to find another way forward that avoids all those pitfalls and which keeps our options open until we can fill knowledge gaps and make an informed decision as a civilization.
My final comment on the draft PEIS and the theme of this book is:
Let's make this an even better mission and SAFE for Earth.
(Walker, 2022, Comment posted December 20th)
Roadmap for this book in sixteen steps
The aim of this book is to help find ways forward for NASA and other space agencies and private space companies as they gear up to send humans to the Moon and elsewhere in the solar system. It may not seem so at first as this book begins with harsh criticisms of NASA's plans which we need to look at closely as the first ever such Programmatic Environmental Impact Statement. However we have to cover the issues first to motivate the solutions.
It may help the reader to have an outline of where this book is headed from the outset. This is like providing a roadmap for a road trip before we set out.
- Major issues with NASA's current plans and its Programmatic Environmental Impact Statement which also raise major issues for any plan for unsterilized samples returned to labs run by human technicians
- Leading to a proposal for a way forward that plays to NASA's excellence in space, with a miniature
life detection satellite in a safe orbit above GEO. Humans go nowhere near the satellite. This is
based on a joint ESA / NASA plan from 2016 an in situ life detection lander on Jupiter's moon
Europa. This is a scaleable solution for any space agency or private space companies. Anything
returned to Earth is sterilized, Anything unsterilized is returned to satellites in safe orbits
above GEO.
See: Miniature life detection lab above GEO: a teleoperated alternative that plays to NASA's strengths, modelled on its proposed in situ Europa life detection lander - A respect for the unknown, there are scenarios consistent with current knowledge where it's never
biologically safe to land humans on Mars and return them. This is motivated by vivid planetary
protection scenarios such as life based on mirror organics, or a novel fungal genus our biology has
never seen before,
See: Detailed scenarios of mirror life and a novel fungal genus to motivate the need for great care in biosafety planning to protect Earth - NASA's end goal of a human presence throughout the solar system doesn't change. However, prior to knowing if Mars is safe to land on, they don't land human habitats or pressurized rovers on Mars. Anything deployed to the surface has to be dual purpose, for instance rovers that can be operated by telepresence. Otherwise we risk expensive stranded assets if we find the surface of Mars is not biologically safe for humans. If Mars is safe, many of the tools to keep exploring with humans in situ are already on ground.
It will also be difficult to sterilize complete pressurized habitats and rovers of all terrestrial life in order to do a reasonably thorough search of Mars for possibly hard to detect native life, while small robotic landers are far more easily sterilized.
In this vision, humans on Mars need to be a secondary goal for now, because its feasibility is outside of our control. Whether we can do this safely is dependent on what we find out on Mars.
See: A more resilient way to achieve NASA's end goal of humans throughout the solar system with an intermediate goal to explore Mars from orbit, with In Situ Resource Utilization (ISRU) on its moons - If Mars turns out to be biologically safe for humans, it is still likely to be some time before we can send humans to the surface of Mars. Sending humans to the martian Moons first as an intermediate step won't even delay things significantly. We don't yet have a life support system capable of operation for more than a few months without resupply from Earth and constant supply of components to repair it or consumables.
See: Reality check on timescale: when we have the capability to send humans to Mars safely we will also be able to run a human base on the Moon that needs resupply only every few years and following sections.
Then once we get to Mars it is far safer to explore from orbit than to land on the surface. The landing on Mars is exceptionally difficult with many failed attempts at robotic landings. This will be even more difficult for multi-ton human landings. NASA has abandoned the idea of using enormously large parachutes or ballutes for now because of the risk of the parachute tearing or tangling.
SpaceX's plan is currently the most favored approach for landing humans on Mars. This involves the rocket flying first sideways to the direction of travel to present as much resistance to the atmosphere as possible, then a phase of supersonic retropropulsion, flying backwards, skimming within hundreds of meters of the surface to use the thicker near surface atmosphere for aerobraking. Then finally the rocket as it slows down further, gradually tilts over to a vertical position for the final landing while still skimming the surface. It is also impossible to take enough fuel to the surface to take off again so the rocket has to be refueled on the surface after landing. This also means that the landing can't be aborted if it starts to go wrong when the rocket is about to land. Also all this happens too fast for human reaction times to respond to any developing emergency.
See: Exploring Mars from orbit avoids the dust and risk of a Columbia type crash on Mars
By comparison, landing on Phobos would be easier than landing on the Moon. Phobos is also interesting for the search for life as it is sure to have pristine meteorites thrown up from impacts into the early Martian oceans, and seems to be a likely source of water, and for first steps in In Situ Resource Utilization (ISRU) as far away as Mars. It is also a perfect base off planet for exploring Mars via telepresence. - We can however keep the focus on preparation for humans throughout the solar system, and also for a landing on Mars if this is biologically safe. The intermediate goal is humans to Mars orbit and to the Martian moons as for Lockheed
Martin's "Stepping stones to Mars", the HERRO mission, Martian base camp and the 2012
telerobotic conference [add links]
See: A more resilient way to achieve NASA's end goal of humans throughout the solar system with an intermediate goal to explore Mars from orbit, with In Situ Resource Utilization (ISRU) on its moons - We still have ISRU and our first tests in closed system habitats but in Mars orbit and on the
Martian moons. CO2 is a waste gas to be scrubbed in human habitats. In a closed system
the CO2 exhaled by humans plus a small amount from processing excreta exactly balances
the CO2 taken up by crops as they grow. if any food needs to be imported astronauts
exhale more CO2 than is needed to grow their crops. The external Martian atmospheric pressure at
less than 1% of the terrestrial atmospheric pressure has little effect on the engineering to contain
even a low pressure greenhouse for plants only (not breathable) at 10% of terrestrial atmospheric
pressure (Bucklin et al., 2002, Low Pressure Greenhouse Concepts for Mars:
Atmospheric Composition : figure 6). Later on we can grow crops on the surfaces via telepresence if it turns out to be biologically unsafe to send humans there. Seeds can be sterilized (unlike humans).and can be grown in sterilize aquaponics.
See: We can grow food in greenhouses on Phobos in low gravity as easily as on Mars and if Mars has hazardous indigenous life, we can grow it on Mars by telepresence - The first priority for robotic missions is a rapid biological exploration of Mars. These are
operated first from Earth and then from orbit around Mars. All assets on the surface of Mars are
dual purpose. So for instance we can generate fuel for rovers but the rovers would be
telerobotically controlled from orbit and so don't need to be pressurized. Fast unpressurized rovers
will be useful for reconnaissance and other duties if we do send humans to the surface.
See: A more resilient way to achieve NASA's end goal of humans throughout the solar system with an intermediate goal to explore Mars from orbit, with In Situ Resource Utilization (ISRU) on its moons - It is vital to keep Mars free of terrestrial life or it will be almost impossible to find out if there are a few martian microbes of novel biology or capabilities amongst the terrestrial contamination.
- However we have the technology to achieve 100% sterile landers due to remarkable advances in high temperature electronics, with silicon on insulator chips at 0.35 microns resolution able to run indefinitely at 300°C, video cameras that work in ovens, and many other capabilities including the Venus HOTTECH, with plans already available for a lander that could survive several months at 500°C on Venus.
- To achieve 100% sterile landers and rovers on Mars they need to withstand heating for a few
minutes, an hour is likely fine, to 300°C which is enough to evaporate and destroy amino acids
and DNA bases, not just break the bonds but vaporize them. A natural first here would be a 100%
sterile Marscopter, if Curiosity had such it could have used it to study its candidate RSLs close up
to see what they are with no risk of forwards contamination.
See: How to do a rapid 100% heat sterilized unmanned biological exploration of Mars using a simpler version of NASA's Venus HOTTech - The HERRO study found that one team in orbit can do as much as three teams on the surface in this
way (ADDCITE). Our capabilities for telerobotics are greatly increased since then so the ratio is likely
greater. Astronauts on the surface may not be able to travel that far from their base but may be
limited like the lunar astronauts to be close enough to walk back with the oxygen supply in a
space-suit in case of irreparable damage to the rover. And the lunar spacesuits reached end of life
at 2-3 days because of the lunar dust. There are numerous challenges on the surface of Mars such as
the perchlorates, the dust, the dust storms, that astronauts won't encounter anywhere else in the
solar system, while the ISRU in Mars orbit and using the Martian moons presents challenges that
prepare astronauts for the asteroid belt, NEOs, exploring Venus from orbit, Mercury, Callisto and
beyond.
See: A more resilient way to achieve NASA's end goal of humans throughout the solar system with an intermediate goal to explore Mars from orbit, with In Situ Resource Utilization (ISRU) on its moons
Also, see: Dust as the great inhibitor on Mars and the Moon
Also, see: Lunar dust is more easily managed than Martian dust and likely similar to dust found on larger asteroids and perhaps the regolith of Phobos too
Also, see: The spectacular HERRO orbit skimming close to Mars twice a day at local midday for our first telerobotic explorers of Mars - So in terms of NASA's end goal this apparent diversion to missions in orbit around Mars is on a more direct path than missions to the surface and it is future proof for whatever we find on Mars, and indeed if we find life based on mirror organics, this would stimulate space exploration and humans in space even if it meant that humans can't ever land on Mars, but they can explore it from orbit.
- This book also proposes a new planetary protection agency, independent of NASA, with its own
funding, that can add a significant amount, perhaps up to 10% or more of the cost of any mission that needs it, based on the extra costs
of the Viking missions planetary protection. It would provide prizes, grants and incentives for 100%
sterile landers and would also provide infrastructure similar to public roads, in this case the main
infrastructure needed right now is broadband optical communication with Mars which could enable all
our assets on Mars to achieve an order of magnitude more science return, at present we operate
landers on Mars with a cadence that would work equally well on Pluto due to the low bandwidth.
See: An independent body to safeguard Earth and Mars: avoiding perverse incentives to reduce safety by ensuring planetary protection costs don't come from NASA's budget - This proposal is future proof. We are making decisions not just for us but for all future
generations. If we return a microbe from Mars with a different biology then it is an irreversible
change to Earth's biosphere. In the forwards direction if we introduce terrestrial life able to live
on Mars the nearest terrestrial planet that hasn't been colonized by terrestrial life will be light
years away. We make it impossible not just for our own scientists but also for all future
civilizations in our solar system to study a pre-anthropocene terrestrial planet. We also initialize
the new terrestrial biosphere of the martian anthropocene to the start of a random future trajectory
based on whatever microbes we happen to introduce accidentally with the first human missions.
Depending on what we find there Mars could be far more valuable without terrestrial life than with it. There are many places we can send humans in our solar system that have no planetary protection issues right now including the Moon (apart from probably some lunar park areas to be kept clean), Callisto eventually (with its ancient unaltered craters, the same planetary protection classification as the Moon) and Titan (needs evaluation but even if it has some exotic life, this is highly unlikely to be able to survive on Earth, For terrestrial contamination, though there is some evidence suggesting cryovolcanoes, they cover only a small part of its surface and the water in the cryolava is likely to freeze as the methane boils and to have large amounts of ammonia making it likely uninhabitable) (Sogin et al., 2012. Assessment of planetary protection requirements for spacecraft missions to icy solar system bodies. 39th COSPAR Scientific Assembly, 39, p.1854.). We don't need to rush into a decision for Mars and the Moon is by far the best place for a backup of seeds, knowledge, etc., with its cold traps at the poles at liquid nitrogen temperatures.
See: How introducing microbes in the wrong order in an accidental anthropocene could harm human prospects on Mars - Many have been inspired by the idea of Mars as a way to add a second terrestrial planet like
Earth.
What happens to that vision and inspiration if Mars is a mirror life planet or in some other
way is biologically unsafe? Or what happens if we find microbial treasures of completely novel
biology on Mars that of great value on Mars that could be extinguished by terrestrial microbes?
The Stanford torus study in the 1970s suggested another inspiring idea of human habitats spinning for artificial gravity. These ideas have never been tested with experiments in space, but we'll find that there is some evidence from experiments in Skylab and anecdotal observations of astronauts in the ISS suggesting humans completely lose the feeling of nausea even when spinning really fast in micro gravity. There are several low cost tests we could today that could quickly evaluate the feasibility of artificial gravity and the spin tolerance of humans when there is no gravity or low gravity acting along the spin axis.
Low cost artificial gravity could open up many opportunities both for healthy long distance travel in the solar system and also for settlement of the smaller moons and asteroids, including the martian Moons.
See: Artificial gravity: promising data from Skylab and parabolic flight could be followed up by low cost small centrifuges and tether experiments in orbit with the potential to greatly expand options for space explorers and colonizers
This book touches on these points because of the importance of bringing everyone together on this path, of finding an inspiring future that we can all subscribe to. If part of the community is not happy with the direction we are taking it will be far harder to follow this path.
Especially we need private space companies to be inspired to support a rapid biological exploration of Mars. The martian moons can either be seen as stepping stones to humans living on Mars, or as small asteroids orbiting a fascinating planet which we'd always explore from orbit and stepping stones to the asteroid belt and to use of the Near Earth Objects also for making space habitats, and perhaps also using materials from the Martian moons to
construct habitats around Mars itself.
Phobos may have a substantial ice content and there is enough material for radiation shielding for a habitable area in orbit around Mars constructed only of materials from Phobos equivalent to a combined area of Texas, California, Montana, New Mexico, and Arizona or in terms of European countries, France, Spain, Sweden, Norway, Germany and Finland. So the potential habitability of the martian moons alone is perhaps more than most would expect. There may well be more ice available in Phobos than in Jezero crater for a first base since so far no ice has been discovered in Jezero crater reachable from the surface. It's likely to be some time before we have a Mars colony with a population greater than the combined population of those states or countries. We could use Phobos as a stepping stone to humans on Mars or a stepping stone to the habitable area of 1000 terrestrial worlds using materials from the asteroid belt, or of course both if we get a biosafety green light to land humans on Mars.
See: Phobos may have abundant ice and has mass for radiation shielding of habitats spinning for artificial gravity with land area of Texas, California, Montana, New Mexico, and Arizona
Also, see: Asteroid belt materials can form the living area of a thousand new worlds as habitat bubbles if humans can't land on Mars
While if we find it is biologically safe to land on Mars this approach leads to a far more robust way to explore Mars, far safer and more resilient with a gateway already established in Mars orbit for the rapid astrobiological survey
See: If we determine it is biologically safe to land on Mars, the surface colony will be far more resilient with a "Mars gateway" settlement in orbit
In this way we don't need to be rushed into making decisions about Mars based on inadequate knowledge and guesses to try to fill knowledge gaps. We can find out what we have in the only other terrestrial planet in our solar system and THEN decide.
I hope this vision will be inspiring to the reader, and may stimulate ideas and other suggestions.
Some readers may be interested to jump ahead to the conclusions to see where the reasoning in this book is headed at this stage before continuing:
NASA's PEIS as a likely model for future impact assessments by private space and other space agencies globally
This book focuses on NASA’s Programmatic Environmental Impact Statement (PEIS) because it is the first environmental impact statement for a Mars sample return ever published. As such it is likely to be influential on other space agencies and private space companies. It is also published by NASA which was formerly known for its excellence in planetary protection.
For both these reasons this PEIS can be expected to be a model for how to do environmental impact assessments for Mars sample returns in the future by private space, and other countries such as China, India, UAE, Japan, etc. This makes the PEIS itself of global importance as a precedent and template for the future.
Although experts are of the view the risk of large-scale harm to human health and the environment is likely low, it is not demonstrably zero (Ammann et al., 2012, Mars Sample Return backward contamination–Strategic advice and requirements 20, 24, 58) (SSB, 2009, Assessment of planetary protection requirements for Mars sample return missions : 48). When we build houses we pay a great deal of attention to the very low risk of a house fire. In the same way plans such like this need intense scrutiny. NASA sadly stepped back from its world leading role in planetary protection,
We find an interesting historical parallel in the Apollo era. NASA told the Apollo 11 astronauts to open the door of the capsule and exit into a dinghy in the open ocean.
Other agencies advised them against this plan. Vishniac of the National Academy of Sciences told NASA that:
Opening and venting the spacecraft to Earth’s atmosphere after splashdown would, in his view, make the rest of Apollo’s elaborate quarantine program pointless.
(Meltzer, 2012, When Biospheres Collide : 452).
However NASA ignored their advice.
The astronauts, as advised by NASA, opened the door of the capsule while it was still floating in the sea. Some lunar dust must have got into the ocean at that point.
Then divers swiftly swabbed down the outside of the astronauts' biological containment garments with a bleach solution, and they were lifted up into a helicopter and flown to the ship. Meanwhile the divers washed down the dinghy with an iodine solution and weighted the dinghy and swabs to sink them into the ocean.
Text on graphic: Astronauts swiftly swabbed down with bleach - then the cloths were weighted and dropped into the ocean. Background image: Apollo 11 crew await pickup by a helicopter from the USS Hornet (NASA, 2013ach).
In the dinghy are the Apollo 11 astronauts Neil Armstrong, Michael Collins, and Buzz Aldrin, and Navy Frogman Clancy Hatleberg
The bleach and iodine solutions used by the divers wouldn't have sterilized the dinghy or the external biological containment garments of the astronauts even with the understanding of the day. For details see:
Similarly this century. NASA closed down the interagency panel and the planetary protection subcommittee after they had already ceased to serve any useful purpose, then finally closed down their planetary protection office.
This is a brief summary by the Space Studies Board.
To be effective, planetary protection measures should be integrated into the engineering and design of any sample-return mission, and, for an oversight panel to be in a position to coordinate the implementation of planetary protection requirements, it should be established as soon as serious planning for a Mars sample-return mission has begun.
… the Planetary Protection Advisory Committee (PPAC) was formed in 2000
… In 2006, … the PPAC became a subcommittee under the NAC’s Science Committee.
… by being subordinated under a committee that focused on the science missions, the new planetary protection subcommittee lacked the level of independence necessary for effective planetary protection.
… By 2016, the committee had become completely moribund, and it was formally disbanded in late 2017.(SSB, 2018, Review and Assessment of Planetary Protection … : 26 - 27).
Finally, NASA closed down its planetary protection office, which had some measure of independence from NASA, able to review NASA's decisions. This office had also developed a great deal of expertise in planetary protection for a Mars sample return mission. It operated from 1997 to 2017 (Voosen, 2017, With planetary protection office up for grabs, scientists rail against limits to Mars exploration).
NASA's current planetary protection engineer is now an employee in its Office of Safety and Mission Assurance, with no independence from NASA. I cover this below:
The issues we find in this PEIS are not likely to be unique to NASA. They are systemic issues we can expect to find in other space agencies of other countries, and in private space when they start to return samples from Mars in the 2030s.
It is natural for a space agency or private space to be focused on mission goals and on engineering achievements. They are unlikely to have in-house expertise in planetary protection, biosafety, human diseases, alien life, and the other topics necessary for adequate biosafety plans to protect Earths biosphere.
Given that even NASA responded like this not just once but twice at the time of Apollo and today, it is likely other space agencies will do the same. So, we need to find a way forwards that works not only for NASA but for other space agencies and also for private space, but also protects Earth and its inhabitants adequately.
So, we can't expect space agencies or private space to have the in-house expertise to prepare a biosafety plan. But who else can prepare a biosafety plan for them? The systemic issues we find here suggest that they will not find it easy to work with other agencies on biosafety.
This issue is compounded by the ESF recommendation in 2012 to contain all particles of 0.05 microns and larger with 100% containment, a requirement no current air filter technology can achieve. It gave 0.01 microns as the preferred size limit and required expert review before permitting particles of 0.01 microns to 0.05 microns.
- Why NASA can't rely on HEPA filters for the ESF recommendation to contain 100% of ultramicrobacteria
Also any biosafety laboratory has to have precautions in place to respond to lab leaks. However for alien life, Carl Sagan raised the vexing issue of the latency period which is now known to be up tot 20 years for his example of leprosy. He could also have mentioned lifelong symptomless carriers like typhoid Mary.
He could also have mentioned Aspergillus fumigatus (which causes a serious disease in immunocompromised with fatality rate of 35% to 90% in 200,000 a year and the opposite problem of a progressive allergic lung disease in 400,000 a year). It is one of the most common microbes on the ISS and all the astronauts go through health stabilization before they fly (NASA, n.d., Health Stabilization Program)but it is harmless to young healthy astronauts who indeed would be disqualified from flying if they had a serious allergic reaction to Aspergillus. Similarly a fungal disease of crops can’t be kept out or mirror life, or many other diseases.
As the WHO say even the most well prepared labs can have lab leaks for many reasons (WHO, 2020, World Health Organization Laboratory biosafety manual, 4th edition : 87). The lunar receiving facility in the Apollo era had many lab leaks (Degroot, 2023. One Small Step for Man, One Giant Leap for Moon Microbes? Interpretations of Risk and the Limits of Quarantine in NASA’s Apollo Program : figure 5).
Based on these examples even if we can achieve the ESF recommendation of 100% containment of all particles of 0.05 microns and upwards, we would still have no way to respond to lab leaks that is able to contain unknown pathogens or unknown disruptive organisms like mirror life that might have contaminated human technicians.
Perhaps this can be resolved by a first of its kind fully telerobotic terrestrial laboratory. However it may need end of facility life time sterilization of everything inside including the telerobots, control systems, anything that could contain mirror life after decades of work studying the samples remotely using telerobotics that may have involved several incidents where there was a lab leak but one that only affected the telerobots. It is also likely to be very expensive and is not a natural fit to NASA's areas of excellence as an agency, or of any other space agency or of private space
Those considerations lead to the alternative solution that I proposed to NASA in both rounds of public comments under NEPA, of a miniature lab above GEO based on a joint mission proposal by NASA and ESA from 2016 to send a life detection lab to search for life in situ as far away as Jupiter's moon Europa.
This is a way forward that doesn't require NASA or private space or other space agencies to do biosafety plans. Everything returned to Earth is sterilized.
One of NASA's main priorities as an organization is to facilitate sending humans throughout the solar system. This is also a priority for private space and other space agencies.
At our current level of understanding one possible outcome is that we find that Mars is biologically unsafe for humans, with anything from a novel fungus that human biology has no defences against through to mirror life. So we need plans flexible enough to be resilient to such discoveries. habitats.
Carl Sagan talked about the need for an “exhaustive program of unmanned biological exploration of Mars”. We can actually achieve 100% sterilization by heating landers to 300°C which would completely destroy amino acids and DNA bases. Silicon on insulator chips can be run indefinitely at 300°C and there are many modern components we can use including some in development for your Venus HOTTECH program that can even withstand 500°C for months on end.
Most of the components for a Marscopter seem to fit this specification and a 100% sterile Marscopter might well be useful for a future mission, for instance Curiosity could have used it to explore the potential RSLs in Gale crater. That then can be a starting point for many future versatile and mobile landers on Mars.
This leads to an alternative vision where the In Situ Resource Utilization is done in Mars orbit and on the Moons of Mars. That is of far more applicability to missions elsewhere in the solar system while the unique issues of perchlorates, dust storms and the unique potential asset of a CO2 atmosphere require solutions that are unlikely to be of use anywhere except on Mars.
The astronauts in orbit would be of great value stepping in much as in the “Game of Civilization” to control rovers on the surface that face issues they can’t resolve through their own autonomy and impacted by the long round trip time for control from Earth. By then we likely have hundreds or even thousands of landers for them to use to explore Mars and they can “teleport” from one to another. This would lead to a far more interesting and varied experience for the astronauts too, with telepresence avatars on the surface. We see with the ISS how interesting it can be for astronauts to orbit a planet.
In this vision the surface assets on Mars are dual purpose, and we don't land any habitats there until we know if it will be biologically safe to land humans. This avoids the issue of stranded assets of unusable human habitats on a mirror-life Mars. We can still do things like rovers that use in situ production of fuel on Mars since this is dual purpose and such rovers can be useful for rapid in situ exploration so long as they can be 100% sterilized as they likely can be. But the experiments in human habitation would be done in orbit and on the Martian moons. We keep everything dual purpose until we know if humans can land on Mars and knowing that a possible answer here is "no" depending on what we find on Mars.
Let's imagine that we follow this idea of initial remote exploration of Mars from orbit or from it's moons. What would be the worst case scenario, for the exploration in on itself? All of this effort and then Humans not being able to actually land on Mars? Well let's explore that a bit
If we find something as interesting as a mirror life planet on Mars, life independently evolved from mirror organics, this would invigorate human space exploration, with numerous other places in the solar system humans can explore. NASA themselves don't see Mars as a final goal for humans in space but as a staging post to the rest of the solar system.
If we find unsafe biology on Mars it can still be a stimulus leading to a permanent presence in orbit around Mars and likely to a settlement there which could use surface assets too, but remotely from orbit because it is not safe to land there. This would invigorate space settlement in habitats independent of planets which has potential to unlock a far larger habitable area for humans in our solar system over a far shorter period of time than terraforming.
I go into this in the paper starting from:
My hope is that as a result of this book the debate is opened up and many others are encouraged to submit their ideas similarly and a process is set up to evaluate them based on not just science return but also how well they achieve safety for Earth's biosphere and whether the way of keeping Earth safe is one that NSAA and other space agencies and private space can realistically be expected to implement at a reasonable cost and how this all fits into the future aims and visions of space agencies and private space.
NASA's four apparently plausible findings already rebutted in 2000
Before we can open up the debate for the public, we need to look at the arguments NASA present in the Programmatic Environmental Impact Statement (PEIS). At first sight they might seem to have shown that it is next to impossible for Earth's biosphere to be harmed by anything from Mars. We need to go into details because these arguments seem so plausible to many, especially to enthusiasts for humans to land on the surface of Mars as soon as possible.
These are the four findings again to summarize:
- any harmful life can get here better protected in meteorites (NASA, 2023. Mars Sample Return final PEIS : 3–3),
- Mars has been uninhabitable for millions of years (N 3. Mars Sample Return final PEIS : 1–6)
- martian microbes would have near zero chance to harm humans because of the lack of similar hosts on Mars (Craven et al., 2021. Biological safety : 6)
- any martian life would be unable to survive on Earth (Craven et al., 2021. Biological safety : 6)
Or more succinctly, that with certainty or near certainty:
- if there is life on Mars it already got here in meteorites
- Mars is uninhabitable
- any martian life would be unable to harm humans
- any life on Mars wouldn't be able to survive terrestrial conditions
These are the four rebuttals:
- How NASA's central finding that any harmful life can get here better protected in meteorites is rebutted by BOTH of its main cites for it
- NASA's uninhabitable Mars finding rebutted by its own cite, and by NASA's own plans to test for life in its samples
- NASA's Biological Safety Report's finding of near zero risk that martian life can harm us rebutted by soil pathogens like tetanus and aspergillus fumigatus not adapted to any terrestrial host
- NASA's Biological Safety Report's finding that any martian microbe would not be viable on Earth rebutted by the only plausible Mars analogue in its cite: Planococcus Halocryophilus
With these four findings, NASA presents a description of planet where humans can go land, raise a flag, build a settlement and do what they like just as they do on the Moon, with no risk of any harm to either Earth's biosphere or Mars' biosphere. The very first finding by itself would seem to be enough reason not to have any concern. If any life on Mars gets here frequently on meteorites what would it matter if we also bring some rocks back in our spaceships? They would be as harmless as moon rocks.
However if we look at the literature on this topic more closely, it turns out that the rocks we have from Mars come from deep below the surface of the high cold dry southern uplands where the air is very thin making it easier for a glancing impact to throw rocks into space. The best habitats for martian life are in the lower lying regions where the air is thicker, making it far harder for an impact to eject material into space, and we have no recent meteorites from regions of Mars at the same altitude as Jezero crater, which are also the easier altitudes for our spacecraft to land because of the thicker atmosphere.
What's more, the best place for life to live on Mars is either in deep caves or in small microbial oases in the dust, dirt and salts and ice in the top few centimeters of soil. Life in caves is highly unlikely to get into space after an impact. Meanwhile, life in the surface microbial oases can't be ejected at all, the surface dust, dirt, salts, and ice, would never get into space after an impact.
Also only a few terrestrial microbes are so hardy that they could survive the shock of ejection, fireball of exit from Mars' atmosphere and reentry to Earth's atmosphere, and the cold and desiccating vacuum of the journey through space. If there is life on Mars it's possible that many or even all native life has never developed those adaptations, while the sample tubes are like miniature spaceships for a microbe complete with a small amount of atmosphere at Martian surface atmospheric pressure.
So the Mars meteorite argument is far less persuasive than it might seem at first. NASA's own main cite for their Mars meteorite finding makes this very argument that the samples may come from materials that can't get into space, concluding:
Finding: The committee finds that the content of this report and, specifically, the recommendations in it do NOT apply to future sample return missions from Mars itself.
(SSB, 2019, Planetary protection classification of sample return missions from the martian moons : 45)
NASA's team do not seem to have noticed this rebuttal in their own main cite for their Mars meteorite argument. We cover this in the next section:
It is the same for the other three findings. As soon as you dig in deeper and read NASA's own cites and other material on the topic you find they are ALL invalid. We need to look at the cites here in order to show that their arguments for these findings are invalid and will do so in the next few sections.
That then opens the debate up. Those who think we need to do more to keep Earth's biosphere safe from potential life from Mars have valid concerns. These not only need to be addressed but will be addressed at some point.
We see these concerns in the many public comments on this not very well publicised PEIS.
There will be many more such public reactions and comments in the future as it gets more publicity. The main reason there hasn't been more reaction to date is NASA's decision at the outset of the NEPA process that any effects would be local to the Utah Test and Training Range, and that the PEIS only needed to be publicised locally. See below:
- NASA's novel conclusion that any effects would be local to the Utah Test and Training Range, and not significant
- Impact of limited scope for tier 1: NASA only publicised its PEIS and scoping meetings in newspapers local to the Utah Test and Training Range and Kennedy Space Center
All the major studies on planetary protection for a Mars sample return mission make this point, that NASA can only succeed in this mission if it has broad public engagement and it answers the public concerns with its plans. The sooner this debate starts the better and the purpose of this book is to help facilitate it. We cover this below in:
NASA can delay this debate by using these plausible seeming findings, but as we'll find, none of them are well grounded in the science. So NASA can't sustain its current position on the matter once its findings encounter any detailed level of scrutiny.
In particular it would only take one detailed expert review of NASA's plans, for instance by the US National Academy of Sciences or the European Science Foundation to overturn NASA's four findings and two conclusions in this PEIS. It seems certain this review will happen at some point in the next decade as there is more public attention on what NASA plan to do and as it becomes clear to the public that their novel findings and conclusions are not yet peer reviewed.
All four of NASA's four findings were first presented by Robert Zubrin, a Mars colonization enthusiast and president of the Mars society, in a non peer reviewed op ed (Zubrin, 2000. Contamination From Mars: No Threat)
Zubrin's four findings were immediately rebutted in the next edition of the Planetary Report by planetary protection experts including NASA's own first planetary protection officer who was also the lead author of the rebuttal (Rummel et al., 2000. Opinion: No Threat? No Way : 4 - 7). They first rebuttal of all four arguments in the peer reviewed literature was two years later by Clark (Clark, 2002, Martian meteorites do not eliminate the need for back contamination precautions on sample return missions). The Mars meteorite argument was rebutted again by the National Academy of Sciences in 2009 as we already saw (SSB, 2009, Assessment of planetary protection requirements for Mars sample return missions : 47 - 48) and again in 2019 (SSB, 2019, Planetary protection classification of sample return missions from the martian moons : 5) .
We will see that NASA doesn't get to its four findings based on new evidence or new sources. They also don't cite any previous publications of their findings or rebuttals of them.
Yet, because of the prestige of NASA and because this is the first ever Mars sample Return Environmental Impact Statement, if these arguments are not immediately challenged they could be used by other countries and by space colonization enthusiasts. If the findings were true it's not clear why we wouldn't just drop all planetary protection for Mars samples and for missions to Mars. The meteorite argument alone would be sufficient reason to drop all planetary protection if valid and is correctly used in that way for Phobos and for samples from NEOs. See below:
The Mars meteorite argument, if valid, would be a very major finding in the planetary protection literature. Indeed, any of these would be major findings. Any new paper that came to these conclusion would be amongst the most cited papers on the topic because of their planetary protection implications. So it shouldn't be a difficult task to find previous occurrences in the peer reviewed literature if any recent peer reviewed paper had come to similar conclusions to NASA.
Zubrin's non peer reviewed op ed in the Planetary Society monthly, the only previous occurrence of these four findings in the planetary protection literature, has only two cites listed in Google Scholar and both cites rebut his conclusions.
The first cite of Zubrin's 2000 paper by Clark et al. rebuts all four of Zubrin's findings, and says they can be readily discredited, with special focus on the Mars meteorite argument::
Recently, it has been suggested in print (Gladman and Burns, 1996; Gladman, 1997) and verbally by a variety of planetary scientists that the newly accepted reality that martian material periodically ejected from Mars and finding its way to earth as meteorites is "evidence" from which we might conclude that back contamination is of minor concern. This even has been extrapolated by some that there should even be no concern at all (Zubrin, 2000), in contrast with the recommendations of a study sponsored by the U.S. National Academy of Sciences, which concluded although the risk of contamination of Earth with significant impact is unlikely, the risk is not zero (p. 2, NRC, 1997). In this paper, additional aspects of this problem are examined to show that sweeping judgmental conclusions that the risk can be assumed to be zero on the basis of the existence of martian meteorites can be readily discredited on several grounds.
(Clark, 2002, Martian meteorites do not eliminate the need for back contamination precautions on sample return missions)
The second cite by Warmflash et al rebuts specifically Zubrin's conclusion that Mars can't have pathogens of humans:
certainly, we cannot depend on the following a priori conclusion, as expressed by a popular Mars colonization enthusiast, that “there is no evidence for the existence of macroscopic Martian fauna and flora. Without indigenous hosts, the existence of Martian pathogens is impossible”
(Warmflash et al, 2007, Assessing the Biohazard Potential of Putative martian Organisms for Exploration Class Human Space Missions : 14 - 15)
So let's now look at the arguments in detail. You can jump to any of the four sections of interest using these links:
- How NASA's central finding that any harmful life can get here better protected in meteorites is rebutted by BOTH of its main cites for it
- NASA's uninhabitable Mars finding rebutted by its own cite, and by NASA's own plans to test for life in its samples
- NASA's Biological Safety Report's finding of near zero risk that martian life can harm us rebutted by soil pathogens like tetanus and aspergillus fumigatus not adapted to any terrestrial host
- NASA's Biological Safety Report's finding that any martian microbe would not be viable on Earth rebutted by the only plausible Mars analogue in its cite: Planococcus Halocryophilus
Then we cover NASA's two major findings from novel plausible but invalid arguments later in the book:
How NASA's central finding that any harmful life can get here better protected in meteorites is rebutted by BOTH of its main cites for it
This is by far the most important of the four findings. It is so important a JPL risk assurance expert Chester Everline commented on NASA's plans saying they should focus on establishing this finding.
He also said that if NASA are unable to demonstrate its Mars meteorite argument convincingly, the risk from this mission should be considered unacceptable. He said in that case, they should leave the rocks on Mars and study them in situ until we know more about the risks.
A better statement of options should include the possibility of delaying the return of Mars samples until the risks associated with their return are better understood
…If the MSR Campaign is unable to convincingly demonstrate that the risk it poses to Earth is [...] less than an analog natural hazard [i.e. Mars meteorites] the risk from MSR should be considered unacceptable. If the MSR Campaign can convincingly demonstrate the risk it poses to Earth is less than an analog natural hazard, then returning samples using MSR is analogous to having another Mars meteorite impact earth. The MSR assurance case should focus on this.
(Everline, 2022, Comment posted December 20th)
[explanatory comment added in square brackets][removed redundant "not" in the red text marked as [...] ]
We discuss his expert opinion and his suggestion to defer the sample return below in:
As we saw above:
NASA themselves agree that the worst case result of this mission is a possible mass extinction in their response to public comments in the final PEIS though they don't say this in the main body of the PEIS.
If NASA continue to respond in this way, their Mars meteorite finding is essential and central to its plans. If this finding is incorrect, NASA depends on its other three findings, and if none of them hold up, here is no way for NASA to get the widespread public support it needs for this mission as currently presented.
However, NASA's Mars meteorite finding is rebutted in BOTH of the National Academy of Sciences cites it uses to support the finding in the PEIS. The reader isn't alerted to this. Indeed it seems clear that NASA's own Mars Sample Return team is completely unaware of these rebuttals from their responses to my public comment on the their draft PEIS. It gives no new evidence to override the assessments in NASA's two main cites that their main finding is invalid.
The way NASA presents their finding is that they say that any life on Mars can get here better protected in a Martian meteorite than in their sample tubes. This is a strong statement that is likely to seem implausible to the reader without very strong arguments in support of it.
I include screenshots for many of the quotes from the PEIS and also sometimes for other sources so readers can see the quotes without clicking through to the cite. Here it is in context with the relevant parts highlighted.
NASA:
One of the reasons that the scientific community thinks the risk of pathogenic effects from the release of small amounts (less than 1 kilogram [2.2 pounds]) of Mars samples is very low is that pieces of Mars have already traveled to Earth as meteorites
…
“The natural delivery of Mars materials [i.e. martian meteorites on Earth] can provide better protection and faster transit than the current MSR [Mars Sample Return] mission concept. First, potential Mars microbes would be expected to survive ejection forces and pressure (National Academies of Sciences, Engineering, and Medicine and the European Science Foundation 2019), …”
(NASA, 2023. Mars Sample Return final PEIS : 3–3)
However, this argument is already rebutted in the 2009 National Research Council cite. This is the most recent comprehensive study for planetary protection for a Mars sample return mission and NASA cites it in the paragraph before the one shown. In context the reader would naturally assume that "scientific community" that NASA is referring to in this paragraph includes the National Academy of Science authors cited in the previous paragraph but as we will see, it does not.
Everyone agrees that the martian meteorites come from Mars, and that there is some potential for exceptionally hardy bacteria like b. subtilis to get from Mars to Earth on meteorite if it has organisms like that there. What is not so sure is if any life from Mars DOES get to Earth in them, and if so if ALL species of life on Mars that can get to Earth in sample tubes can also get here in meteorites. To be safe from any life that we return in sample tubes we need ALL species of life that may exist on Mars to be able to get here on meteorites. For more about this see below:
This is how the National Academy of Sciences puts it in 2009 in NASA's own 2019 cite for "First, potential Mars microbes would be expected to survive ejection forces and pressure" .:
Section “Martian meteorites, Large-Scale effects and Planetary Protection” The potential hazards posed for Earth by viable organisms surviving in samples
is[are] significantly greater with a Mars sample return than if the same organisms were brought to Earth via impact-mediated ejection from Mars…
… Thus it is not appropriate to argue that the existence of martian meteorites on Earth negate the need to treat as potentially hazardous any samples returned from Mars by robotic spacecraft.
(SSB, 2009, Assessment of planetary protection requirements for Mars sample return missions : 47 - 48)
This is also where the National Academy of Sciences touch on the question of whether any past mass extinctions could be due to life introduced to Earth from Mars, as we mentioned. The cite doesn't give a specific example, but the Great Oxygenation Event is the one the immediately springs to mind (if it was a mass extinction). We look at this below:
NASA's 2019 National Academy of Sciences / European Science Foundation cite comes to a similar conclusion, with a more detailed discussion. Page 45 of NASA’s 2019 cite for this passage reads:
National Academy of Sciences, 2019 (NASA's cite)
The microbes may NOT be able to survive impact ejection and transport through space.
…
Finding: The committee finds that the content of this report and, specifically, the recommendations in it do NOT apply to future sample return missions from Mars itself.
(SSB, 2019, Planetary protection classification of sample return missions from the martian moons : 45)
In context:
National Academy of Sciences, 2019 (NASA's cite)
There are several reasons why Mars sample return (MSR) missions differ from those for collecting samples from Phobos and Deimos, including the following:
- … The collected samples will be selected according to specific criteria designed to maximize the chance of sampling evidence of extant or extinct life …
- The various physical processes evoked in the microbial contamination assessment (excavation by impact, collision with Phobos, sterilization, etc.) do NOT have to be considered for the assessment of potential microbial density for MSR, which could increase drastically the potential microbial density in comparison to the Phobos and Deimos sample …
- The reasoning regarding natural flux does NOT apply directly to samples returned from the Mars surface. The material will be gently sampled and returned directly to Earth. The sample may well come from an environment that mechanically CANNOT become a Mars meteorite. The microbes may NOT be able to survive impact ejection and transport through space.
…
Finding: The committee finds that the content of this report and, specifically, the recommendations in it do NOT apply to future sample return missions from Mars itself.
(SSB, 2019, Planetary protection classification of sample return missions from the martian moons : 45)
[In this passage, examples of sites that mechanically cannot survive ejection from Mars would include ice, dust, dirt and salts on the surface of Mars]
The 2019 National Academy of Sciences study explains that JAXA's arguments DO apply to samples Japan plans to collect from its moons. The reason is that any life there has already survived ejection into space after large impacts on Mars in the distant past and then an extra sterilizing step of impact onto the surface of Phobos at kilometers per second. We cover this later in this book::
So NASA's Mars PEIS team got confused by reasoning that is only valid for the moons of Mars, applying it inappropriately to the surface of Mars itself.
NASA's preparers didn't need a high level of familiarity with the planetary protection literature to spot this mistake. The preparers of the PEIS did need to read its 2019 planetary protection cite but only as far as page 5. See:
- Martian material present in a Phobos/Deimos sample would have undergone several physical sterilization processes (e.g., excavation by impact, collision with Phobos, and exposure to radiation), before it is actually sampled. Material collected on the surface of Mars will not have undergone such processes.
- MSR material might come from sites that mechanically cannot survive ejection from Mars and thus any putative life-forms would de facto not be able to survive impact ejection and transport to space. Such mechanical limitations do NOT apply for material collected on
MarsPhobos/Deimos.Therefore, the committee finds that the content of this report and, specifically, the recommendations presented in it do NOT apply to future sample return missions from Mars itself.
(SSB, 2019, Planetary protection classification of sample return missions from the martian moons : 5)
[Corrects obvious typo, Mars to Phobos. In this passage, examples of sites that mechanically cannot survive ejection from Mars would include ice, dust, dirt and salts on the surface of Mars]
There is an unfortunate minor typo there, Mars for Phobos/Deimos but it wouldn't confuse anyone who reads the cite carefully enough to note the emphatic NOT in the next section, or who is familiar with the planetary protection literature. Also, as we saw above, the more detailed presentation later in the book on page 45 doesn't have this typo (SSB, 2019, Planetary protection classification of sample return missions from the martian moons : 45).
I attempted to alert NASA;s team to this issue but to no avail. At the time I hadn't had time to read the 2019 cite, only dipped into it, so I didn't know about its rebuttal. However, I already was very familiar with the 2009 cite, having studied it in some detail as the most recent thorough study of planetary protection for a Mars Sample Return mission, and knew that it rebutted the meteorite argument.
This is their response to my comment: they don't seem to have noticed what I said even though they quoted it in the PEIS. I mention the 2009 NRC mass extinction argument. NASA's team doesn't seem to notice that as being of any special interest to respond to. It doesn't seem to notice my second paragraph where I say there is nothing in the 2009 cite to support the Mars meteorite argument in the paragraph that NASA attached it to. NASA doesn't seem to recognize that this is intended as highlighting an issue with the meteorite argument itself.
Highlighted text:
Robert Walker: NASA fail to adequately consider the risks from life that can’t get to Earth on meteorites 2009, the National Research Council examined the possibility of life transferred on meteorites said the risk is significantly greater in a sample return mission - and said they can’t rule out the possibility of large scale effects in the past due to life from Mars – NASA’s EIS instead claims microbes will survive transfer from Mars to Earth more easily in a meteorite than in a sample return mission but their sources don’t back this up
There isn’t anything here to support the thesis of the draft EIS that it is easier for Martian microbes to get to Earth on a meteorite than in a sample tube. (NASA, 2022eis: 3-3): “The natural delivery of Mars materials can provide better protection and faster transit than the current MSR mission concept.”
[NASA don't seem to notice the highlighted text and instead only respond to the start of the first sentence]
NASA: Additionally, the PEIS details and references on pages 3-3 to 3-4 information on the unlikely risks from “life that can’t get to Earth on meteorites.”
[those pages 3-3 to 3-4 are the pages that outline the Mars meteorite argument that my comment tried to draw their attention to as having a major issue with it]
(NASA, 2023. Mars Sample Return FINAL PEIS : B-59 - B-60)
The highlighted paragraph is from page 22 of my attachment (Walker, 2023. So many serious mistakes in NASA's Mars Samples Environmental Impact Statement it needs a clean restart) (docx) : 22). .
[The way my text is quoted is rather jumbled up here: the first paragraph quoted is from page 110 of my attachment, and the second paragraph is quoted out of context from page 22 where it is supported by several pages of text. The PEIS goes on to repeat my paragraph from page 110 a second time then continues through to page 111 of my attachment where it stops abruptly at a confusing position in the text, unfortunately giving the impression that the last sentence means the opposite of what I said on that page. As a guess, this type of confusion could easily be due to selections made by someone not very familiar with the concepts discussed or with not much time available]
From this it is is clear NASA's Mars sample return PEIS team were unaware of any issues with their cites for the Mars meteorite finding. Also my attempt to alert them to the issue didn't succeed.
It would be inconceivable for an expert familiar with the planetary protection literature for a Mars sample return to make such a mistake as this. This story of NASA’s Mars Sample Return team missing significant details when it read documents prepared by planetary protection experts may remind readers of another NASA mishap, with the Mars Climate Orbiter.
In the case of the Mars Climate Orbiter, the navigation team at JPL used “English units” (pound seconds) to prepare data to export to a file which it transmitted to the spacecraft software for some of its operations that couldn’t be handled by the onboard computer on its own (rare occasions when it needs to use thrusters to supplement the reaction wheels that normally keep the spacecraft orientated correctly because of limitations on how fast the wheels can spin). However, it read this data in metric units (newton seconds).
However, it read this data in metric units (newton seconds). This miscommunication between the navigation team on the ground and the spacecraft’s onboard software led to the Mars Climate Orbiter occasionally thrusting 4.45 times more than it should when it rendezvoused with Mars, and instead of orbiting Mars, the spacecraft crashed into the planet (Stephenson et al., 1999. Mars Climate Orbiter Mishap Investigation Board Phase I Report November 10, 1999 : 16 - 17).
This highlights the need for NASA to have extra processes such as some process of peer review of the document to check its cites match the statements they are cited to, to ensure scientific integrity.
This is why I raised the issue of need for peer review of its novel conclusions in one of my public comments,. NASA's team are unaware of this issue just responding that it is not a NEPA requirement to have peer review. That is correct. but it is a NEPA requirement to ensure scientific integrity and one way to ensure that when the conclusions are novel is through peer review. This book covers this topic below in:
All this fails several central legal requirements in the USA on preparers of an Environmental Impact Statement.
- preparers MUST include members with the appropriate disciplines which in this case would certainly include
- familiarity with the planetary protection literature for a Mars sample return mission would be a central requirement for an environmental statement about how to protect Earth during a Mars sample return mission.
The disciplines of the preparers shall be appropriate to the scope and issues identified in the scoping process
§ 1502.6
See Supplementary information under: Fails NEPA requirement to select preparers with disciplines appropriate to the Impact Statement's scope and issues
This also fails another NEPA requirement
- the agency MUST identify opposing views
- in this case the opposing view that the Mars meteorite finding is invalid :
At appropriate points in the final statement, the agency shall discuss any responsible opposing view that was not adequately discussed in the draft statement and shall indicate the agency's response to the issues raised.
§ 1502.9
As we saw, the PEIS doesn't even mention the opposing view to its Mars meteorite finding that is presented in BOTH of the National Academy of Sciences cites NASA uses to support its finding.
See Supplementary information under: Fails NEPA requirement to identify opposing views to its main findings such as the Mars meteorite finding
It also fails the legal requirement on agencies
- Agencies MUST ensure scientific integrity.
- to use a cite to support a sentence that comes to the opposite conclusion without alerting the reader is a basic failing of scientific integrity, and one of the main reasons for peer review is to catch such mistakes
"Agencies shall ensure the professional integrity, including scientific integrity, of the discussions and analyses in environmental documents"
§ 1502.23
For the other three findings skip to:
- NASA's uninhabitable Mars finding rebutted by its own cite, and by NASA's own plans to test for life in its samples
- NASA's Biological Safety Report's finding of near zero risk that martian life can harm us rebutted by soil pathogens like tetanus and aspergillus fumigatus not adapted to any terrestrial host
- NASA's Biological Safety Report's finding that any martian microbe would not be viable on Earth rebutted by the only plausible Mars analogue in its cite: Planococcus Halocryophilus
However first we will look at some of the subtleties in the meteorite argument that are often missed and the importance of looking at worst case rather than best case scenarios for life from Mars on meteorites to protect Earth adequately on the basis of our current level of understanding of Mars.
Some of the many subtleties to the Mars meteorite argument that make it far harder for extant life on Mars to get to Earth
Let's look at the meteorite argument more closely, as there are many subtleties to this argument, easily missed by those unfamiliar with the literature on the topic.
First, all the martian rocks that arrive on Earth today from Mars left Mars at least hundreds of thousands of years ago. The most recent rocks likely left Mars half a million years before the first modern humans evolved, if we go by our best estimate by the crater counts (this method counts how many small craters have formed per square kilometer since some past event on Mars which works because the influx of small meteorites is reasonably steady). These meteorites also came from several meters below the surface. Modeling suggests they come from deep below the surface, at least 50 meters below the surface. At those depths most of Mars is likely uninhabitable by terrestrial life and probably by martian life, except possibly in caves or geological hot spots.
- The most recent meteorites arriving from Mars today came from the Zunil crater impact somewhere around 700,000 years ago by direct crater count (Hartmann et al., 2010, Do young martian ray craters have ages consistent with the crater count system? : 626) (Clark, 2002, Martian meteorites do not eliminate the need for back contamination precautions on sample return missions : 1594)
- All our Mars meteorites come from rocks from at least 3 meters below the surface by the low levels of radioisotopes produced by cosmic radiation (Eugster et al., 2002, Ejection ages from krypton‐81‐krypton‐83 dating and pre‐atmospheric sizes of martian meteorites : 1355). Impact modeling may suggest a depth of 50 to 100 meters below the surface for a typical impactor half a kilometer in diameter that makes a crater 10 kilometers in diameter (Nyquist et al., 2001, Ages and geologic histories of martian meteorites : 152). There’s other confirmatory evidence that they come from at least 1 meter below the surface (Elliott et al., 2022, The role of target strength on the ejection of martian meteorites : 3) because they don’t show any sign of ionizing radiation from the sky on one side of the rock.
- In a scenario with present day life in the surface dirt of Jezero crater, there may be many species that couldn’t get into rocks meters below the surface even if the subsurface is habitable. Microbes that can live inside rocks are called endoliths. Many terrestrial microbes can't live in rocks. Of those that can, many need to live near the surface of the rock with access to sunlight.
- Life on Mars may be restricted to rare oases unlikely to be sampled by a meteorite impact (Clark, 2002, Martian meteorites do not eliminate the need for back contamination precautions on sample return missions : 1599)
- There are many proposed microhabitats native martian life could inhabit in the top few cms
that don't depend on geothermal heat also life from distant oases could be brought to Jezero crater in dust storms
- r see: Life in the uninhabitable - the Space Study Board's thought-provoking review of SR-SAG2 commissioned by NASA and ESA a year later
So, not only do we not have samples of the surface dust, dirt, and salts in Jezero crater. Even the surface rocks at Jezero crater sampled by Perseverance couldn't become meteorites.
Text on graphic: The last meteorites to leave Mars for Earth
- left Zunil crater 700,000 years ago (approx)
- came from at least 3 meters below the surface
- modelling suggests at most ~100 meters.depth
Distance ~5,000 km from Zunil crater to Jezero crater.
In scenarios with present day martian life:
- most species in Jezero crater probably can't reach Zunil crater
(perhaps biofilms in ultracold brines or in micropores in gypsum)
- most terrestrial microbes can't survive sudden ejection at kms / sec or vacuum
- the surface dust, salts and dirt can't physically become a meteorite
- most species in surface layers probably can't get into deep rocks (e.g. photosynthetic)NASA’s iMOST team: “We cannot predict with any accuracy life's form and characteristics, whether it would be viable …, or whether it shares a common ancestor with life on Earth.”
Quote from: (Beaty et al., 2017. iMOST : 88)
Zunil crater, Jezero crater and Elysium Mons labeled
Background map: Google Mars doesn't seem to have an option to share this exact scene - but this is a zoom in on Jezero crater in Google Mars and Zunil crater in Google Mars
Distance between the two locations measured with (NASA, 2023. New Interactive Mosaic Uses NASA Imagery to Show Mars in Vivid Detail)
Clark makes several of these points in his rebuttal of Zubrin's Mars meteorite argument, and also makes an additional point. All the martian meteorites found so far have been dense strong and brittle igneous rocks. These are not likely rocks for life on Mars below the surface. Extant life would be more likely in rocks from warm wet environments, and we have no examples of rocks from such environments in our meteorite collections.
All meteorites identified to date as martian in origin have been dense, highly competent [i.e. strong and brittle], igneous rocks. These are unlikely sources of biological organisms, especially in large concentrations. Much more likely to contain significant quantities of viable organisms would be samples from sedimentary or hydrothermal (wet, warm) environments, materials which probably cannot be ejected without destruction from the surface of Mars
(Clark, 2002, Martian meteorites do not eliminate the need for back contamination precautions on sample return missions : 1599)
Mars might have warm wet environments below the surface, such as hydrothermal vents or sulfur caves, but if so they must be rare and we have no examples of rocks from them in our meteorite collections. See below:
The best case scenario for planetary protection here is for microbes like b. subtilis that may be able to transfer from Mars to Earth (Cockell, 2008, The Interplanetary Exchange of Photosynthesis : 5) (page 5 of the manuscript). But what matters for invasive species are the ones that can’t get here. For instance most photosynthetic life can’t survive the impact shock (Cockell, 2008, The Interplanetary Exchange of Photosynthesis).
Mileikowsky et al. who first established the potential for very hardy microbes like b. subtilis to travel from Mars to Earth in his paper from 2000, say in the same paper that most terrestrial microorganisms known wouldn’t be able to travel through space:
Whereas this harsh environment sets a definite barrier for most microorganisms known, some have developed survival strategies, by transforming into a dry state, the so-called anhydrobiosis … or by producing spores, the dormant state of certain bacteria
(Mileikowsky et al., 2000, Natural transfer of viable microbes in space: 1. From Mars to Earth and Earth to Mars : 392).
If b. subtilis is like Canada geese in a popular analogy for martian microbes, other scenarios for martian microbes may be like Starlings, which can be invasive because they can't cross the Atlantic
A popular Mars colonization advocate uses the colourful analogy that our use of planetary protection officers rules to protect Earth's biosphere is like a policy to search cars to prevent smuggling canada geese across the border from Canada to the USA.
If microbes of any type live in martian surface materials, they don’t need our spacecraft to get here. They have plenty of their own. If the Red Death could come to Earth, it already has. That means that planetary protection rules seeking to impose strict controls on accidental microbial transport by spacecraft make about as much sense as ordering the border patrol to search all entering cars in order to block the importation of Canada geese.
(Zubrin et al., 2022, How to Search for Life on Mars)
We can use his bird metaphor, which many space colonization enthusiasts will be familiar with, to help explain why it is necessary to be careful about microbes from Mars.
Also although Canadian geese can rarely cross the Atlantic it is usually as a solitary bird in a mixed flock with other geese such as barnacle geese. Europe isn't on their migration route and they never set up a population here until deliberate introductions. They were introduced for the first time 300 years ago in 1665 to add to Charles II's wildfowl collection in St James' park which had a stable population of non migratory birds, less than 4,000 until 1950 when several hundred were moved to other parts of the UK which is thought to have set up the conditions for a population explosion. (Allan et al., 1995, The biology of canada geese Branta canadensis in relation to the management of feral populations). They are able to ross the Atlantic on rare occasions in both directions, for instance a bird ringed in Maryland in 1992 and seen in Wales in 1992 and shot dead in Wales in 1993. (BTO, 2007, Summary of all Recoveries for Canada Goose (Branta canadensis) : record 83831004). Canada geese are seen as an invasive species in Europe and there are some measures taken to try to control the numbers.
The Canada geese may be a good analogy for the possibility that a viable martian microbe might reach Earth on a meteorite but land in a location where it is unable to establish a population, or to establish a small population that is unable to spread and eventually dies out.
We'll use the barn swallow is a better analogy for the hypothesis of a species as hardy as b. subtilis able to establish a population on Earth. There is indeed no concern about barn swallows as an invasive species in the Americas, because they can already fly across the Atlantic.
What matters for planetary protection are the worst case scenarios like the starlings which can't fly so far and can never get here. These cause $1 billion of agricultural damage in the USA every year and the only reason this happens is because of the deliberate introduction of starlings to the United States by colonists from Europe.
Starling damage reported to the USDA’s Wildlife Services program averages less than $2 million per year, but this is a fraction of all starling damage. Agricultural damage alone is estimated currently at $1 billion per year. Other damage, such as costs for cleaning and maintaining city centers near roosts, veterinary care and loss of production at CAFOs, and public health care, are unknown. A complete inventory of all economic damage likely would show that the starling is the most economically harmful bird species in the United States
(Homan et al., 2017. European Starlings : 16).
Text on graphic: Some microbes may be able to get from Mars to Earth – what matters for invasive species are the ones that can’t.
Barn swallow - can cross Atlantic
Starling - invasive species in the Americas
Didymosphenia geminatum invasive diatom in Great Lakes and New Zealand, can’t even cross oceans
Microbes can be invasive too. One clear example of an invasive terrestrial diatom is the freshwater diatom "Didymo" (Didymosphenia geminatum) which causes many problems in New Zealand (Spaulding et al., 2010. Diatoms as non-native species).
The COSPAR Sample Safety Assessment Framework, say that it's not feasible to predict harmful or harmless consequences if life is detected:
Unfortunately, we have only a limited ability to predict the effects of terrestrial invasive species, emerging pathogens, and uncultivated microbes on Earths' ecosystems and environments. This is true even for cultured and fully genome-sequenced terrestrial organisms and more so for potential extraterrestrial life. Thus, conducting a comprehensive sample safety assessment with the required rigor to predict harmful or harmless consequences of potential martian life for Earth is currently not feasible.
(Kminek et al, 2022, COSPAR Sample Safety Assessment Framework (SSAF))
This is something that needs to be expanded on. COSPAR doesn't seem to have incorporated the expertise of scientists who study invasive species.
Advances in planetary biosecurity must keep up with the pace of these potential risks, and this goal could be facilitated by collaborations between researchers in invasion science and astrobiology. To our knowledge, invasion biologists have not been involved in development of the COSPAR policy on planetary protection, in spite of obvious conceptual parallels and decades of empirically derived insights that could be applied.
(Ricciardi et al., 2021, Planetary Biosecurity: Applying Invasion Science to Prevent Biological Contamination from Space Travel : 5)
It would help to fill this gap if we wish to do a future thorough study of potential effects of microbes returned from Mars.
Ricciardi et al. in their paper applying invasion science to planetary protection warn that the worst case effects of introduction of invasive species into isolated island systems can often be ecologically devastating.
Insular systems are most vulnerable to invasion. A major lesson from invasion science is that ecosystems that have evolved in isolation are exceptionally vulnerable to disruption by introduced alien organisms .... For insular systems (e.g., islands, lakes, remote habitats), which typically contain endemic species and unique phylogenetic lineages, the consequences of invasion have often been catastrophic, causing cascading ecological effects and extinctions ....
(Ricciardi et al., 2021, Planetary Biosecurity: Applying Invasion Science to Prevent Biological Contamination from Space Travel : 2)
Astrobiologists say we also need to be prepared to find unfamiliar life on Mars that has no common ancestor with terrestrial life. That includes the iMOST team NASA assembled to advise them on the science experiments for the samples from Jezero crater.
“We cannot predict with any accuracy life's form and characteristics, whether it would be viable …, or whether it shares a common ancestor with life on Earth.”
(Beaty et al., 2017. iMOST : 88)
In short we need to look at worst case scenarios rather than best case scenarios. When we do that, there are issues here that NASA haven't addressed in its programmatic environmental impact statement. This is due to its reliance on a plausible but invalid argument that they found convincing, that any life on Mars has got here already. They missed the rebuttals of this argument due to the lack of anyone on their team familiar with the literature on the topic which we have covered here and due to lack of familiarity with the contents of their own cites on the topic
The Mars meteorite argument is the most important of four arguments NASA relies on to reach the conclusion that the environmental effects of the release of unsterilized martian materials would not be significant and any health impacts negligible.
NASA's uninhabitable Mars finding rebutted by its own cite, and by NASA's own plans to test for life in its samples
With NASA's Mars meteorite finding rebutted, NASA's finding that the surface of Mars has been uninhabitable for millions of years becomes their main support for harmlessness of the samples. But NASA's own studies rebut this argument.
This is what NASA's final PEIS says, that it is a consensus that the surface of Mars has been uninhabitable for millions of years.
Existing credible evidence suggests that conditions on Mars have not been amenable to supporting life as we know it for millions of years… National Research Council 2022).
(NASA, 2023. Mars Sample Return final PEIS : 1–6)
This expert opinion is so far from a consensus that NASA's own most recent cite for this sentence from the National Research Council from 2022 is about searches on Mars for localized habitable regions in a seemingly uninhabitable world.
“The exploration of Venus ... and Mars (e.g., by Curiosity and Perseverance) will help establish whether localised habitable regions currently exist within these seemingly uninhabitable worlds”.
Once habitable environments are identified, the search for evidence of life represents the logical next step and the greatest challenge.
(National Research Council, 2022. Origins, Worlds, and Life : 378)
[If the page is obscured by a splash screen, click on the X to go straight to page 378].
These localized habitable regions haven't yet been found, but that is because we haven't looked for them yet. Apart from the Viking landers in 1979s all our missions to Mars so far have had past habitability as their main focus as we'll see in the next section.
When I raised this point, that their cite was about a search for whether localised habitable regions currently exist on a seemingly uninhabitable Mars, NASA's reply was to assure me that Mars is uninhabitable for life as we know it and that the researchers are searching for habitats for life "not as we know it". This is how they put it:
Robert Walker: Draft EIS says (MISTAKENLY) existing credible evidence suggests Mars hasn’t been habitable for life as we know it for millions of years - their cite says that we need to search for current habitats in a seemingly uninhabitable Mars
NASA: This comment confuses two concepts: “life as we know it,” and potentially extant life on Mars that is different than life as we know it.
(NASA, 2023. Mars Sample Return FINAL PEIS : B-81)
However it is easy to confirm the researchers in the study they cited are talking about "life as we know it". For instance it lists Mars Life Explorer (MLE) as the most important next mission after Perseverance, a mission to search for present day life on Mars. They say that it's purpose is to detect native Martian life before any potential habitats are contaminated by terrestrial life brought to Mars by humans.
The committee ranked MLE as the highest priority medium-class mission for the MEP. “Are we alone?” is one of the most profound questions that can be addressed by solar system exploration (Q11).
Mars has subsequently undergone profound climate change (Q6), and key questions are whether any habitable environments persist to the present and whether they are inhabited. The focus of Mars Life Explorer is to seek extant life and assess modern habitability.7 The notional mission concept examines Mars’s lowest latitude ice deposits that preserve a record of recent climate change and may provide a recent habitat for life. MLE would land and drill into the ice to characterize and quantify organics, trace gases, and isotopes at a fidelity suitable for biosignature detection. It would also assess ice habitability and the question of modern liquid water via analysis of elemental chemistry, salts, conductivity, and ice thermophysical properties. Long-term atmospheric measurements over a martian year would determine the current stability versus instability of the ice deposits.
Recommendation: Subsequent to the peak-spending phase of MSR, the next priority medium-class mission for MEP should be Mars Life Explorer.
(National Research Council, 2022. Origins, Worlds, and Life : 569)
Then the Mars Life Explorer mission paper says that we must search for modern terrestrial life on Mars before humans get there. I
The extent to which human microbiota may forward contaminate Mars is under study (Fairén et al. (2017) and references therein). To be confident in the identification of modern Martian life, we must know that the life detected did not originate from terrestrial forward contamination.
Therefore, in a most conservative approach, we must search for modern life prior to the arrival of humans on Mars. In addition, any detection of extant life may modify approaches to human exploration, including the choice to not send humans to Mars (Persson 2019).
(Williams, 2021. Mars Life Explorer : 1-3)
This issue that human missions might introduce terrestrial life to Mars irreversibly and even globally before we get a chance to study any native martian life that may live there is one of the main topics of discussion in the contemporary planetary protection literature about Mars.
The closest expert opinion to NASA's view that the surface of Mars is currently uninhabitable in the current literature that I found is from the NASA astrobiologist Chris McKay.We will look at his views in this book in detail below:
To see that the search is for potential habitability of the surface of Mars for life "as we know it", we can also go to NASA's own iMOST team, which it assembled for advice on science experiments with the Perseverance samples. iMOST recommended tests for life in the very samples they plan to return from Jezero crater:
Identify and measure evidence for cellular growth, metabolism, and respiration
(Beaty et al., 2017. iMOST : table 2.20)
They plan to:
- test these very samples for metabolism and respiration (Beaty et al., 2017. iMOST : 92).
- experiment to see if they can get anything to grow from them (Beaty et al., 2017. iMOST : 93).
They say we should use our knowledge about microorganisms under similar conditions on Earth as a basis for the experimental design, as well as chemical analysis of the samples and measurements of the Mars surface conditions.
Success in cultivating organisms from martian samples would be the ultimate proof of extant life. On Earth we can only culture a few percent of all environmental microorganisms because it is difficult to predict and reproduce conditions amenable to growth. We expect that martian organisms would be no less recalcitrant. Therefore, it is critical that growth experiments be conducted under conditions present at the sample site.
... In the future, experimental design should be based upon (1) chemical analyses of returned samples, (2) Mars surface conditions (temperature, pressure, and atmosphere), and (3) our knowledge about the physiology and metabolism of microorganisms under similar conditions on Earth
(Beaty et al., 2017. iMOST : 93)
The iMOST team said even related life on Mars is likely to have diverged significantly from the tree of life of terrestrial organisms unless it was recently seeded with Earth life.
Unless Mars was recently seeded with Earth life, it should be phylogenetically different from modern life, either having diverged from the tree of life prior to the most recent common ancestor to life on Earth or showing a deep-branching evolutionary relationship
(Beaty et al., 2017, iMOST : 93).
NASA's iMOST team goes on to say we can't predict whether any life on Mars shares a common ancestor with life on Earth.
“We cannot predict with any accuracy … or whether it shares a common ancestor with life on Earth.”
(Beaty et al., 2017, iMOST : 88)
So yes, NASA's Mars sample return team is correct to say that Perseverance could return life "not as we know it". However NASA's iMOST team also says it is possible Perseverance samples could return viable present day life as we know it, distant cousins of terrestrial life and our search is for microhabitats and localized habitable regions for life as we know it for the simple reason that we know that life is possible in such habitats and we don't know for sure where else to look for exotic life.
Indeed, as we'll see, the main focus for planetary protection especially is on microhabitats for life as we know it because we don't have clear limits for characteristics to look for, for habitats for life not as we know it (Rummel et al., 2014. A new analysis of Mars “special regions”: findings of the second MEPAG Special Regions Science Analysis Group (SR-SAG2) : 888).
So NASA's PEIS team was just confused again. It's impossible to know how theo confusion arose as they give no page number cites to the study for where they think it said that it is about searching only for "potentially extant life on Mars that is different than life as we know it. ". (NASA, 2023. Mars Sample Return FINAL PEIS : B-81)
In any case it doesn't matter if it is life as we know it or not life as we know it, we still need to protect Earth.
For life generally, we can look at NASA's Mars science goals as set out by its MEPAG team. The second half of Goal 1 is to determine if Mars still supports life, it doesn't say whether this is life as we know it or a novel biology.
Goal 1: determine if Mars ever supported, or still supports life
(Banfield et al., 2020. Mars science goals, objectives, investigations, and priorities: 2020 version: 9).
It is also objective 2.3 for NASA's samples which they plan to return from Jezero crater:
Proposed objectives:
2 Life: 2.3 Modern biosignatures: Assess the possibility that any lifeforms detected are still alive or were recently alive(Velbel et al., 2022. Planning implications related to sterilization-sensitive science investigations associated with Mars Sample Return (MSR) : Table 1)
In short, NASA's own argument is rebutted by its cites and by the recommendations of its own iMost team.
A more accurate statement would be
The probability of discovering extant life is generally considered to be low, but there is enough of a chance of life in the Perseverance samples for NASA to test for living organisms in it (iMOST : page 86)
So how did NASA's team make such a major mistake on such an important topic for the PEIS?
It could be a simple lack of communication between the engineering team and the life detection scientists. Such things happen, like the mix-up that led to the loss of the Mars Climate Orbiter which crashed into Mars in 1999 (Stephenson et al., 1999. Mars Climate Orbiter Mishap Investigation Board Phase I Report November 10, 1999).
- Some scientists in JPL used the English units of pounds, feet and seconds and others used the metric units of kilograms, meters and seconds.
- A small file called AMD was saved in English units when its specification said it should have been saved in metric units.
- Modelers assumed the file used the correct units, and that lead to the crash
(Stephenson et al., 1999. Mars Climate Orbiter Mishap Investigation Board Phase I Report November 10, 1999)
Or maybe it is optimism about their respective goals.
- For scientists devising experiments to look for life, the most interesting outcome by far is present-day life. Optimistic iMOST authors will naturally recommend NASA to test for present-day life even if it may be unlikely, because it would be such a major discovery.
- For engineers and mission planners preparing protocols to protect Earth, the less the risk, the lower the cost of the mission. So, it is natural for optimistic planners to find it more plausible that Mars has been uninhabitable for millions of years, as this is the best outcome from their point of view, likely reduce the cost of the mission and make mission planning and engineering simpler.
Whatever the cause, it is another example that shows NASA failed the NEPA requirement to include experts of the appropriate disciplines amongst the preparers of the PEIS. See Supplementary information under:
Why NASA still looks for present day martian life: our only previous attempt to look got confused, the main focus has been on past habitability, and Mars could have barely habitable microbial oases in an almost lifeless planet
It's clear from the last section that NASA is still looking for present day life on Mars. But one might wonder why they do.
Wouldn't we have found life on Mars by now if it was there, after so many decades of exploration with our landers and rovers? We find microbes almost anywhere we look on Earth, even in a scoop of dirt from the Sahara desert, so long as we use equipment sensitive enough to detect life.
There are two reasons we haven't resolved this yet:
- first we have never sent instruments capable of spotting life, except for the Viking landers in the 1970s, which got confused by the unusual surface chemistry,
- second, on such a dry planet as Mars, life might be hard to find. Earth has some ultra-dry spots in remote deserts without life. Mars might be the other way around, almost all lifeless, with only a few small microbial oases that may be hard to find - perhaps supplemented by occasional viable life spores or other dormant life in the dust.
In more detail:
1. We have only ever sent life detection instruments to Mars once, in the 1970s on the two Viking landers. These produced confused results.
The Viking labeled release experiment fed organics labeled with radioactive carbon to the dirt then tested to see if any microbes gave off the gases, an exquisitely sensitive experiment even with 1970s technology. The experiment detected labeled gases, but a less sensitive life detection experiment didn't detect anything and the third experiment, which did chemical analysis with an oven, detected chlorinated organics which were dismissed as a contaminant at the time. Given the evidence of a very dry desert on Mars, most scientists concluded that the labeled release experiment must be somehow mistaken.
Later, Phoenix discovered perchlorates, which are rare in most terrestrial deserts. However, these didn't resolve the issue as they cast doubt on both the life and non-life interpretation of the Viking experiments. The perchlorates could have produced the chlorinated organics when heated with organics in an oven, making them less conclusive as evidence against life on Mars. They could also produce labeled gases when they react with organics which made the labeled release experiment less conclusive.
That is it by way of in situ life detection on Mars. We have had no follow up life detection experiments to check the Viking results.
NASA's iMost team proposes doing a more sensitive version of the Viking experiments with the returned samples. However as a test for respiration, it will only detect present day life on Mars if it survives the journey back to Earth.
In one experiment Viking 1 found that the sample of dirt didn't produce the same evolved gases after being kept in darkness at a temperature of at least 10°C for 141 sols, which was then confirmed by repeating the same experiment deliberately with Viking 2 after storage for 84 sols (Levin et al., 2016, The case for extant life on Mars and its possible detection by the Viking labeled release experiment). Whether the active ingredient is chemical or life, it seems we may need to be careful about the conditions in which we store it on the journey back to have a decent chance of replicating the Viking results.
However if the samples do contain still viable life, including dormant life that we are able to revive, then these tests may find it. It may also tell us more about surface chemistry.
This book suggests we have a greater chance to resolve this if we can return a scoop of soil collected in a 100% clean sterile container.
For details of the iMost proposed experiments see also this book's discussion of how their refinement of the Viking experiments could be done telerobotically in a small satellite above GEO:
2. On the potential for microbial oases in an almost uninhabitable planet, gradually over the decades since the 1970s we have found life able to live in more and more extreme conditions on Earth including ultra cold deserts, with almost no access to water and exposed to high levels of ultraviolet light and reactive chemicals. We have also found that in Mars analogue deserts, the ultra dry deserts most like those on Mars, that life isn't found everywhere. We find some rocks that have life and others that don't, apparently identical, with the habitability varying due to minute variations of the humidity.
This leads to the idea that perhaps on Mars we might find the reverse, a surface that is uninhabitable and uninhabited almost everywhere but with small microbial oases that microbes could inhabit in small quantities. See below:
The next change is the discovery of the Phoenix leg droplets in 2009, which then led to many other new ideas about possibilities for microbial oases on Mars. It also led to Mars simulation experiments able to produce conditions where terrestrial life can survive and even grow slowly in simulations of Mars surface conditions possibly in some places on Mars. I cover this research in the section:
There are now numerous proposals for microhabitats on Mars including some that may be possible in Jezero crater itself. See below, this and following sections:
Astrobiologists want to send our rovers to search for these microhabitats in regions on Mars with a high level of potential for present day life. But Perseverance wasn't adequately sterilized to do that. To sterilize it sufficiently would add 10% to the cost of the mission. NASA is required to be very careful about costs.
This book identifies this funding issue as one of the main restraints on astrobiological study of Mars. We look at this in the sections below:
- Need to avoid a trade-off between planetary protection and NASA's obligations to minimize costs
- An independent body to safeguard Earth and Mars: avoiding perverse incentives to reduce safety by ensuring planetary protection costs don't come from NASA's budget
- Suggestion to resolve this debate with more, rather than less ambition, making a case to Congress for extra funding for 100% sterile rovers, integrated closely with human missions with the aim to find out if it is safe for humans to land on Mars
So Perseverance has been sent to a region of Mars considered unlikely to have present day life, but not impossible. No mission has had in situ life detection since the Viking missions in the 1970s. So, if there is present day life in Jezero crater, we don't know where to look for it because we've never searched for it on Mars in situ since Viking. It is the same situation for past life. Our recognizable traces of distant past life from three billion years ago are often patchy and hard to find or confirm even on the far more habitable Earth. Astrobiologists say it is likely to be as hard to find past life on Mars, though the reasons for this differ in detail. Without in situ life detection, Perseverance doesn't know where to look.
Perseverance will greatly advance geology and tell us more about past conditions in Jezero crater, which we already know was habitable over 3 billion years ago. It will return a couple of samples of the soil which may help us to get more insight into what Viking found. But the chance of it resolving central questions in astrobiology may be low.
With this background the chance of Perseverance returning viable life from Mars still seems very low. However it is not zero. It is high enough to explain why NASA consider it to be worth the effort to test to see if there is life in the samples, as we saw.
However, this means we do have to consider the need to protect Earth's biosphere for this mission, and also as precedent for future missions.
Life in the uninhabitable - the Space Study Board's thought-provoking review of SR-SAG2 commissioned by NASA and ESA a year later
Though the chance of life in Jezero crater is likely low it may not be as low as NASA suggests. NASA tell us that there is a consensus that the martian surface is too inhospitable for life to survive there today, particularly in the shallow subsurface in Jezero crater. Their source SR-SAG2 is the controversial one.
Consensus opinion within the astrobiology scientific community supports a conclusion that the martian surface is too inhospitable for life to survive there today, particularly at the location and shallow depth (6.4 centimeters [2.5 inches]) being sampled by the Perseverance rover in Jezero Crater, which was chosen as the sampling area because it could have had the right conditions to support life in the ancient past, billions of years ago (Rummel et al. 2014, Grant et al. 2018).
[Rummel et al., 2014 is often referred to as SR-SAG2]
(NASA, 2023. Mars Sample Return final PEIS : S-4)
In this passage, Grant et al is uncontroversial, it just explains why one of the proposed landing sites for Perseverance was excluded, Vastitas Borealis. because of a risk of near-surface water ice (Grant et al.,, 2011. The science process for selecting the landing site for the 2011 Mars Science Laboratory). For a rover partially sterilized like Perseverance there has to be a very low risk of terrestrial life to spread on Mars. The partial sterilization of Perseverance then reduces the risk further to the point where it is considered tolerable for planetary protection. Because of the potential of water ice in Vastitas Borealis. near to the surface, even the partially sterilized Perseverance isn't seen as clean enough to send there, in case it might introduce terrestrial life to Vastitas Borealis. This does NOT mean that it is impossible for terrestrial life to spread in Jezero crater, just that the risk is so very low that Perseverance is considered to be adequately sterilized enough to prevent it. For details see below:
The controversial cite here is Rummel et al (SR-SAG2). This is the one that got a thought-provoking review a year later from the Space Studies Board / National Academy of Sciences, commissioned by NASA and ESA (SSB, 2015. Review of the MEPAG report on Mars special regions). This found many ways that there could be small inhabited oases for life which we can't spot from orbit, so that the planet seems to be uninhabitable. We don't know these oases exist but we have many knowledge gaps that could permit them to exist depending how they are resolved. It is because of these knowledge gaps that the NRC in 2022 described Mars as only "seemingly uninhabitable" in the quote we used:
“The exploration of Venus ... and Mars (e.g., by Curiosity and Perseverance) will help establish whether localised habitable regions currently exist within these seemingly uninhabitable worlds”.
Once habitable environments are identified, the search for evidence of life represents the logical next step and the greatest challenge.
(National Research Council, 2022. Origins, Worlds, and Life : 378)
NASA's Rummel et al. cite SR-SAG2 describes what WAS the consensus at the turn of the century, or a near consensus (a few such as Gill Levin continued to think there could be microhabitats on the surface of Mars throughout). This remained the consensus through to most of the first decade of this century. However, by 2014 when SR-SAG2 was written expert opinion was already diversifying which is why both NASA and ESA took the very unusual step of calling for a review of SR-SAG2.
This book looks at the circumstances that led to the review below:
The SSB review identified several major knowledge gaps of special relevance to the potential for terrestrial life to grow in locations on Mars like Jezero crater.
This book looks at five of those knowledge gaps of special interest to Jezero crater.
The 2015 SSB Review of SR-SAG2 said that
- Numerous ideas for microenvironments, such as a micropore in a salt deposit or a microcave or a thin briny seep below the soil, which is small and hard to spot but still a vast region for a microbe to explore.
See below: though SR-SAG2 considered microenvironments on Mars, it only briefly considered the implications of our lack of knowledge of them - Microbes in terrestrial deserts normally live in multi-species communities called biofilms. These communities build themselves miniature "homes" or "cloches" or "polytunnels" by extruding bioplastics (called EPS) which the microbes cover themselves with to keep out the harsh conditions, and make the interior more habitable. Also they function rather like mobile homes or caravans or parachutes for the microbes. Fragments of biofilms can break off and spread like dandelion seeds in the wind and settle in another part of the desert. Then as soon as the biofilm touches down, the microbes have a ready built home to live in right away.
See below: microbes are likely to need to develop biofilms, like organic plastic "houses" or cloches, before they can grow in otherwise uninhabitable ecological niches on Mars - These "mobile homes" can be blown hundreds of kilometers in the dust. Though the surface conditions are very hostile to life in direct sunlight because of the uV from the sun. But in these biofilm "mobile homes" they can withstand it for hundreds of kilometers or more, especially if they travel at night (winds continue to blow at night) or during the thick dust storms that can turn day to night SR-SAG2 didn’t adequately discuss the potential for life to be transported in the dust in the atmosphere (e.g. dust storms)
- It is impossible to see a micropore in a salt deposit, or a microcave or fracture or a thin seep of habitable salty water beneath the dust from orbit.
See below: See below:any maps from orbit can only represent incomplete knowledge about habitability that will certainly be changed - Mars is likely to have the full range of caves that Earth has as well as some other types that can only form on Mars (e.g. from sublimation of dry ice). As on Earth they could extend for hundreds of kilometers below the surface, could have warm pools in them heated from below, and in the low martian gravity they can be far larger than is possible for any terrestrial cave. The temperature in near surface caves even not geologically heated would be around -25 C which is warm enough to make it possible that cold tolerant life could be able to grow, very slowly, in salty brines. The entrances to most caves would be impossible to see from orbit or even from close up on the surface and Mars has occasional slumps of the sand which could reveal a previously buried cave entrance.
See below: there may be more potential for life in caves than suggested in SR-SAG2
We will look at them in detail later in the book in those linked sections.
We also look at some of the microhabitats from the literature suggested by experts on Mars surface conditions that could exploit the conditions that these knowledge gaps may permit.
- Potential for ultra low concentrations of life from microbial oases in Jezero crater itself
- Caves in Mars habitability studies: a major focus and the difficulty of detecting them from orbit or using rovers on the surface
- NASA discovered potential for current habitats for terrestrial life in Gale crater AFTER Curiosity’s landing: RSLs, most are likely dry dominated but some still could have a wet dominated mechanism
- Potential for distant microbial oases as sources of ultra low concentrations of viable spores in dust storms
- Potential for distant Mars habitat common in Antarctica: Fresh water drilled by sun warmed dust and prevented from evaporation by an ice lid
- Ice boulders thrown onto the surface in 2021 from a formerly habitable site on the flanks of Olympus Mons
[This last section is speculation combining the ideas of habitable ice with the discovery of these recently ejected ice boulders that might conceivably contain still viable microbes.]
The details are postponed to later in the book because though there is much of interest in them, at this stage the aim is just to outline the main points.
You might wonder why, with all these knowledge gaps, we still use partial sterilization just as we did before the SSB Review instead of the much higher level of sterilization used for the Viking landers. The reason is that after the SSB review, even with the knowledge gaps interpreted in their most optimistic way for habitability the new picture is still one of an almost entirely uninhabitable Mars. However, these knowledge gaps might permit occasional microbial oases scattered over the surface of Mars, where a few microbes may be able to survive especially in biofilms, with a possibility that some of those oases might even exist in places like Jezero crater.
This is too small of a change to make a significant difference to a partially sterilized rover like Perseverance. That's because the partial sterilization ensures that Perseverance has no biofilms and low levels of single microbes, most of which would die on the way to Mars or on the surface of Mars in the harsh conditions there. It continues to be a consensus opinion of experts that the risk of forward contamination from such a mission is very small even if there are rare oases for microbes in Jezero crater, so the planetary protection classification didn't change.
However these knowledge gaps do make a difference to discussions for the risk of forwards contamination after a human landing, opening up prospects for biofilms to colonize even many drier areas of Mars if there is some prospect for water to get trapped in them, or for hardy spores or biofilms to still be viable after transporting the dust storms.
Indeed these knowledge gaps have made such a difference that one of the main planetary protection discussions in the journals today, spanning numerous papers, is how much science we can do before human missions start an anthropocene on Mars. Some astrobiologists have as their expert opinion that this is precisely what will happen after our first human mission, if we send humans to land on the planet: that it will irreversibly introduce terrestrial life which will then spread globally throughout the planet.
The clearest way to show that there is no longer an expert consensus that Mars is uninhabitable is to look at the expert opinion furthest from the views of NASA's Mars sample return team in the spectrum of current expert opinions in the literature.This is how Alberto Fairén puts it as his expert opinion:
Text on graphic: Alberto Fairén - expert opinion at opposite end of the spectrum from NASA. Already certain of microbial oases for life throughout Mars globally.
What we already know is that the moment that astronauts set foot on Mars, microbial contamination will be inescapable and irreversible.
,,,
The microbial leaks and species invasions could spread far enough to produce a global impact on Mars, eventually creating identifiable sediments.(Fairén, 2019, The Mars Anthropocene)
For an intermediate position between NASA and Alberto Fairén on this topic we can go to NASA's first two planetary protection officers, John Rummel and Cassie Conley. They agree we need to do a dedicated robotic search for life on Mars before humans get there, agreeing with this sentence by Alberto Fairén: ‘‘that we need to resume a dedicated robotic search for life on Mars as soon as possible, before manned missions reach the planet and it becomes too late.’’. They agree that humans would irreversibly contaminate Mars in the forward direction. However, their expert opinion is that if humans land in a site like Jezero crater, the contamination can be localized to near the landing site at least in the near term and that there would remain microhabitats on Mars which life doesn't spread to for a significant period of time (Rummel et al., 2018, Inadvertently Finding Earth Contamination on Mars Should Not Be a Priority for Anyone : 114).
These two groups of experts have debated this topic in a series of many papers from 2017 onwards. They continue to have different expert opinions on the topic, many of which can only be resolved with more data from Mars.
The closest to NASA's view in the current literature that I found is NASA astrobiologist Chris McKay. He sees the potential habitability of the surface as very low and gives as his expert opinion that we can reverse the effects of a human landing if needed (McKay, 2018,. The Search on Mars for a Second Genesis of Life in the Solar System and the Need for Biologically Reversible Exploration : abstract)
We will look at his views in this book in detail below:
These views all survive peer review and so the debate can’t be resolved at present. It depends on how sterilizing the martian surface is for terrestrial life, whether there are surface microhabitats that it can colonize, how far apart they are, if they exist, and whether terrestrial life can be transferred from one to the other, perhaps in biofilms blown in the winds. These are knowledge gap. As we’ll see there is a fair bit of research into the topic with simulation experiments in terrestrial labs, but these experiments are not able to decide the debate. We need experiments we can only do on Mars before we are likely to get a consensus of experts and in the peer reviewed literature.
The PEIS doesn't mention any of these papers. It's another surprising omission suggesting once more a lack of familiarity with the planetary protection literature. This range of expert opinions has obvious relevance in the reverse direction to the (likely still low) potential habitability of Jezero crater for martian life. We look at it below here:
Although the discussion in the literature focuses on forward contamination of Mars by terrestrial life, the same knowledge gaps also change discussions about the potential for native martian life in Jezero crater which is our main focus here.
If Mars does indeed have microbial oases almost everywhere that could be exploited by terrestrial life to lead to an irreversible global martian anthropocene soon after a human landing, this means that it also has conditions where native martian life may occur globally on Mars. The likelihood of martian life at locations such as Jezero crater is still considered to be low, but not as low as previously thought depending on how the knowledge gaps get filled as we find out more, which is why it is reasonable for NASA to consider testing to see if they return viable life from Mars.
We see that at present there is a wide range of expert opinions on the topic consistent with the data, with no sign yet of convergence on a single consensus opinion. This can be resolved but it depends on further in situ research on Mars which hasn't yet been done.
NASA's Biological Safety Report's finding of near zero risk that martian life can harm us rebutted by soil pathogens like tetanus and aspergillus fumigatus not adapted to any terrestrial host
So far we have found rebuttals for NASA's Mars meteorite finding and its finding that the surface of Mars has been uninhabitable for millions of years.
So the main remaining support for the harmlessness of the samples is in their two findings that martian life can't harm us. These findings are
- that there can't be any pathogens of terrestrial higher organisms on Mars, and
- that any martian life can't live on Earth .
In the next few sections we will look at its pathogens finding. For the finding that no life from Mars can live on Earth see below:
NASA' finding of near zero risk of martian pathogens may seem intuitively plausible. Most of us would think first of pathogens adapted to higher organisms, like flu or COVID. If there is multicellular life it is likely to be primitive and not at all similar to terrestrial mammals or even insects. The chance that the fungi, lichens or whatever else Mars has have diseases that could harm humans would seem to be very remote.
However NASA's finding goes against the findings of all the main planetary protection studies on a Mars sample return mission as we saw above.
So what are the issues with this reasoning? What it misses are soil pathogens like tetanus, which most of us give little thought to. We've most of us been vaccinated against tetanus as a child, but it remains a serious disease that kills newborns in weaker economies without the same level of access to vaccines. There are other soil pathogens too, the one that might be most familiar to some non medical readers is Aspergillus fumigatus as it is one of the common causes of asthma and sometimes of more serious diseases. Like tetanus, it is not adapted to any host. One of the best known examples is Legionnaires' disease which is also not adapted to any hosts. Perhaps the most detailed review of this topic in the planetary protection literature so far is the one by Warmflash et al. (Warmflash et al, 2007, Assessing the Biohazard Potential of Putative martian Organisms for Exploration Class Human Space Missions : 14 - 15):
However, NASA's Biological Safety Report somehow missed the planetary protection literature on soil pathogens. It looks at nine examples of infectious human diseases (Ebola, HIV, influenza, Escherichia coli strain 0157:H, Candidiasis yeast, Kaposi's sarcoma, malaria, yellow fever, and schistosomiasis). They find that all nine of their example diseases needed to evolve in an Earth host. They found that they either co-evolved with humans or jumped to humans from birds or mammals, or in the case of malaria, yellow fever and schistosomiasis, evolved in a more complex relationship between humans and snails or mosquitoes (with common ancestor between snails or mosquitoes and humans 600 to 1,200 million years ago).
As we will see in a minute, they may have made a mistake even in this short list. Researchers don't yet know if Shiga's toxin produced by e.coli coli strain 0157:H co-evolved with humans. It may be more plausible that this strain evolved this toxin in biofilms to defend against protozoa which resemble white blood cells and was only accidentally useful for infection of humans.
Anyway, based on these nine examples NAS's report concludes that we have near zero risk from martian microbes. Here is where they make this deduction:
Text on graphic: Leaves out Legionnaires' disease, Tetanus, Coristidia perfringens [food poisoning], Botulism, Ergot disease, Rock inhabiting black fungi like Exophiala jeanselmei MA 2853, Aspergillus fumigatus, etc. None adapted to any higher organism.
“Since any putative martian microorganism would not have experienced long-term evolutionary contact with humans (or other Earth host), the presence of a direct pathogen on Mars is likely to have a near-zero probability.”
(Craven et al., 2021. Biological safety : 6)
The main omission here is that the report doesn't include any attempt at a search to see if there are any diseases of humans that are not adapted to an infectious lifestyle in any terrestrial host. Any such literature search would have quickly turned up many examples such as tetanus, which experts in human diseases would think of immediately, soil pathogens.
This isn't an issue with the individual scientists but with the choice of preparers. it shows there is nobody on the team familiar with
- risk assurance (or they would have focused attention on worst cases and done the literature search to see if they exist)
- the planetary protection literature (as it has covered such examples before)
- public health (any expert, for instance from the CDC, would be familiar with many of these examples such as tetanus which causes thousands of deaths of unvaccinated newborns every year).
This goes against the NEPA requirement:
The disciplines of the preparers shall be appropriate to the scope and issues identified in the scoping process
§ 1502.6
See Supplementary information under: Fails NEPA requirement to select preparers with disciplines appropriate to the Impact Statement's scope and issues
The passage shown in the screenshot from NASA's biological safety report has no cites to the planetary protection literature or to anyone else who has used this argument before.
The only previous occurrence of this argument I know of is in that non peer reviewed op ed by Robert Zubrin, president of the Mars Society, already mentioned (Zubrin, 2000. Contamination From Mars: No Threat) which was immediately rebutted in (Rummel et al., 2000. Opinion: No Threat? No Way : 4 - 7) and in more detail in (Warmflash et al, 2007, Assessing the Biohazard Potential of Putative martian Organisms for Exploration Class Human Space Missions : 14 - 15).
There are many soil toxins and pathogens not adapted to an infectious lifestyle in any organism. Let's look for instance at tetanus.
We can now protect babies with tetanus vaccines, and they are widely available, yet tetanus still kills thousands of newborns every year in weaker economies as cases of neonatal tetanus [neonatal means in the first 28 days of a baby’s life] (WHO, n.d., Tetanus). Tetanus is a common anaerobic (non oxygen using) soil bacteria. The tetanus toxin is made by a plasmid (pE88), a small self-contained circular DNA molecule, which is independently evolved from the main genome of the microbe. The origins of this plasmid are unclear as it seems to be unique to Cloristidium tetani
The origin of pE88 remains unclear. Over 50% of all ORFs on pE88 are unique to C. tetani
(Brüggemann et al.., 2003. The genome sequence of Clostridium tetani, the causative agent of tetanus disease)[Open Reading Frame (ORF) means a section of a strand that can be transcribed by a sign le ribosome before it gets to a stop signal (NIH, 2023, Open Reading Frame) ]
If we go to the planetary protection literature, Warmflash et al looks at various counterexamples (including tetanus)
The examples include (Warmflash et al, 2007, Assessing the Biohazard Potential of Putative martian Organisms for Exploration Class Human Space Missions : 14 - 15):
- Legionnaires' disease
- Tetanus (caused by Clostridia tetani)
- Clostridia perfringens, one of the most common causes of food poisoning
The Clostridia genus of fungi is anaerobic so doesn't need oxygen. Warmflash et al. also look at fungal diseases of crops that can harm humans when they eat them
- Botulism (C. botulinum)
- Ergot disease (C. purpurea)
We now have many terrestrial fungi which do well in Mars simulation chambers. This suggests that there might be a realistic possibility in the other direction that Mars has fungi that would be able to grow on Earth. The rock inhabiting black fungi are especially interesting here. These can adapt to extreme environments, hot and cold and other extremes such as high salinity, acidity, and desiccation, and many have been able to colonize rocks in Mars analogue conditions in Antarctica (Selbmann et al, 2015, Rock black fungi: excellence in the extremes, from the Antarctic to space)
One of these black fungi, Cryomyces antarcti was tested in the BIOMEX experiment simulating a martian atmosphere, exterior to the ISS. At the end of the experiment it was not only still viable but showed only slight damage too fine to see with optical microscopy (Pacelli et al., 2017, BIOMEX experiment: ultrastructural alterations, molecular damage and survival of the fungus Cryomyces antarcticus after the experiment verification tests) These types of fungi have been given many names in the literature including “black yeasts” and “micro-colonial fungi”..
With this background, it makes sense to look carefully at fungi in searches for a worst case soil pathogen from Mars
One of these black fungi is closely related to human pathogens. That’s Exophiala jeanselmei MA 2853, a rock inhabiting black fungus in moderate climates, which turned out to have the potential to survive and grow in the Mars simulation chamber of the German aerospace center with daily temperature changes from below -40°C to above 15°C and also simulating the day to night humidity cycle (Zakharova et al., 2014. Protein patterns of black fungi under simulated Mars-like conditions)
E. jeanselmei is sometimes a pathogen itself and its close relatives are sometimes fatal. In a review of 84 case reports since 1980, 2 out of 29 patients with normal functioning immune system died and 7 out of 55 immunocompromised cases died (Wu et al., 2015, Cutaneous Phaeohyphomycosis of the Right Hand Caused by Exophiala jeanselmei:, 2022 : table 2). These were all classified at the time as E. jeanselmei, but many early cases may be other cryptic species of Exophiala that are clinically identical and can only be distinguished with gene sequencing such as such as E. heteromorpha, E. lecanii-corni, E. oligosperma, and E. xenobiotica, and E. jeanselmei itself is also sometimes an opportunistic pathogen of humans (Zeng et al., 2017, Spectrum of clinically relevant Exophiala species in the United States). This for example is a case from 2010 confirmed by gene sequencing (Badali et al., 2010, The clinical spectrum of Exophiala jeanselmei, with a case report and in vitro antifungal susceptibility of the species) as was the case studied by Wu et al. (Wu et al., 2015, Cutaneous Phaeohyphomycosis of the Right Hand Caused by Exophiala jeanselmei:, 2022)
E. jeanselmei is classified as a risk group 2 organism (moderate individual risk, low community risk) and can grow up to 37°C but not up to 40°C (University of Adelaide, n.d., Exophiala) [click to expand the Exophiala Jeanselmei section]
The paragraph from the Biological Safety Report which we are looking at in this section (Craven et al., 2021. Biological safety : 6) is also unusual for a scientific paper in a peer reviewed journal. It doesn't have any citations of previous writings on this topic in the planetary protection literature. Also, it has no cites for any of the diseases it does mention. This paragraph has only have one cite, which they use to show that humans have a common ancestor with snails and with mosquitoes 600 million to 1200 million years ago. This makes it impossible to look closely at the basis for their conclusions, especially for their statement about Shiga's toxin, which is controversial.
As already mentioned, when we look at the literature of these example diseases we find that the strain of e-coli that produces Shiga's toxin may have got that capability in biofilms rather than humans. That's a theory of Łoś et al. from 2013.
Łoś et al. reasoned that (Łoś et al., 2013, Altruism of Shiga toxin-producing Escherichia coli: recent hypothesis versus experimental results):
- in humans, this strain kills itself when it produces Shiga’s toxin – so it only benefits other e. coli altruistically by destroying the white blood cells (phagocytes) that attack them.
- in biofilms, this strain stays alive when it produces Shiga’s toxin –, the toxin only kills the attacking protozoa.
- e. coli strain 0157:H rarely spreads human to human. It only does this during rare outbreaks
In short, Shiga’s toxin seems to be more beneficial to e. coli in biofilms than in humans. Additionally, human outbreaks don’t spread often enough or far enough for much evolution.
There is some evidence both ways, for and against this hypothesis. A 2018 review paper by Sun et al. looks at the arguments both ways and concluded that it is a possibility that this strain of e.coli developed Shiga's toxin to allow it to survive within protozoa, accidentally creating a toxin that kills white blood cells in humans.
In conclusion, evolution of mechanisms that allow for survival within protozoa may have selected for traits that also allow bacteria to escape that harmful effects of phagocytes [in humans].
(Sun et al., 2018, Dual role of mechanisms involved in resistance to predation by protozoa and virulence to humans)
So even one of the biosafety report's nine diseases may have gained its toxicity for humans as a response to protozoan grazing in biofilms. Whether it did or not, this alerts us to the possibility of pathogens that can become hazardous to humans through adaptations to biofilms.
Whether or not Shiga's toxin did evolve in a biofilm, the better known example of Legionnaires' disease certainly did, a disease with no adaptations to humans. This is another example covered in the paper by Warmflash et al. which the Biological Safety Report somehow missed (Warmflash et al, 2007, Assessing the Biohazard Potential of Putative martian Organisms for Exploration Class Human Space Missions).
Their list of human pathogens misses out the important exception of Legionnaires’ disease , an example from (Warmflash, 2007). This a disease of biofilms and protozoa that also infects human lungs and sometimes can kill us, yet it’s evolved to live in biofilms, not to attack humans.
Researchers into Legionella say that to the microbe Legionella pneumophila, the macrophages in our lungs must seem like large protozoa (Alberts et al. 2002) and it can even persist in biofilms outside of any host (Warmflash, 2007).
Legionella pneumophila isn’t an exact analogue for a microbe we could return from Mars in these sample tubes, as it needs an oxygen rich fresh water environment to survive, can’t survive drying and can’t form spores. But it is a useful example to show a martian microbe could be preadapted to live in human lungs without ever encountering anything except biofilms and the protozoa that are often found in biofilms. It can infect our lungs because of a close resemblance in relevant details between the conditions L. pneumophila encounters in lungs, and in biofilms.
Warmflash used Legionnaires’ disease to challenge whether there is a need for human pathogens to co-evolve with us:
In essence, all that a potentially infectious human pathogen needs to emerge and persist is to grow and live naturally under conditions that are similar to those that it might later encounter in a human host. On Mars, these conditions might be met in a particular niche within the extracellular environment of a biofilm, or within the intracellular environment of another single-celled Martian organism.
…
To be sure, the genetic similarity between humans and protozoa is much greater than could be expected between humans and the Martian host of a Martian microbe.
…
Even in the context of a planetary biosphere that is limited to single-celled life, and even where there is unlikely to have been a co-evolution between agent and host organism, the possibility of infectious agents, even an invasive type, cannot be ruled out.
(Warmflash et al, 2007, Assessing the Biohazard Potential of Putative martian Organisms for Exploration Class Human Space Missions)
Other examples like Tetanus (mentioned by Warmflash) and Aspergillus fumigatus (which seems to be new to the planetary protection literature) show that soil pathogens don't even need to evolve to infect protozoan hosts or biofilms to harm humans. These organisms are not adapted to an infectious lifestyle in any host. See below:
Warmflash et al conclude that not even all infectious human pathogens require a multicellular macroscopic host to evolve harmful conditions and directly rebut Zubrin's finding:
While, based on the terrestrial examples, invasive capabilities will likely be rare among putative martian microorganisms (32), we cannot be sure that they will be non-existent, nor can we depend on the following a priori conclusion, as expressed by a popular Mars colonization enthusiast, that there is no evidence for the existence of macroscopic martian fauna and flora. Without indigenous hosts, the existence of martian pathogens is impossible (50). In fact, not even all infectious human pathogens - let alone non-infectious pathogens - on Earth require a multicellular, macroscopic host in order to evolve harmful capabilities.
(Warmflash et al, 2007, Assessing the Biohazard Potential of Putative martian Organisms for Exploration Class Human Space Missions : 14 - 15)
So NASA's report's finding is rebutted by Warmflash et al. NASA's team clearly were not aware of Zubrin's earlier presentation of a similar finding or Warmflash et al.'s rebuttal of Zubrin's finding, or they would have mentioned them.
There's an interesting earlier paper by one of the report's co-authors, Schuerger from 1998 (though not used as a cite in the report). He argues that each stage in the life-cycle of the pathogen require pre-adaptation to higher life forms, in this case plants, through genetically controlled recognition and responses:
The life-cycle of a terrestrial plant pathogen generally includes the following stages:
1) attachment of an infective propagule to a host surface,
2) germination of the infective propagule,
3) infection of host tissue,
4) inter- and intra- cellular growth of the pathogen into non-infected host tissue with acquisition of essential nutrients,
5) formation of reproductive structures by the pathogen,
6) formation of secondary infective propagules (i.e., spores),
7) spore dispersal, and
8) survival of infective propagules between life cycles of the pathogen.At each point within the life-cycle of a terrestrial pathogen, genetically controlled recognition and response phenomena occur between the constantly interacting pathogen and host. It seems very unlikely that a putative martian microbe separated by possibly 3 - 4 billion years of evolution (assuming Panspermia contributed to the exchange of life-forms between early Mars and Earth, [7,8]) or separated completely (assuming Mars and Earth always have been biologically isolated) would possess appropriate recognition, attachment, and develop- mental attributes to induce disease in terrestrial life-forms.
(Schuerger, 1998, Application of basic concepts in plant pathogenesis suggests minimal risk for return of extraterrestrial samples from Mars : 1594)
However though this is true of many pathogens, it's not true of some of the soil pathogens. Clark rebuts arguments like this, by showing that soil pathogens from Mars could be pre-adapted because of resistance to martian surface conditions. These opportunistic pathogens like tetanus (or this book's new example of Aspergillus fumigatus), don't rely on recognition and adaptation but simply resist the mechanisms that the immune system and our body uses to try to dislodge it in the same way that it resists hostile conditions it may encounter in the soil.
Clark comments also that for animals, their interior has much to attract a pathogen. Especially, a warm-blooded animal is warm, wet and with a cornucopia of nutrients. He suggests that the mechanisms microbial life use to resist hostile conditions on Mars could help resist the acids in the stomach, and cause stomach ulcers and stomach cancers, or they might have a different protein chemistry or even an inorganic cell wall and confound the immune system in that way. He also draws attention to the way that some vertebrates including some humans are immunocompromised which can make them vulnerable to pathogens that a healthy vertebrate would easily overcome.
As unlikely as successful infection is in a healthy vertebrate, we must be also be cognizant that some animals, particularly humans, may be immunocompromised due to poor health (e.g., age or AIDS) or purposely immunosuppressed due to medical treatment (e.g., organ transplants or side effects of a critical medication). Furthermore, the interior of an animal, especially the warm-blooded variety like us, is by definition a warm, wet spot with a cornucopia of nutrients readily available. These are the ideal conditions sought in the search for life in favorable environments on some alien planet, such as Mars.
Numerous life forms can survive quite well in such environments. Suppose that martian organisms happen to be resistant to acid (since the martian soil may be strongly acidic), are normally dormant as cryptobiotic forms (spores or cysts) but are designed to multiply at extremely high rates as soon as they are exposed to liquid water with nutrients (the wintering-over hypothesis). Such an organism just might be compatible with the interior of a vertebrate's gut, much as the bacterium Helicobacter pylori (causative agent for approximately 90% of cases of stomach ulcers, as well as some stomach cancers) lives inside the human stomach. Or, cell walls designed to survive the UV and oxidants present at the martian surface might confer resistance to the acids of the upper gut, allowing the putative organism to safely reach a more hospitable environment in the lower gut, much as is accomplished by spore coats of certain microbes. If martian organisms have a different protein chemistry or include an inorganic wall, their surface markers may be quite different from those of normal pathogens, thereby confounding the immune system's ability to recognize them as potentially dangerous entities.
(Clark, 2002, Martian meteorites do not eliminate the need for back contamination precautions on sample return missions : 1597)
We can't conclude a high risk of a soil pathogen from Mars from this research. However, there is enough material here to make it clear that it does not have a near-zero probability, based on our current knowledge of Mars and of extremophiles, and needs to be considered seriously in a search for a likely low risk but high consequence worst case scenario, which is what we have to do for responsible risk assurance.
Warmflash et al argue benefits for humanity of humans on Mars outweigh risks of returning novel pathogens - starting point for a debate about acceptable levels of risk which NASA bypasses by mistake
Warmflash et al found a possibility astronauts on Mars could be harmed by extant microbes there and bring back pathogens to Earth. They concluded however, that we should accept these risks because in their view they are outweighed by the benefits of human exploration of Mars. They suggested we contain them as far as possible, through biological containment on Mars and quarantine on return to Earth., writing:
Since the discovery and study of martian life can have long-term benefits for humanity, the risk that martian life might include pathogens should not be an obstacle to human exploration.
(Warmflash et al, 2007, Assessing the Biohazard Potential of Putative martian Organisms for Exploration Class Human Space Missions : 2)
Many wouldn't agree with Warmflash et al. that the risk of novel and potentially serious pathogens from Mars is counterbalanced by long-term benefits to humanity of astronauts on Mars. However this could be the start of a debate about what to do, based on a careful assessment of potential risks and the extent to which we can manage them.
This would lead to an open public debate about what is acceptable by way of risk, if anything, and what level of risk is acceptable for human health and Earth's biosphere.
The issue with NASA's PEIS is with its starting point which has bypassed all these ethical discussions with its novel non peer reviewed findings. It needs to grounded in the science and the existing literature on planetary protection.
How did NASA miss these issues? Focus on best case scenarios when for risk assessment the focus should be on worst cases
So how is it that NASA's risk assurance came to be not well grounded in science and the literature on planetary protection? It may be due to lack of experience in risk assessment of the preparers of the PEIS. That may seem surprising, as NASA have a history of careful risk assessment. However this seems to be an example of a rare lapse in their usually careful work. The preparers were not able to answer many questions they were asked on the topic by an expert and seemed out of their depth. See below:
We can see this issue rather clearly with this example of the soil pathogens from Mars.
If the focus is on worst case scenarios then after finding 10 diseases that co-evolved with higher hosts, the natural next question is the one Warmflash et al must have asked while compiling their report:
Are there any diseases of humans that didn't co-evolve with us?
It doesn't take long from starting a literature search based on that question to find the examples of tetanus, many fungal diseases, etc. we just looked at.
So the lapse here is that NASA's biosafety assessment team doesn't ask this question in the report.
To help motivate space agencies to take care when they return martian materials to Earth I worked up some vivid worst case scenarios. One of my scenarios for human health is a species from a novel fungal genus from Mars similar to Aspergillus fumigatus which is not adapted to an infectious lifestyle in any organism, even microbes or biofilms. With a close look at it we find that it shares many characteristics with martian life. It harms 200,000 immunocompromised a year with a serious lung disease with a fatality rate of 35% to 90% and 400,000 have the opposite issue, a serious chronic progressive allergic lung disease. A species from a novel fungal genus from Mars with similar properties would be far more serous as we have never encountered it before.
This would also be an exceptionally serious pathogen for wild birds, pet birds and domestic poultry which are severely impacted by Aspergillus fumigatus with die-offs that can kill a farmer's entire turkey flock.
- The novel fungal genus scenario also doubles as an illustrative worst case for poultry and wild bird
These are not meant to be likely scenarios particularly, and especially not likely for the first microbes returned from another planet, but we do need to look at worst case scenarios rather than only the scenarios we consider to be most likely, to protect Earth adequately.
In the Supplementary information section, we also look at the capacities for completely alien life to harm humans. This is a first time in the planetary protection literature. Sample return studies recognize there is a potential risk for alien life but there don't seem to be any other papers that look into this with vivid concrete example scenarios. There are only two papers in the literature that touch on the topic, both by Joshua Lederberg, pioneer in planetary protection from the late 1950s who got his nobel prize for his discovery of bacterial sex.
The papers are from 1999 and he found a possibility of a "zoonosis to beat all others" which he meant in a non standard general way as a microbe from Mars able to harm humans. I looked for terrestrial analogues for potential modes of action of accidental or opportunistic martian pathogens based on alien biology and found several. Google scholar turns up only 19 cites. The ESF Mars sample return in 2019 adds an extra cite they missed, for a total of 20 but none of these add to his work. It seems important to make a start on this topic and I found several useful terrestrial analogues for a starting point based on advances in science since Lederberg's two papers.
Several of them are based on Aspergillus fumigatus, because it is essentially agnostic about the human immune system and is one of many such fungi. They protect themselves with biopolymers and chemical barriers and they insert tendrils (hyphae) to inject corrosive chemicals into the tissues to extract organics which they then digest. An alien microbe could use a similar method with no knowledge of our immune system. Our immune system defends against fungi with pattern recognition methods but these would not be likely to work with an alien biology. If our immune system did recognize a threat it might also overreact with an allergic response.
I also look at analogues based on microplastics
- Nanoplastics and microplastics as an analogue of alien life that doesn't even notice terrestrial life but still gets through our skin and lungs into our blood stream which then covers them in plasma coronas that stick together in blood clots
- Totally alien life could cause allergic reactions or sterile inflammation similarly to silicosis - analogy of microplastics and nanoplastics
- Alien life with a different vocabulary of amino acids to make its proteins could lead to protein misfolding as a result of alien amino acids getting attached to transfer RNA in our cells - similarly to BMAA which causes protein misfolding and is neurotoxic and may be a contributing cause for neurodegenerative diseases such as the one Steven Hawking suffered from
This seems to be a common theme in NASA's approach to risk assurance in this PEIS which we see in all four of these plausible seeming findings. They look for the best rather than worst cases.
- best planetary protection case for the Mars meteorite argument is a microbe that can get here on a meteorite
- NASA found such microbes in the literature such as, b. subtilis, though if Mars has such microbes it likely gets here rarely
- but for planetary protection we need to look for the worst case of microbes that can't get here and it doesn't take long to find examples
- they also didn't check to see if anyone has rebutted their Mars meteorite argument even in their main cites - best planetary protection case for habitability is an uninhabitable Mars which is the conclusion of the PEIS
- there seems to be some potential that Mars is uninhabitable for terrestrial life
- best case for scientific interest is a Mars with present day life which makes this the main focus of the iMost team
See above: NASA's uninhabitable Mars argument rebutted by its own plans to test for life in its samples . - best planetary protection case for a pathogen is one that requires co-evolution with humans other higher organism hosts
- NASA's team found 10 diseases, all of which seemed to have co-evolved with humans or other higher organism hosts (though one is controversial)
- but for planetary protection we need to look for microbes that had no adaptations to any host and NASA's team didn't look for these
See above: NASA's Biological Safety Report's finding of near zero risk that martian life can harm us rebutted by soil pathogens like tetanus and aspergillus fumigatus not adapted to any terrestrial host - best case for extremophiles on Mars is if they can't survive on Earth
- there are many extremophiles that only live in extreme conditions
- but for planetary protection we need to hunt for evidence that some might be able to survive on Earth and there was an example of this in the very cite NASA uses.
See next section: NASA's Biological Safety Report's finding that any martian microbe would not be viable on Earth rebutted by the only plausible Mars analogue in its cite: Planococcus Halocryophilus
We should be looking for worst case scenarios for risk assurance to do planetary protection properly.
NASA's Biological Safety Report's finding that any martian microbe would not be viable on Earth rebutted by the only plausible Mars analogue in its cite: Planococcus Halocryophilus
With all of the other three findings rebutted now, the only finding left to look at is that it is impossible for any life from Mars can live on Earth anyway. If this was true then we wouldn't need to worry about pathogens either. The martian soil pathogens wouldn't be able to live on Earth and human bodies would be just too warm for any martian life to affect us.
This again may seem plausible at first given how very cold conditions are on Mars, and very dry, and the perchlorates and UV. It is such an alien world, just as it would be hard for terrestrial life to live there, wouldn't it be hard for martian life to live here?
However with some background on extremophiles on Earth, and on the possibilities of underground refuges on Mars, one might see things a bit differently. Perhaps surprisingly, many microbes that live in the coldest places on Earth actually are very tolerant of warm conditions and some grow optimally at room temperature or higher. They have the capability to live in very cold temperatures but they don't require such cold temperatures.
It turns out that NASA's own cite has one of these extremophiles, which the team don't seem to have noticed, a microbe that grows best in warm conditions but that is found growing in ultracold conditions as well, isolated from the permafrost.
Here is how they put it, with a few of the earlier sentences to give it context, with the relevant sentences highlighted in red:
QUOTE:
For example, could martian material somehow interfere with the ability of photosynthetic bacteria in Earth’s ocean to fix carbon dioxide and produce oxygen?
…
Planetary protection must consider not just human health directly, but the entire biota of Earth. Organisms evolve to live in a particular environment, and while some are generalists, others can only survive in very specific conditions. In all cases, organisms do not replicate if critical nutritional or environmental conditions are lacking.
…
Invasive species or pathogens have been successful on Earth because they were adapted to similar environmental niches in their original and dispersed ecosystems (Bleuven and Landry 2016). There are many described extremophiles that may survive in environments that are extreme to human or animal life (e.g. extremes of temperature or pressure) but do not survive under conditions in our normal habitat (Merino et al. 2019).
Thus, it is plausible that any martian microbe, after it arrives on Earth, would not be viable on Earth due to a lack of its required martian nutritional and environmental conditions. Indeed, the martian environment is inhospitable to Earth life, but conversely, the Earth environment is likely to be just as inhospitable to martian life. Based on these factors, a very low qualitative probability of biological risk can be assumed.
(Craven et al., 2021. Biological safety : 6)ANNOTATION:
The only Mars analogue extremophile in Merino et al., Planococcus Halocryophilus, grows up to human blood temperature, has optimal growth at 25°C, was isolated from permafrost at around -16°C and has metabolic activity down to at least -25°C
NASA's only cite on extremophiles for this augment, Merino et al, lists only one plausible Mars analogue in temperature range, which is a counterexample: Planococcus Halocryophilus: (Merino et al., 2022, Living at the extremes: extremophiles and the limits of life in a planetary context: table 3)
This microbe has
- Optimal growth at 25°C
- Grows up to 37°C (human blood temperature)
- metabolic activity down to -25°C, the lowest temperature tested and may grow at ultra low temperatures too slowly to test in the laboratory.
- was isolated from Canadian permafrost with ambient temperature around -16°C.
see: (Merino et al., 2019, Bacterial growth at− 15°C ; molecular insights from the permafrost bacterium Planococcus halocryophilus Or1)
Text on graphic: Merino et al.'s table of extremophiles has one remarkable exception with a very broad temperature range
Planococcus Halocryophilus
Optimal growth 25°C. Metabolic activity at least to -25°C. Found at -16°C.
(Merino et al., 2022, Living at the extremes: extremophiles and the limits of life in a planetary context: table 3)
Then another thing that gives pause for thought is that Mars has been geologically active in the not very distant geological past, and may have subsurface caves warmed by geothermal process, such as sulfur caves, even today. So there could be microbes there used to warm and even very hot conditions underground, that also have the ability to live in cold surface conditions. This is another big surprise of terrestrial microbiology, the "geobacillus paradox" that spores adapted to high temperatures can be found in all habitats on Earth in surprisingly high concentrations. See Supplementary information section under:
In most places, the geobacillus spores are just dormant, waiting for hot conditions to thrive, but they are everywhere in surprisingly large numbers. So it's not so impossible that Mars might have microbes, even with surprising abundance, either living there or ones that are just dormant, and adapted to warm and even very hot conditions.
Many candidate Mars analogue terrestrial organisms do well in Mars simulation chambers, showing a possibility for martian life to be able to live on both planets too.
This is the problem of invasive species. Clark puts it like this:
Even in the case that a martian organism does not directly interact with, or parasitize terrestrial organisms, there is reason for concern. This arises from the serious current ecological problem known as "biological invaders." Invader organisms are those which are transported to a location on Earth where they have not been previously present. Although it is sometimes argued that highly efficient organisms already fill all available ecological niches on Earth, such is clearly not the case.
Numerous problems have occurred as invaders from all levels of biology, including birds, worms and human beings themselves, have upset an ecological balance and driven one or many species to extinction.
Microorganisms have caused problems as well. If a martian microorganism could survive and then thrive in one or more environments on Earth, the concerns would be very great if they were allowed access to such environments. Microbes perform essential functions in recycling carbon, nitrogen, sulfur and phosphorus compounds in the biogeosphere. Without this global microbial ecology which makes nutrients available, the macroscopic organisms - not just animals, but also the natural flora as well as agricultural crop plants - would not survive.
The space program would receive the blame for poor control of any alien life form because of the specter of dire consequences. Such blame could be severe, possibly resulting in more ramifications than would be logical or would occur in cases where publicity and the sensational aspects were of lesser visibility.
(Clark, 2002, Martian meteorites do not eliminate the need for back contamination precautions on sample return missions)
For an example of a microbial invader we have invasive freshwater diatoms such as diatom "Didymo" (Didymosphenia geminatum) which causes many problems in New Zealand (Spaulding et al., 2010. Diatoms as non-native species). They can be invasive because they are confined to fresh water and can't easily cross oceans. Similarly any microbes on Mars that can't survive the shock of ejection and the vacuum and cold of travel through interplanetary space has potential to be invasive, even if it is related to terrestrial life, as we saw above.
See
To encourage space agencies to take this possibility seriously I developed a concrete scenario of a Martian analogue of Chroococcidiopsis, a blue-green algae that does well in Mars simulation chambers.
Strains of chroococcidiopsis are found almost anywhere on Earth from Antarctic cliffs to reservoirs in Sri Lanka, tropical seas, and even in complete darkness with no source of energy except hydrogen tens of meters below the sea bed, using alternative metabolic pathways it has accumulated over billions of years. It is also sometimes found in the human microbiome, for instance in the nasopharynx.
Chroococcidiopsis is a genus, and those strains are like species though since they are asexual, they are a little different from how normal species function. Some strains of chroococcidiopsis are good candidates to survive almost anywhere on Mars if there is liquid water available.
This seems a plausible scenario for the probably low likelihood case where Mars has had a second genesis, and life on Mars has survived to the present. Even those who are most enthusiast for humans on Mars might well agree that if we did find a scenario like this on Mars. we wouldn't send humans there because of the risk of returning mirror life to Earth. Though we could still send humans to Mars orbit. See below:
- Mirror life as a potential astrobiological surprise like the unexpected carbon dioxide geysers for geology
- Scenario of a mirror life algae from Mars pre-adapted to normal organics brought to Mars by infall from space
Need for peer review for an issue of global import as one of the best ways to ensure scientific integrity for novel reasoning
NASA preparers of the PEIS confirmed that its central planetary protection argument hasn't been through peer review. They confirmed this in their response to a comment I made in the second round of comments required by NEPA.
I asked NASA to provide a cite for the most important planetary protection sentence in the PEIS, their conclusion that any environmental effects would not be significant. NASA said the reference is the PEIS itself.
Robert Walker: "This sentence is not cited"
NASA: —the reference is the PEIS itself.
(NASA, 2023. Mars Sample Return final PEIS : B-67, response on B-68)
In a separate comment I said the public deserve peer review. NASA said NEPA doesn't require this.
Robert Walker: The public deserves it to be peer reviewed first by independent reviewers not connected with the project
NASA: NEPA does not require a “peer” review prior to release. The purpose of releasing the Draft PEIS is to allow the public, agencies, and other interested parties to review the document and provide substantive comments on the alternatives and/or analyses presented
(NASA, 2023. Mars Sample Return final PEIS :B-71)
Text on graphic: NEPA does not require peer review, but it DOES require scientific integrity. Peer review is one of the best ways to ensure this when the reasoning used is very novel.
For this book's discussion of their reasoning in the PEIS to this conclusion see below:
NASA is correct to say that NEPA doesn't require peer review of the plans. However it does require scientific integrity § 1502.23. The easiest way to achieve this is with peer review
See:
Those public comments and their replies become of especial significance because of the potential global import of NASA's plan. The aim is to protect Earth's entire biosphere and inhabitants from any life there might be in samples from Mars. Eventually NASA's findings and reasoning is sure to be discussed in the academic literature, but for now this is all we have by way of comments on NASA's novel plans and responses by NASA.
NASA is widely respected on planetary protection, so we can expect other space agencies and private space companies will follow its lead as will NASA itself for later missions. We need to be sure any plan they produce is credible, both for the mission itself, and for the precedent for other missions by other spare agencies, and private space, and indeed future missions by NASA itself.
It is especially important to maintain scientific integrity for a biosafety plan for Earth's biosphere and its inhabitants.
Aim of this book to start a process which will shine the light of peer review on current biosafety plans
[THIS SECTION MAY NEED TO BE UPDATED BEFORE PUBLICATION BASED ON LATEST DEVELOPMENTS / OUTCOMES]
The preprint of this book will be shared to NASA before submission for publication. I hope this initial courtesy and attempt at bridge building will work This is likely to be a matter for decisions at a higher level in NASA than the current team, as one of the major issues is the choice of disciplines for the preparers. So it may take some time.
I hope the outcome will be that NASA withdraw the PEIS to work on another way forward to resolve these many issues. Agencies do occasionally withdraw final EIS's. A search of the Environmental Impact Statement database (EPA, n.d., Environmental Impact Statement Database) for "withdrawn" turns up 76 withdrawn out of 16,262 submitted (including drafts and supplements) as of 07/20/2023 or 1 in 214, including 20 final EIS's withdrawn.
If NASA withdraw its final PEIS, as suggested in this book, this will be an important move as the discussion can then shift to a new direction, with many others involved, including experts familiar in the planetary protection literature for a Mars sample return and others of appropriate disciplines depending on its plans,
I hope NASA will also take up the recommendations of all the major sample return studies to involve the public fully at an early stage and to include on their team experts in public communication, risk assurance, and to set up fora open to representatives from all countries globally because negative impacts could affect countries beyond the ones involved directly in the mission (Ammann et al., 2012, Mars Sample Return backward contamination–Strategic advice and requirements : 59)
In that case the suggestions given here will likely be only some of many alternatives for NASA to evaluate according to the biosafety and the science return amongst other criteria. This will involve a much wider participation of the general public and of scientists from other disciplines and internationally, at a far earlier stage than we saw with the current PEIS.
The aim of submitting this paper for peer review and publication is to start a process of shining the light of peer review on current biosafety plans to protect Earth's biosphere. I hope this will become part of a wide-ranging peer reviewed debate amongst scientists, ethicists and others about how to refine these and other plans to protect Earth's biosphere effectively. I hope it will also encourage work more generally on how to continue to promote discovery in space, adventures into the unknown, science return and human and robotic exploration of space in a way that is always safe for Earth's biosphere whatever we might find in our solar system by way of extraterrestrial life.
This book focuses on NASA's current biosafety plan as the first published. Neither NASA's PEIS nor my comments on it during the NEPA process or its replies have been through any previous process of peer review.
[ASSUMING THIS BOOK OR A PAPER BASED ON IT IS ACCEPTED FOR PUBLICATION]
If NASA remain of the view their current PEIS doesn't need revision, I hope it will be encouraged by this book to publish a paper of its own in response which would also go through peer review, so we can have a clear look at the scientific basis for its plans and any of its response to the issues raised here.
This paper is part of any larger discussion whatever NASA's decisions, with its discussion of
- quarantine issues,
- lab leaks,
- issues with complying with the ESF standard,
- vivid planetary protection scenarios like mirror life,
- impossibility of containing unknown life from another planet without a fully telerobotic facility,
- details of my alternative solution of a miniature lab above GEO based on the Europa lander in situ life detection mission NASA / ESA designed together in 2016.
- suggested revision of NASA's intermediate goal of humans on the surface of Mars to humans on Phobos / Deimos until we know if it is safe to land.
Essentially the same paper but I hope NASA will withdraw the PEIS to prepare for a new way forward.
Why a new Mars sample return study is vital to prepare thoroughly for an unsterilized sample return 14 years after the last study
NASA rather surprisingly did this PEIS without doing a new Mars Sample Return review. The last major study from 2009 is based on science that is now at least 14 years out of date (SSB, 2009, Assessment of planetary protection requirements for Mars sample return missions). The 2009 study was published 12 years after the previous major study in 1997 (SSB, 1997, Mars Sample Return: Issues and Recommendations (1997))and 2009
Then the ESF size limit review focusing on the Mars Receiving Facility is also based on science of over a decade ago (Ammann et al., 2012, Mars Sample Return backward contamination–Strategic advice and requirements) and one of its recommendations is that the size limit and level of assurance needs periodic review, which is certainly needed now.
Not only that, the 2009 study was published just before a major surge in research into novel potential habitats and microhabitats on Mars. It was published just before the publication of the discovery of the Phoenix leg droplets, the first of many discoveries that lead to a picture of a significantly more habitable Mars than expected in 2009 (Rennó et al., 2009, Possible physical and thermodynamical evidence for liquid water at the Phoenix landing site).
This is a truncated version of the sequence for the Phoenix lander droplets, turned into an animation:
Caption: Possible droplets on the legs of the Phoenix lander – they appeared to merge and sometimes fall off. In this sequence of frames, the rightmost of the two droplets grows and seems to do so by taking up the water from its companion to the left, which shrinks – highlighted in green on this black and white image
(Gronstall, A., 2014, Liquid Water from Ice and Salt on Mars)
Nilton Rennó was part of the team that discovered the droplets on the Phoenix legs. In 2014 Rennó and his team used their recently built Mars Environmental Chamber (Fischer et al., 2013, The Michigan Mars Environmental Chamber: Preliminary Results and Capabilities) and found droplets like these form within a few tens of minutes at -50 °C when salt overlaid ice (Fischer et al., 2014. Experimental evidence for the formation of liquid saline water on Mars). After they achieved this, Rennó said
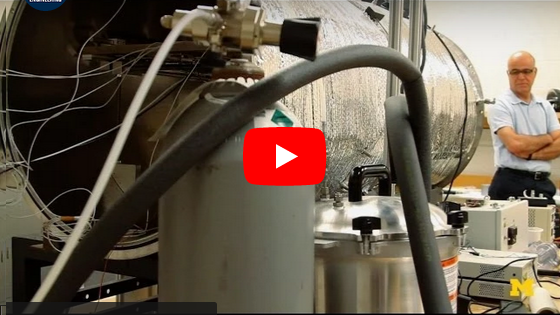
Video: How Liquid water forms on Mars
This is a small amount of liquid water. But for a bacteria, that would be a huge swimming pool – a little droplet of water is a huge amount of water for a bacteria. So, a small amount of water is enough for you to be able to create conditions for Mars to be habitable today. And we believe this is possible in the shallow subsurface, and even the surface of the Mars polar region for a few hours per day during the spring.
(Rennó, N., 2014, Video: How liquid water forms on Mars),(transcript from 1:48 onwards)
This was first of numerous discoveries, suggestions for microhabitats, new results about extremophiles and transport in dust storms which happened in the last 14 years. We would expect NASA to do a new study first to take account of all this new research on relevant topics.
Based on my literature survey I have done a list of some of the topics a new Mars sample return study would expect to cover, topics new since the last major study:
There doesn't seem to be any sample return study or a literature survey or any large-scale review available for the field more recent than the ones in 2009 and 2012 already mentioned. Not only that there seems to be no prospect of any such study before the Perseverance sample return in the 2030s.
So, I decided to do a preliminary literature survey myself. I needed this to be reasonably up to date on the latest research, given how wide ranging and rapidly changing the field is. It turned out to be valuable background material for this book.
Space Studies Board told NASA it needs external peer review to ensure adequate planetary protection
NASA is not expert in biosafety and the Space Studies Board stresses the need for external peer review of its plans.
Recommendation 3.6: NASA should reestablish an independent and appropriate advisory body and process to help guide formulation and implementation of planetary protection adequate to serve the best interests of the public, the NASA program, and the variety of new entrants that may become active
The roles of the advisory body include the following:
[other roles] …
Act as a peer review forum to facilitate the effectiveness of NASA’s planetary protection activities.
(SSB, 2018, Review and Assessment of Planetary Protection … : 61 - 62)
NASA are correct to say NEPA doesn't require peer review. However it does require agencies to ensure scientific integrity.
"Agencies shall ensure the professional integrity, including scientific integrity, of the discussions and analyses in environmental documents"
§ 1502.23
Peer review is one way to achieve scientific integrity. However the general public aren't reviewers, and the public comments haven't been through peer review either.
Given the high level of attention for a Mars sample return mission, it's not credible that NASA's plan can survive through to the 2030s when the samples are returned without peer review.
NASA's plans are bound to get responses eventually, as academics write papers about its plans. This book will provide it sooner rather than later. Unlike NASA's original PEIS I will submit papers based on this book to major journals who will then provide the peer review that is clearly needed for a matter of this level of significance.
I recommend that NASA do the same with its plans if they have responses to the serious issues I've raised in this book or to elaborate on their reasoning. In this way we can bring the spotlight of peer review to NASA's biosafety plans. Of course if I have made any mistakes in any of this however minor or major, I welcome corrections and this is something peer review will check for.
The current near impossibility of public dialogue with experts at NASA or elsewhere about potential major issues with NASA's biosafety plans
Many of the issues raised here could perhaps have been resolved in one way or another through dialogue at an early stage. However NASA doesn't seem to provide any avenue for public engagement except as legally required under NEPA. My experiences here may perhaps serve as an example for NASA and other agencies of the value of enabling two-way dialogue at an early stage.
I will ask NASA if there is any opportunity for further dialogue that I missed here. I will also ask if they wish to correct any inaccuracies in my account of their findings, conclusions or anything else I said about NASA in this manuscript. If I get any other response or clarification I will update this draft book accordingly.
This section has some repetition of quotes and other material from the rest of this book to show it in the context of attempts at dialogue with NASA and others.
You can get an overview of this section by reading just the sentence in bold.
The sample return studies all emphasize the need to encourage the public to get involved in risk governance for a Mars sample return mission at an early stage. Early and frequent engagement seems likely to be key for the success of the mission according to experts.
See:
There seem to be considerable barriers to be overcome to achieve that goal of involving the public in risk governance. My experiences as described here show how hard it is at present for a member of the public or even an independent researcher working on a paper for NASA's very mission, to try to raise concerns about specific biosafety plans for a Mars sample return mission.
This section ends with some practical suggestions for ways to remove the barriers to achieve more open dialogue with the public than is possible at present.
At the time the PEIS started, I was about to finish my first paper in astrobiology, on planetary protection for NASA's mission. Amongst other things, it found that existing biosafety labs can't fulfil the ESF recommendation to contain 100% of ultramicrobacteria, as mentioned in the previous sections of this book, starting from:
- Why NASA can't rely on HEPA filters for the ESF recommendation to contain 100% of ultramicrobacteria
My original preprint also looked at the vexing issue of quarantine for lab leaks, which doesn't seem to have been looked at closely since the Apollo era:
I found existing plans published this century for Mars Receiving Facilities run by human technicians have no solutions so far to these two issues. Indeed they don't look at them.
They include no plans for how to respond to lab leaks of potentially harmful life if it can't be contained using quarantine. They also have no way to contain 100% of ultramicrobacteria sized particles only 0.05 microns upwards.
My original paper (and now this book) then proposes the solution of a miniature life detection lab above GEO. It gets to this solution by taking as a starting point a terrestrial solution to the two issues:
- a lab staffed by telerobots which don't need to be quarantined if they are contaminated, but can just be sterilized
- plus the entire lab can then be sealed to any desired containment requirement
That is a possible solution, but no lab has ever been designed like this before, and it is likely to be expensive to build and lead to significant extra time and cost to make sure operators are familiar with how to operate the facility to contain samples reliably. It also has issues of end of lifetime sterilization (for instance if contaminated with mirror life) and there's the potential for plane crashes, criminal damage, etc, for the highest levels of assurance.
So my original paper combines this with ideas from the joint ESA / NASA proposal from 2016 for an in situ life detection mission as far away as Jupiter's moon Europa (Hand et al., 2017, Report of the Europa Lander Science Definition Team : xi). That is what led to the solution of a receiving lab which is miniaturized and placed above GEO and run remotely through telerobotics. Humans never go near it.
This
- solves the problems of quarantine as there are no lab leaks.
- solves the issue of air filters as it doesn't depend on HEPA filters or any other air filters to contain the samples
- is future proof for any decision on the minimum size or on the level of assurance, as any materials returned to Earth are thoroughly sterilized.
For details, see:
I also did a sketch for a terrestrial telerobotic lab future proof for any size limit / level of assurance.See the Supplementary information section under:
Though if we need a more elaborate lab later on, and we have a substantial presence on the Moon by then, that may be an easier place to build it because of the natural containment of the lu8nar vacuum and separation from Earth.
For more on my background see the Supplementary information section under:
- My background, I was working on my first astrobiological paper when the NEPA comments process started, about NASA's Mars sample return mission, not yet submitted to journals
- My main strength is interdisciplinary
So that is what I expected NASA's plan to be focused on, how to protect Earth's biosphere from a likely low risk of large-scale harm to human health and the environment. My original preprint about NASA's mission was based on NASA's planetary protection policy as it was described by the first two planetary protection officers.
As we saw, NASA's second planetary protection officer Cassie Conley touched on these plans in her video which is still in the official NASA YouTube channel, saying they plan to contain the samples as if they were the most hazardous Earth organisms known. See above:
My original preprint was based on these earlier plans. Its discussion of HEPA filters, the ESF recommendations and of quarantine raised some issues NASA would need to look at when it got to a more detailed proposal, and then with the miniature lab above GEO it provides a reasonable alternative to solve the problems I identified.
However when NASA published their draft PEIS I was astonished to see NASA didn't even discuss this issue of how to contain samples to protect Earth's biosphere against large-scale effects. They simply said there would be no significant environmental effects (NASA, 2023. Mars Sample Return final PEIS : 3-16) and any health effects would be negligible (NASA, 2023. Mars Sample Return final PEIS : 3-14), as we've seen:
Also as we saw, NASA do think there is enough of a potential for life in Jezero crater to test the samples for metabolism and respiration and to see if they can get anything to grow from them (Beaty et al., 2017. iMOST : 92-3). See:
In place of the usual discussions of large scale effects the PEIS has four findings on the harmlessness of the samples, which their first planetary protection officer and other planetary protection experts had rebutted over two decades ago in 2000 (Rummel et al., 2000, Opinion: No Threat? No Way : 5).
I would never have guessed that NASA itself would promote those ideas in their PEIS. My original preprint covers the rebuttal of Zubrin's arguments by NASA's own first planetary protection officer and others in the section:
Title: "Zubrin's arguments in: "Contamination from Mars: No Threat" - not likely to be decisive in legal process - response of planetary protection experts in "No Threat? No Way"
[And following pages]
(Walker, 2022, NASA and ESA are likely to be legally required to sterilize Mars samples to protect the environment until proven safe : 136)
[As submitted to the final round of public comments. I continue to make minor fixes, and the latest version is at: (Walker, 2023, NASA and ESA are likely to be legally required to sterilize Mars samples to protect the environment until proven safe).
However NASA never got to see this summary of the rebuttals of Zubrin's arguments, and so also of their findings, because it prescreened the preprint for my original paper as nonsubstantive, my attachment 8 (NASA, 2023. Mars Sample Return final PEIS : B-28). The PEIS doesn't cite Zubrin's op. ed., and doesn't cite the four findings to any other source either. So it is possible NASA's team didn't know about Zubrin's earlier publication of their four findings. They surely didn't know they were rebutted or they would have discussed the previous rebuttal.
NASA gives no cites to previous statements of their four main findings. It presents them as new to the published planetary protection literature. As we saw. NASA's reasoning for these four findings has major issues of scientific integrity, and doesn't depend on any new evidence. However, it seems that so far at least, from the PEIS and their official reply to the public comments that NASA's team are still unaware of these issues.
Indeed NASA's reasoning for their four novel findings lack scientific integrity, as we saw
- any harmful life can get here better protected in meteorites (NASA, 2023. Mars Sample Return final PEIS : 3–3),
rebutted by its main cite (SSB, 2019, Planetary protection classification of sample return missions from the martian moons : 5) - Mars has been uninhabitable for millions of years (N 3. Mars Sample Return final PEIS : 1–6)
rebutted by its own plans to test for life including metabolism and respiration in the samples (Beaty et al., 2017. iMOST : 92-3). - any martian life would be unable to survive on Earth (Craven et al., 2021. Biological safety : 6)
rebutted in their own cite, by the only Mars relevant extremophile Planococcus Halocryophilus shown with a range of -15°C to human blood temperature, 37°C (Merino et al., 2022, Living at the extremes: extremophiles and the limits of life in a planetary context: table 3) - martian microbes would have near zero chance to harm humans because of the lack of similar hosts on Mars (Craven et al., 2021. Biological safety : 6)
rebutted by soil pathogens and toxins that are not adapted to an infectious lifestyle in any organism such as tetanus (WHO, n.d., Tetanus). and Aspergillus fumigatus (McCormick et al., 2010, Aspergillus fumigatus: contours of an opportunistic human pathogen)
See:
We see the effects of NASA closing down its planetary protection office in 2017 (Voosen, 2017, With planetary protection office up for grabs, scientists rail against limits to Mars exploration), and its interagency panel in 2006 (SSB, 2018, Review and Assessment of Planetary Protection … : 26 - 27).
It is clear from the PEIS that NASA's Mars sample return team had nobody left familiar with planetary protection as previously understood or the planetary protection literature for a Mars sample return mission or they would have known about the rebuttals of the Mars meteorite argument and their other four findings and would surely have discussed these rebuttals.
Also there was nobody left on NASA's team familiar with basic ideas of public health such as soil pathogens or they would have discussed those. This is one of many examples where NASA's PEIS shows that they failed the NEPA requirement to included experts of the appropriate disciplines amongst the preparers of the PEIS. See Supplementary information under:
I tried to get in touch with someone at NASA to discuss serious concerns with the draft PEIS, but with no response. I only found out why I got no reply when they published the final PEIS. It may be useful to say a bit in detail about what happened.
Let's look at an example of how the PEIS ignores a serious issue I raised, that there was no mention of the European Science Foundation (ESF) recommendations from 2012, the most recent study for planetary protection for a Mars sample return mission. This is one of many examples in their official responses to my comments where NASA
- didn't notice an issue I raised in the first or second round of public comments, or
- its official answer didn't resolve my concerns, or
- our comments got lost in the process of aggregation and prescreening (as with all the issues I raised in the first round comment and many in the second round)
I gave my email address to NASA under NEPA, when I filled in the form to submit my comments, for all my comments, in both rounds of public comments.
I raised one of the most serious issues I found with NASA's plans in the first sentence of my first comment in the first round of public comments [before the draft PEIS]. I found no discussion of whether NASA's plans can comply with the revised ESF size limit and level of risk assurance from 2012 of 100% containment of the very small ultramicrobacteria (which don't need to be contained in normal biosafety labs):
Are you aware of the ESF Mars Sample Return study (Ammann et al, 2012:14ff)? It said "The release of a single unsterilized particle larger than 0.05 μm is not acceptable under any circumstances”.
(Walker, 2022, Comment posted on May 16th by Robert Walker to NASA’s first request for comments)
I found no mention of this issue or any response to it by NASA in the section of the draft PEIS where NASA gives its official answers to issues raised in the first round of public comments. Also the draft PEIS still didn't cite the ESF study in its references list.
So this is an example of a case where a serious issue raised got lost in the process of aggregation of public responses.
In one of my comments in the second round of public comments under NEPA, I alerted NASA once more to this omission that the PEIS still has no discussion of the recommendation in the ESF study. I also added that NASA hadn't contacted me via email either to explain the omission.
Robert Walker: They still don’t cite (Ammann et al, 2012) in the draft EIS. Nor do they give any reason for not citing it in the draft EIS or in the materials provided. I can also confirm that they never contacted me in response to my comment though I did provide my email address in the form
(NASA, 2023, Mars Sample Return FINAL PEIS : B-71)
The Council on Environmental Quality advises the public that if our concerns aren’t resolved by the official replies, we need to contact the agency:
Your first line of recourse should be with the individual that the agency has identified as being in charge of this particular process. See if you can sit down with him or her to discuss your concern(s). You may be pleasantly surprised at the response.
(CEQ, 2007, A citizen’s guide to the NEPA: Having your voice heard : 28=9)
I assumed this meant the planetary protection officer or CEO of planetary protection (I now know it means the official point of contact at the head of the PEIS but I doubt that would have made any difference, surely the CEO of planetary protection would have forwarded my email to the correct contact email for the PEIS if necessary).
I did try to contact them NASA of the official replies. During the second round of public comments, I sent an email to Dr. Alvin L. Smith, II, CEO of planetary protection at NASA (NASA, n.d. Contact Planetary Protection Center of Excellence) (after first trying the published email address for NASA's current Planetary Protection Officer, James Bernardini which didn't get through).
Once again I got no reply to my new NEPA comments on the draft PEIS and I got no reply to my attempt to contact NASA's CEO of planetary protection. This is the letter.
I found no other way to contact NASA to ask about planetary protection issues. This is the main contact page for the Planetary Protection section of the Office of Safety and Mission Assurance:
The planetary protection literature stresses the importance of setting up easy dialogue with the public at an early stage
- should have started over a decade ago
- ESSENTIAL FOR SUCCESS OF THIS MISSION.No email addresses given.
NASA currently provide no easy way to contact anyone about planetary protection issues
A search of NASA's website turns up Rob Margetta as Media contact for its Office of Technology, Policy and Strategy.
Screenshot shows the Planetary Protection page of NASA's Office of Safety and Mission Assurance (NASA, n.d. OSMA: Planetary Protection)
Rob Margetta's email address is given elsewhere as the Media contact for NASA's Office of Technology, Policy and Strategy (NASA, n.d. OTPS: News and Resources )
At that stage, after the second round of public comments in December 2022, I had no explanation for why NASA's draft PEIS didn't mention the serious issues I raised in the first round of public comments.
NASA's official reply: not required to speak to commenters directly, only provide public responses to aggregated scoping comments
I only found out why NASA never responded to my email or contacted me about the public comments when it published the final PEIS six months later in June 2023. NASA's official reply is that it isn't required under NEPA to speak to commenters directly.
Robert Walker: I can also confirm that they never contacted me in response to my comment though I did provide my email address in the form
NASA (NP-003): The NEPA process involves a scoping process, where the agency notifies the public of its intent to conduct NEPA on a proposed action and solicit input.
Agencies are not required to provide responses to scoping comments, nor contact and speak with commenters directly; only to summarize the inputs received (if relevant and substantive)
(NASA, 2023, Mars Sample Return FINAL PEIS : B-70, B-71)
In the public comment NASA replied to there, I didn't think to mention the email to it's planetary protection CEO, but this presumably also explains why I got no reply to that email either.
The CEQ says our first line of recourse is with the agency but it just say "see if you can" discuss your concern(s) with the point of contact.
"See if you can sit down with him or her to discuss your concern(s). You may be pleasantly surprised at the response."
(CEQ, 2007, A citizen’s guide to the NEPA: Having your voice heard : 28=9)
It .doesn't say that the agency is required to reply to us. As we see, sadly that wasn't NASA's response. See above:
NASA's response NP-003 shown in the screenshot also says my specific inputs may have been aggregated with the other comments.
NASA: … your specific inputs may have been categorized/grouped with other similar comments.
(NASA, 2023, Mars Sample Return FINAL PEIS : B-70)
But no, my inputs were not considered in the draft PEIS (NASA, 2023, MSR DRAFT PEIS : Table 4.3-1) as I say in the comment they reply to in the final PEIS
Robert Walker: More precisely, in this EIS, they say they read our comments. But in their summary of what they say we said, they don't mention many of the things we raised as issues. NAS can't have given the public comments a thorough examination as required for the EIS process.
(NASA, 2023, Mars Sample Return FINAL PEIS : B-71)
My comments were about the European Science Foundation Mars sample return study from 2012 (Ammann et al., 2012, Mars Sample Return backward contamination–Strategic advice and requirements), which is also never mentioned in the draft PEIS or its references (it isn't mentioned in the references for the final PEIS either).
I asked why they didn't consider the ESF study is the most recent evaluation of the size limit and level of assurance needed for a Mars sample return in the literature. The ESF study itself says that their recommendations led to a major change in containment requirements. It also recommends that its conclusions are reviewed on a regular basis and says it can't rule out the possibility that a future review may once again shatter our understanding of the minimum size of biological entities.
The starting point of this activity was the requirement used since the late 1990s specifying that: … the probability that a single unsterilised particle of 0.2 micron diameter or greater is released into the Earth environment shall be less than [one in a million]
(Ammann et al., 2012, Mars Sample Return backward contamination–Strategic advice and requirements : 3)
As self-replicating free-living organisms are likely to be the main concern following a release event, the study group considers that the release of a particle larger than 0,05 µm [0.05 microns] is not acceptable under any circumstance.
…
Based on our current knowledge and techniques (especially genomics), one can assume that if the expected minimum size for viruses, GTAs [Gene Transfer Agents] or free- living microorganisms decreases in the future, and this is indeed possible, it will be at a slower pace than over the past 15 years. However, no one can disregard the possibility that future discoveries of new agents, entities and mechanisms may shatter our current understanding on minimum size for biological entities. As a consequence, it is recommended that the size requirement as presented above is reviewed and reconsidered on a regular basis.
(Ammann et al., 2012, Mars Sample Return backward contamination–Strategic advice and requirements : 21)
In the final PEIS published in June 2023, NASA finally did give me an official response on this issue of containment for ultramicrobacteria, but only to say they don't concur there is an issue.
NASA: NASA is aware of the ESF Mars Sample Return backward contamination study. NASA does not concur that 0.05-micron (50 nm) particles cannot be managed; standard High Efficiency Particulate Air (HEPA) filters like those used in biosafety facilities are tested for effectiveness at or near the Most Penetrating Particle Size (MPPS), which is typically 0.12 micron …. (Perry et al, 2016, Submicron and nanoparticulate matter removal by HEPA- rated media filters and packed beds of granular materials). “Particles both larger and smaller than the MPPS (including bacterial spores and viruses) are removed with greater efficiency.”
(NASA, 2023. Mars Sample Return final PEIS : B-62)
In my literature searches, I have not found any air filters that can fulfill the ESF recommendations. In this official reply, the cite NASA provide seems to miss the point. It is similar to the other HEPA filter cites I provided for NASA in my public comments. It talks about a most penetrating particle size for HEPA filters. This of course means some particles get through, so HEPA filters with a most penetrating particle size can never satisfy the ESF recommendation of 100% containment at all sizes above 0.05 microns.
All I have so far is a proposal by one astrobiologist to use air incinerators.
I cover this issue in:
- Why NASA can't rely on HEPA filters for the ESF recommendation to contain 100% of ultramicrobacteria
The only reason NASA gives for not mentioning the ESF study or its recommendations is this official reply to me: they don't concur with my comment that these updated requirements could potentially be an issue for HEPA filters.
See:
We see that NASA'S official response to this comment didn't resolve my concerns. Indeed, I don't understand their response, why NASA seem to interpret the cite they provided as demonstrating that HEPA filters can contain 100% of ultramicrobacteria at 0.05 microns and upwards. NASA has provided no opportunity for further dialogue to clear up such communication issues.
This shows there was nobody left on NASA's team familiar with basic ideas of biosafety containment such as how HEPA filters work.
Also the ESF study still isn't given in the list of references for the final PEIS although it is the most recent study of the size limit and level of assurance for containing samples for a Mars sample return mission.
NASA also give no reason for ignoring the recommendation to do another size limit or level of assurance review. Their only response is to say that this discussion is to be deferred to tier II (which is meant for matters not ripe for discussion at tier I).
Robert Walker: The 2012 European Science Foundation study says its 0.05 micron size limit needs to be reviewed regularly … this alone is sufficient reason to halt this EIS process until the new size limits review is done
NASA: [statement already given that they don't concur that this goes beyond the capabilities of a HEPA filter]
…
Requirements associated with the SRF [Sample Receiving Laboratory] (to include management of particle size under the conditions required for sample curation) would be addressed in the Tier II phase of the NEPA process
(NASA, 2023. Mars Sample Return final PEIS : B-62)
For details see:
Since NASA doesn't mention the ESF recommendation for a review of the size limit and level of assurance in its reply to me, or anywhere in the final PEIS, it's impossible to deduce whether NASA's team
- just didn't notice the ESF recommendation or my comment alerting them to it, or alternatively,
- are of the view that HEPA filters are future proof against any future decision about the size limit of particle to contain and the level of assurance required for containment for a Mars sample return mission.
This is one of numerous examples where in their official responses NASA hasn't given adequate responses to the public comments.
Example of another substantial issue in urgent need of public dialogue: limitations of relying on studies for previous biosafety labs for human diseases and animal diseases for samples from Mars
It was a similar situation with the issue of lab leaks and quarantine. NASA are convinced it can handle these with standard biosafety protocols for known infectious diseases, and so, treats them as not ripe for discussion until tier II, when it decides on the site for the Mars Receiving Facility and the details of its design.
NASA’s draft EIS has no mention of quarantine or other precautions for accidental release on Earth even for any technicians that might be accidentally exposed during the landing in the Utah test and Training Range– just sterilization of the landing site.
NASA's one mention of quarantine is a historical mention for Apollo.
The MSR Campaign is the first sample return mission to be classified as Restricted Earth Return, since the term was defined. (The Apollo 11, 12, and 14 missions were subjected to quarantine upon return until lunar samples were assessed and found to pose no hazard.)
(NASA, 2023. Mars Sample Return final PEIS : 3-15)
I raised this issue with them:
Robert Walker: Then they just say (NASA, 2022eis: S-11): … Tier II analyses for determination of impacts associated with health and safety would consider the location of the proposed facility and surrounding community/land use type, health and safety system requirements associated with a BSL-4 equivalent facility, and risk analysis involving failure of containment systems that results in a release.
...The Apollo mission used quarantine of technicians who were exposed to lunar samples in the sample receiving laboratory - and though they mention this they don't discuss it as a possibility for the Mars sample return.
(NASA, 2023. Mars Sample Return final PEIS : B-59)
NASA refer me to its official response to this same question about lab leaks by William Agger who is an infectious disease specialist and clinical microbiologist at Gundersen Lutheran Medical Center (USNews, n.d., Dr. William A. Agger MD)
William Agger: What would society do, for instance, if researchers in a Utah lab or research astronauts in a biosafety 4 lab on the Moon were in a lab accident? What could be done for them and ethically with them?
(NASA, 2023. Mars Sample Return final PEIS : B-30)
It is worth reading the rest of William Agger's comment (which NASA doesn't quote in the final PEIS):
William Agger: In addition, even a level 4 biosafety lab on Earth or even on the Moon, is of great concern to this Infectious Disease/Clinical Microbiologist. Even at the protection level of biosafety 4 labs have not been, nor will be, without accidents. What would society do, for instance, if researchers in a Utah lab or research astronauts in a biosafety 4 lab on the Moon, were in a lab accident?
What could be done for them and ethically with them? Bring them back to earth and/or let them out of the lab, potentially contaminating our biosphere?
In short, it's a scientific gamble with a small upside against a catastrophic downside for the living people on the Earth and to the only biosphere they will survive on. Finally, I am not opposed to looking for life on Mars. If found, it should be left on Mars and studied there.
(Agger, 2022, Comment Submitted by William Agger)
NASA just reply to William Agger that this is for discussion later saying that we have existing protocols for known infectious diseases:
NASA: High-containment laboratories around the world have existing protocols for maintaining staff safety and addressing possible exposures to known infectious substances. The MSR program would develop specific plans and procedures for safe handling of the Mars samples based on these protocols and related lessons learned—including what to do in the case of breach of containment—in close consultation with experts at organizations such as the Centers for Disease Control and the National Institutes of Health. These plans will prioritize staff health and safety, and open communications with local, state, and federal organizations, as well as with the general public.
(NASA, 2023. Mars Sample Return final PEIS : B-30)
The issue with NASA's reply here is the "known infectious substances". Potential alien pathogens are the epitome of unknown never encountered infectious substances. What NASA is missing here is that we can't assume in advance without analysis that existing protocols for known pathogens will also work for unknown pathogens with unknown capabilities and potentially based on an alien biology.
See below:
The only other mention of lab leak is here where NASA argue from previous Environmental Impact Statements for two biosafety labs, one for human and one for animal diseases that this means that public health impacts for a Mars sample return must be negligible.:
An alternative release path resulting from the contamination of workers leading to direct contact with others (members of the public) was also analyzed [Not by NASA]. Qualitative risk assessments for this mode of transmission [for the two previous EIS's for ordinary BSL-4 labs] have shown that the risk to the public [from lab leaks] is negligible (NIH/DHHS 2005, DHS 2008).
Should the Proposed Action be chosen, Tier II NEPA analyses of the proposed SRF11 would include analysis similar to those performed for existing BSL-4 facilities.
(NASA, 2023. Mars Sample Return final PEIS : 3-14)
NIH/DHHS. (2005) = (NIH, 2005. Final Environmental Impact Statement, National Emerging Infectious Diseases Laboratories)
DHS. (2008) = (DHS, 2008. Final Environmental Impact Statement for the National Bio and Agro-Defense Facility with later supplements listed in (NBAF, n.d., Document Library) )
NASA didn't do those analyses. The cites are to previous Environmental Impact Statements, one for animal diseases and one for human diseases.
In my final comment I raised the issue of quarantine of mirror life and fungi.
12. Quarantine of humans can’t keep out a fungal disease of crops, mirror life etc. (Walker, 2022, Comment posted December 20th).
The attachments 7 and 8 go into this in great detail with cites to explain why mirror life is a possibility for life on Mars and how challenging it would be to contain it and the issues with soil pathogens, and Sagan's vexing question, etc., as in this book. For details see below:
- Even the most well prepared labs can have lab leaks - Sagan's insoluble "vexing question" of the quarantine period for a novel alien pathogen
However NASA's team must have prescreened all this material I supplied on issues with quarantine after a lab leak of mirror life and alien fungi as nonsubstantive. It doesn't mention mirror life in any of its quotes from my comments.
This is likely because it decided in advance these matters are not suitable for detailed discussion until tier II once NASA knows where it is going to build the sample receiving facility.
In contrast , Mars sample return studies devote large sections to sample containment in biosafety labs. See below:
NASA's only mention of precautions for lab leaks in the PEIS is here:
An alternative release path resulting from the contamination of workers leading to direct contact with others (members of the public) was also analyzed [Not analyzed by NASA and not analysed for potential alien life]. Qualitative risk assessments for this mode of transmission [for the two previous EIS's for ordinary BSL-4 labs] have shown that the risk to the public [from lab leaks] is negligible (NIH/DHHS 2005, DHS 2008).
(NASA, 2023. Mars Sample Return final PEIS : 3-14)Cites:
NIH/DHHS. (2005) = (NIH, 2005. Final Environmental Impact Statement, National Emerging Infectious Diseases Laboratories)DHS. (2008) = (DHS, 2008. Final Environmental Impact Statement for the National Bio and Agro-Defense Facility with later supplements listed in (NBAF, n.d., Document Library) )
To take an example of the limitations of relying on previous environmental impact statements for issues to do with alien life in their second cite, the National bio- and agro-defense facility (NBAF) facility in appendix D looks at effects of pathogens they study in their labs if t accidentally released in a lab leak. It looks at only three pathogens, all known viruses, foot and mouth disease, Rift Valley fever virus and Nipah virus,
Although the NBAF would study a number of pathogens determined as high priority by the U.S. Department of Agriculture’s Animal and Plant Health Inspection Service nd the Department of Homeland Security, only three pathogens are evaluated for this appendix.
- Foot and mouth disease virus (FMDV)
- Rift Valley fever virus (RVFV)
- Nipah virus (NiV)
The diseases caused by these three pathogens sufficiently cover the spectrum of outcomes likely to occur if
any of the pathogens to be studied at the proposed NBAF were to release to the surrounding areas and infect
animal and human populations.,(DHS, 2008, Appendix D: Potential economic consequences from the proposed NBAF : D-2)
The NBAF knows in advance the types of pathogens it will study in this biosafety level 4 facility, and so can pick representative examples. It has experience of outbreaks of all three of its representative examples, and knows the transmissibility, and virulence and potential economic effects of each one. But these three examples are all viruses adapted to terrestrial animals over millions of years of evolution and are of no interest to a Mars sample return mission
This appendix is not going to inform NASA about the potential economic effects of accidental release of alien soil pathogens and mirror life.
Similarly if we look at its first cite, it lists precautions to prevent harm to human health in event of a lab leak, vaccines and therapeutics.
The potential to acquire a laboratory-caused disease is further reduced through the use of effective vaccines or therapeutic measures (CDC & NIH 2007).
,(DHS, 2008, Appendix E: Accidents methodology : E-66)
There won't be vaccines for alien microbes, and it may take a long time to develop an effective vaccine if it is possible at all. There may be no therapeutic drugs either. For instance an alien fungus may be naturally resistant to all our antifungals. See Supplementary information under:
This book as a way forward to encourage peer review of safety plans for Earth's biosphere and to encourage NASA to move to a more open approach and facilitate public dialogue as recommended by all the Planetary Protection studies
The upshot is I and others who commented under NEPA had no opportunity for further dialogue with NASA's team if our concerns weren’t resolved, or were lost, or they quoted the comment but didn't seem to notice what it said.
Another major surprise for me from the draft PEIS is that NASA relies on material over a decade old for such topics as in situ life detection, acceptable levels of contamination in the Mars samples and so on. NASA doesn't seem to have attempted a literature survey of the topic for newer material. Also, there is no mention of any proposal for a new study of planetary protection for a Mars sample return mission in the near future, although the last comprehensive study was 14 years ago.
We can see the effects of a lack of even an updated literature survey, throughout the planetary protection sections of the PEIS.
When I received no response from NASA after the second round of public comments, I started work on a new paper, and rapidly decided that whatever I might do next, it is a top priority to do a preliminary literature survey on topics relevant to planetary protection for a Mars sample return mission. This survey took the material I found for my original preprint as a starting point but was more thorough.
It would be far beyond the capability of a single independent researcher to do a new comprehensive study on planetary protection for a Mars sample return mission. A literature review needs to be systematic and would also be a long job for such a multidisciplinary subject, not far off the complexity of climate change in the range of topics that would need to be covered. For my preliminary literature survey, the aim is simply to include some of the more significant recent developments in the most important topic areas relevant to planetary protection for a Mars sample return.
The main motivation was that there have been numerous new developments in that time period. Experts on martian climate and the potential for liquid water on Mars, might not necessarily be familiar with the latest research on air filter technology or fungal pathogens of microalgae, and so on. It’s as comprehensive as I could make it in the time available with the resources I had. Since I didn't set out to do a literature survey originally it's intermingled with other material. Perhaps it could be characterized as a literature survey organized as part of a response to NASA's plans. Still it may well be useful to other researchers and not just myself. I used it as a basis for this book and it has a lot of material that expands further on some of the topics covered in this book.
The range of topics relevant to planetary protection for a Mars sample return mission is so vast, and there has been so much new research every year on those topics since 2009, that even with this limited scope, the literature survey took me about 5 months working half time.
Based on that I then drafted (but didn't send) an open letter to NASA hoping it might get more attention than my comments and my previous email to their office. This took a few more weeks of work. However, a week or two before I was ready to send the open letter to them, NASA published the final PEIS. The draft open letter is here (Robert Walker, 2023, Open letter to NASA)
When I read the final PEIS and NASA's responses to the public comments it became clear an open letter wouldn't work. On a first glance at the PEIS it was already clear from the responses on HEPA filers, risk assurance, lab leaks and so on that there is nobody in NASA's team that could respond to an open letter like that. Also it seems unlikely there is anyone in NASA with the executive power to make the decisions that would be needed to withdraw the final PEIS and restart it, taking account of the serious issues raised, which is what I ask them to do in the open letter.
It became clear that a new approach is needed.
If our concerns can't be met by dialogue with the agency, the only other option provided legally seems to be a legal challenge.
If NASA's PEIS was ever taken to court, it seems certain they would lose the case. The PEIS fails basic NEPA requirements on multiple counts, such as the requirements for scientific integrity, the issues with prescreening and many other topics. These are exceptionally clear cases too, not gray area issues that one would need to consult a NEPA lawyer about. For an overview of the NEPA issues I identified see Supplementary information under:
There are organizations in the USA which have the funding and possibly the interest in taking out such a case such as the Audubon society, for the potential effects on wild birds.
However any such organization would have to do it in collaboration with someone who has legal "standing". There are two requirements for that. They must have commented under NEPA raising the issues during the official period for comments. They also must show that they are personally potentially affected by the outcome.
From similar legal cases under NEPA, since NASA would lose the case if it got to the courts, NASA's most likely legal response would seem to be to argue that everyone on Earth would be equally affected by any adverse effects, and so, that nobody individually is affected more than others, so nobody has standing to take out a case. Environmental cases under NEPA based on similar arguments have gone either way in the past. Some succeed, some fail.
In the view of the author, the failed NEPA requirements are there for a reason and should be respected.
Taking NASA to the courts doesn't seem a good way to achieve this however. Whether the legal case succeeded or failed, this legal case risks triggering the very polarization between the public and NASA that I believe we need to avoid. This in turn has a risk of leading eventually to cancellation of the mission. Also the justice decides on the remedy and often the remedy is to stop the project. Either way it could cause problems for attempts to redo the plans on sound planetary protection principles.
My aim is for NASA to do the PEIS again following the NEPA recommendations properly, not to cancel the mission or cause problems for NASA.
For details see:
So I decided that the legal route is ruled out.
Before I read the PEIS in detail I had the idea of asking various experts on topics relevant to the PEIS for endorsements of various statements such as that a HEPA filter can't contain ultramicrobacteria, or that NASA's Mars meteorite argument (NASA, 2023. Mars Sample Return final PEIS : 3–3) is rebutted on page 5 of their main National Academy of Sciences cite (SSB, 2019, Planetary protection classification of sample return missions from the martian moons : 5), and signatures for a letter to NASA. However, that was an unusual approach for a topic that would normally be sorted out by peer review. Also on reflection, there seems unlikely to be anyone at NASA with the authority to withdraw the final PEIS at this stage, which is what the letter requested. It soon became clear that endorsements were not a viable way ahead either.
That then led to the idea for this book, using as its basis the material I prepared for the requests for endorsements, open letter and the literature survey together with the earlier preprints.
My main focus now is on peer review for both my own work and to encourage independent review of NASA's biosafety plans by appropriate organizations.
For peer review of my own work, I plan to submit this book to an academic paper and / or submit papers for publication based on this book.
I also encourage review of NASA's plans by an independent body in high standing such as the National Academy of Sciences / Space Studies Board in the USA, or the European Science Foundation, which could presumably be requested at any stage by NASA, or ESA. Also, as we'll see, the leader of NASA could request it under NSC-25 with the review organized by the chair of the Council of Environmental Quality.
In one of my comments on the draft PEIS that NASA published in the final PEIS, I said the public deserve peer review of NASA's plans.
Robert Walker: NASA show no indication of having read the first sentence of my comment [about the ESF requirements that go beyond HEPA filters].
… More precisely, in this EIS, they say they read our comments. But in their summary of what they say we said, they don’t mention many of the things we raised as issues.The public deserves it to be peer reviewed first by independent reviewers not connected with the project.
(NASA, 2023, Mars Sample Return FINAL PEIS : B-71)
NASA's response was they are not required under NEPA to do peer review. They said that these comments and their replies to them are all that is required under NEPA, and that they substitute for conventional peer review.
NASA: NEPA does not require a "peer" review prior to release. The purpose of releasing the Draft PEIS is to allow the public, agencies, and other interested parties to review the document
(NASA, 2023, Mars Sample Return FINAL PEIS : B-71)
It is true NEPA doesn't require peer review of the plans. However it does require scientific integrity § 1502.23.
The easiest way to establish the scientific integrity of NASA's novel reasoning is to get it peer reviewed.
See:
NASA's novel reasoning would be one of the top stories in planetary protection of the decade, if it passed peer review. It would overturn two decades of research on the topic.
Given the high level of attention for a Mars sample return mission, it's not credible that NASA's plan can survive through to the 2030s when the samples are returned without peer review.
NASA's first planetary protection officer John Rummel wrote in 2002:
Reviews of the Draft Protocol [for receiving and testing Mars samples] should provide for the highest degree of scientific scrutiny and evaluation The evaluation should be conducted jointly by scientific organizations from both the United States and France (and other countries, as appropriate) to avoid prolonged negotiations and resolutions that may arise when such reviews are conducted separately.
This review should probably occur at the level of the National Research Council in the United States, and its equivalent scientific organization in France, whichever is most appropriate
(Rummel et al., 2002, A draft test protocol for detecting possible biohazards in martian samples returned to Earth : 98)
Also as recently as 2019 the Space Studies Board recommended NASA set up an independent advisory body to act as external peer reviewers for NASA's plans (amongst other tasks):
Act as a peer review forum to facilitate the effectiveness of NASA’s planetary protection activities.
(SSB, 2018, Review and Assessment of Planetary Protection … : 62)
This wasn't done.
In addition to its novel findings and reasoning, NASA also isn't doing probabilistic risk assurance as it is normally understood, something NASA are usually good at. Its approach is novel.
Normal risk assurance starts with a target probability, often one in a million, though it can vary from 1 in 10,000 to less than 1 in a million depending on the level of assurance that seems necessary for a particular risk. This is an ethical rather than an engineering decision. However once the target is decided, the rest is a matter that can be studied using the methods of science and engineering.
However in place of this approach, NASA uses very novel reasoning that bypasses the approach of risk assurance altogether.
NASA told me that based on an assessment that significant ecological impacts are unlikely they can deduce that any environmental effects would not be significant. This novel reasoning is logically invalid. It is like concluding that because the risk of a significant house fire is unlikely, any house fire would not be significant.
Chester Everline, a co-author of NASA's handbook on probabilistic risk assurance (NASA, 2011, Probabilistic risk assessment procedures guide for NASA managers and practitioners) unusually commented on the last day of public comments (about the same time as my last comment). He found the PEIS didn’t state clearly what level of risk NASA is prepared to take for Earth’s biosphere and raised many other issues from the perspective of risk assurance.
NASA replied saying that they don't have a campaign level target probability and aren't required to have one. See below:
Chester Everline recommended that if NASA can't establish the Mars meteorite argument, it should consider a deferred return until it has the information needed to assess risks better.
NASA didn't mention his proposed alternative in the PEIS. In their official reply to his public comment they told him it was already covered by the "no-action" alternative (which doesn't mention any suggestion of a deferred return). See below:
This shows there was nobody left on NASA's team familiar with risk assurance as it is normally done, even though this is a topic where NASA has in-house excellence.
This novel approach to risk assurance is another of numerous matters that could be reviewed by an independent body.
Many issues of scientific integrity and bioethics would be raised with peer review
As soon as they get peer review from a peer such as the Space Studies Board or the European Science Foundation any issues of scientific integrity will be discovered.
If the Mars meteorite argument doesn't survive peer review, NASA will be required to achieve a higher level of biosafety assurance to handle the potential for large-scale effects.
The simplest way such a review could happen would be under NSC-25. According to this presidential directive, the leader of an agency is required to tell the director of the Office of Science and Technology Policy if the experiment they are planning could lead to allegations that they might have protracted or large-scale biological effects (amongst other reasons). This can lead to the chair of the CEQ ordering a review of the proposal.
On the face of it NASA's biosafety plan for its Mars sample return mission seems a particularly clear case for potential allegations of prolonged or large scale biological effects under NSC-25. That's because NASA themselves imply in their official response in the PEIS that there is a non zero risk that one outcome of their experiment could be that humans might have to live in biomes for the rest of time, or that all life on Earth might be made extinct.
Greger: Are you certain that in any way, this mission won’t end with the total annihilation of the entire planet, or force us to live in biomes for the rest of time?
NASA: As discussed in Section 3.2 of the PEIS, the exact nature of the Mars sample constituents regarding biosignatures and potential biological activity is currently unknown.
The PEIS cites several sources supporting the position that contamination of Earth by Martian microorganisms is extremely unlikely to pose a risk of significant harmful effects. However, the risk cannot be demonstrated to be zero (see Response ID HS-001 for information regarding containment measures).
[NASA go on to say that with our current state of knowledge it is impossible to do a comprehensive analysis and continue to say: ] any such analysis would be theoretical at best, involving substantial speculation and supposition. For this reason, the emphasis of the MSR approach is on sample containment(NASA, 2023. Mars Sample Return final PEIS : B-43)
In total, ten out of 63 public comments specifically raised issues of unprecedented harm. NASA prescreened most of those as nonsubstantive. But they did quote Spotts in the PEIS
Spotts: What happens if a Mars life form escapes containment and, without evolving in Earth's ecosystems, spreads uncontrollably and devastates Earth's species including us humans? There might be no way to reverse or even mitigate for that devastation.
NASA just refer him to their response to Greger HS-002
(NASA, 2023. Mars Sample Return final PEIS : B-51)
This is Richard Spotts' public comment in full:
Richard Spotts: I am extremely concerned that this proposed action could potentially contaminate native life forms on Mars and/or bring back alien virus, bacteria, or other life forms from Mars to Earth.
I understand that there are planetary protection protocols. However, Murphy's Law says that if something horrible could happen, it eventually may indeed occur. History is filled with examples where Acts of God and/or human arrogance caused otherwise unforeseen disasters.…
The Earth is already dealing with increasingly serious problems from invasive or alien species being transported to new locations, and viruses mutating and causing deadly pandemics. We have not been able to solve many of these problems.
What happens if a Mars life form escapes containment and, without evolving in Earth's ecosystems, spreads uncontrollably and devastates Earth's species including us humans? There might be no way to reverse or even mitigate for that devastation.
I support scientific research when it is safe and in the public interest. However, I oppose research when there is no absolute guarantee of safety and when the risks outweigh the potential benefits.
(Spotts, 2022, Comment Submitted by Richard Spotts)
The other comments raising similar points which NASA prescreened as nonsubstantive include Thomas Dehel, who quoted from an interview he did with Gill Levin, principal investigator for the first and only direct life detection experiment sent to Mars, who died shortly before the start of the PEIS process
"I believe people will realize, especially after the Covid-19 catastrophe, that even if there’s only a small chance that something could be contagious and pathogenic, coming from a foreign planet, I don’t think it’s worth taking that chance….you don’t take unnecessary chances where the risk-to-benefit ratio is almost infinite.”
(Dehel, 2022, Comment posted December 13th)
Also, Barry DiGregorio who quoted from an interview he did with Carl Woese when he was alive. Carl Woese is the biologist who used gene sequencing to identify the archaea, the third realm of life. As the botanist Otto Kandler put it: “He opened a door which nobody expected to exist” (Sapp et al., 2013, The singular quest for a universal tree of life).
“When the entire biosphere hangs in the balance, it is adventuristic to the extreme to bring Martian life here. Sure, there is a chance it would do no harm; but that is not the point. Unless you can rule out the chance that it might do harm, you should not embark on such a course”
(DiGregorio, 2022, Comment posted December 5th)
Then myself, quoting Carl Sagan in both my attachment 7 and attachment 8 (Walker, 2022, Comment posted December 20th)..
“The likelihood that such pathogens exist is probably small, but we cannot take even a small risk with a billion lives.”
(Sagan, 1973, The Cosmic Connection – an Extraterrestrial Perspective)
This means NASA prescreened Carl Sagan's quote as nonsubstantive too.
The other four comments raising issues of unprecedented harm which NASA prescreened as nonsubstantive were these (direct links to the comments with brief summary):
- test first, unprecedented harm [Evelyn B] - stop mission, unprecedented harm [Gabor Bihari] - unprecedented harm, test first [Emily Whitehead] – unprecedented harm, return to space station [Paul Marks]
This does NOT mean there is any active suppression of the comments going on here. All this is easily explained as due to the difference between an engineer's and an ethicist's mindset / focus. For an engineer matters to do with ethics are likely to be seen as nonsubstantive as they are not directly related to the engineering requirements of the mission. These would seem to be mere opinion. But for an ethicist these are the most substantive of the public comments received on the draft PEIS.
This shows there were no ethicists on NASA's team.
In total 49 out of 63 made comments make it clear they would agree with Sagan’s view and of those, those nine commenters specifically mention unprecedented harm. Chester Everline also specifically mentioned unprecedented harm but didn't present a personal view of what would count as an acceptable level of risk for the mission.
See:
It's not possible to convert this to a percentage as it wasn't a poll. But we do see that more than four dozen people to raise the issue in a not very well publicized PEIS, ten specifically raised issues of unprecedented harm and those ten include quotes from three well known scientists (sadly all deceased) with all the quotes from well known scientists prescreened as nonsubstantive.
I think it is reasonable to conclude that this will be a major concern for many members of the public as well as many scientists too, once it gets wider attention. This concern has been averted so far only because of the low key way NASA publicised its plans, with its novel biosafety reasoning.
However as we saw, NASA say in the main document that any environmental effects would not be significant, and any public health effects negligible. From what NASA say in the PEIS itself, about NSC-25 where it is only mentioned in one sentence in passing (NASA, 2023. Mars Sample Return final PEIS : 1-5), it seems unlikely that they intend to raise it as an issue in this way.
This may be based on their novel deduction which we saw above:
NASA might use this reasoning to deduce that NSC-25 doesn't apply to the Mars sample return mission. However it is this very deduction that is one of the main points that needs to be reviewed by the National Academy of Sciences, if it is referred under NSC-25.
The leader of NASA has to signal to the director of the OSTP that a review is needed. If this doesn't happen, NSC-25 doesn't give a way for the general public to raise this issue.
However, I plan to check to see if NASA do plan to raise this matter, and update this section accordingly depending on what I find out. If NASA's plans are reviewed under NSC-25, the Space Studies Board is the likely body to be asked to do the review, with the National Academy of Sciences, and any such review would surely discover the same issues I identified in this manuscript. See:
So there may be potential for NASA's plans to be reviewed under NSC-25. However, at present we can't count on that.
Another possibility is that ESA might ask the European Science Foundation to review the plans. However at present this seems unlikely. See below:
Need to overcome communication barriers to get the public and scientists generally involved in dialogue on risk governance for the safety of Earth's biosphere
My experience, and that of others I have contacted, is that at present it is virtually impossible for the general public to engage with anyone connected to NASA or able to talk to NASA on this topic.
All this would have been far simpler if NASA had a policy of encouraging the public to get involved in risk governance, as recommended by the ESF in 2012 in a report co-authored by NASA's first planetary protection officer John Rummel (Ammann et al., 2012, Mars Sample Return backward contamination–Strategic advice and requirements : 59)
However, the experiences described here show the opposite. NASA doesn't seem to provide an avenue for public engagement in risk governance, except as legally required under NEPA via scoping comments.
I contacted over a dozen experts but found hardly anyone was available to discuss these issues.
Given the circumstances, that NASA's current planetary protection officer or CEO of planetary protection at NASA are not available for dialogue, it's perhaps not surprising I also can't discuss serious issues with NASA's plans with NASA's employee Chester Everline (who raised issues with how risk assurance is done in the PEIS under NEPA). I also can't discuss them with NASA's first two planetary protection officers and the former ESA planetary protection officer (all published authors on planetary protection for a Mars sample return mission).
All this applies more generally to experts in other topics related to a Mars sample return mission such as public health, early life, etc. A few encouraged me to continue what I'm doing.
Hardly anyone expert on topics related to a Mars sample return mission was available to talk about the serious issues I raised. The only exception so far is one astrobiologist. He didn't have much time for discussion, but did reply.
This astrobiologist suggested adding an air incinerator to a HEPA filter to contain ultramicrobacteria. I discuss this idea and pros and cons later in the book. For instance it would need to contain 100% of ultramicrobacteria even if protected in a crack in a dust grain and with microbes that may use PNA which is more heat resilient than DNA, and may have spores with extra layers of protection from UV and protection from superoxidants for transport in martian dust storms. It seems likely to need new methods developed to test the air incinerator and to ensure that no nanoparticles escape if the incinerator fails for some reason or when the HEPA filter is changed.
See : Air incinerators are not certified to contain alien ultramicrobacteria yet - could they be a solution?
Then there is still the issue of lab leaks and quarantine. In any human run lab however good the containment method we need precautions in place for lab leaks in case the method fails which leads to the quarantine issue. The air incinerator may be part of a possible solution. I'm interested to discuss these issues and possible solutions with anyone and will update the book accordingly if there are other ideas suggested that are worth considering. Maybe I'll get more feedback as part of the peer review process.
A small amount of discussion may be possible if one is lucky. However, for most in the public, such as nearly all of those who added public comments, there is nobody they can go to for further dialogue, or indeed reassurance, if they find NASA's response to them unsatisfactory. Also since NASA didn't notify any of the commenters about their replies to their comments in the final PEIS, it is likely that many who commented under NEPA are unaware that NASA did reply.
This communication barrier is something that would need to be researched by experts in sociology. Whatever the reasons for this, it seems to be barely possible for the general public, or even an independent researcher working on a paper on planetary protection for NASA's mission to enter into dialogue with experts on potential serious issues with NASA's biosafety plans. I have also found no organization, forum, individual or method of communication set up to facilitate dialogue with the general public on risk governance for NASA's biosafety plans.
From the Mars sample return studies, the sooner any issues with NASA's biosafety plans are widely aired the better. NASA's Mars sample return project started in 2011:
NASA and the European Space Agency are collaborating on proposals for a mission to gather samples of Martian rocks and bring them to Earth after 2020.
(NASA, 2011, Martian Samples Leaving Mars)
That is when work should have started on facilitating the public discussion according to the Mars sample return studies:
RECOMMENDATION 3
Potential risks from an MSR are characterised by their complexity, uncertainty and ambiguity, as defined by the International Risk Governance Committee’s risk governance framework. As a consequence, civil society, the key stakeholders, the scientific community and relevant agencies’ staff should be involved in the process of risk governance as soon as possible.
(Ammann et al., 2012, Mars Sample Return backward contamination–Strategic advice and requirements : 59)
[bolding and red text added to highlight relevant text]
The Risk Perception Workshop published a consensus statement which was included in the ESF report along with those recommendations. Some of the relevant points include:
- The ‘trust me I am a scientist’ approach cannot be considered valid when considering potential release of Mars organisms. The uncertainties have to be clearly listed and explained;
- Structured and targeted communication throughout the whole process is crucial to gain trust.
- Mars research may yield beneficial outcomes that range from useful to tremendous; not undertaking the MSR mission may result in lost opportunities.
- Cultural differences in risk perception and acceptability are real.
- Reversibility of the effect is an important characteristic to consider.
- Risk is an element that does not stand alone. It has to be considered together with risk management and risk communication approaches and strategies, as well as potential benefits.
(Ammann et al., 2012, Mars Sample Return backward contamination–Strategic advice and requirements : 58)
[bolding and red text added to highlight relevant text]
ESA's former planetary protection officer Gerhard Kminek is one of the signatories of that consensus statement and NASA's first planetary protection officer, John Rummel, is one of the authors of the ESF report it is contained in.
The 2009 Mars sample return study put it like this:
Experience with past and present Mars missions, and with the recent Genesis and Stardust sample return missions, indicates there will be keen public interest in any program to return martian samples to Earth. In particular, it should be recognized that such a mission is likely to face intense scrutiny regarding the potential risks associated with handling in an SRF [Sample Return Facility] on Earth pristine martian materials that could potentially contain extraterrestrial life forms .... All of these concerns necessitate that the public should be openly informed of planning for both a sample return mission and the construction, testing and operation of an SRF.
(SSB, 2009, Assessment of planetary protection requirements for Mars sample return missions : 68)
John Rummel put it like this in his earlier paper from 2002:
John Rummel: As part of sample return planning, it will be important to develop an organized communication plan which will lay a strong foundation in public understanding and acceptance prior to the mission, and allow for an open dialogue with all sectors of the public.
Such a plan should include consideration of the diverse questions, concerns, and issues likely to be raised, including those related to the mission and spacecraft operations, the sample return and Biohazard testing, the administrative and legal matters associated with the effort, and to the potential implications of discovering extraterrestrial life. Plans should be developed well in advance in order to avoid a frenzied, reactive mode of communications between government officials, the scientific community, the mass media, and the public.
Any plan that is developed should avoid a NASA-centric focus by including linkages with other government agencies, international partners, and external organizations, as appropriate. It will also be advisable to anticipate the kinds of questions the public might ask, and to disclose information early and often to address their concerns, whether scientific or non-scientific.
(Rummel et al., 2002, A draft test protocol for detecting possible biohazards in martian samples returned to Earth : 94)
…
Evaluations of the proposal should be conducted both internal and external to NASA and Centre National d'Etudes Spatiale (CNES) and the space research communities in the nations participating in the mission. An ethical review should be conducted at least at the level of the Agencies participating and these reviews made public early in the processThe final protocol should be announced broadly to the scientific community with a request for comments and input from scientific societies and other interested organizations
“Broad acceptance at both lay public and scientific levels is essential to the overall success of this research effort.”
(Rummel et al., 2002, A draft test protocol for detecting possible biohazards in martian samples returned to Earth : 99)
Unusual or unprecedented scientific activities are often subject to extreme scrutiny at both the scientific and political levels. Therefore, a communication plan must be developed as early as possible to ensure timely, and accurate dissemination of information to the public about the sample return mission, and to address concerns and perceptions about associated risks.The communication plan should be pro-active and designed in a manner that allows the public and stakeholders to participate in an open, honest dialogue about all phases of the mission with NASA, policy makers, and international partners. Risk management and planetary protection information should be balanced with education/outreach from the scientific perspective about the anticipated benefits and uncertainties associated with Mars exploration and sample return.
(Rummel et al., 2002, A draft test protocol for detecting possible biohazards in martian samples returned to Earth : 101)
[bolding and red text added to highlight relevant text]
For details see:
But this is for NASA and ESA to do. Researchers can't achieve this by writing papers recommending such a policy.
Since then, all we have had by way of opportunities for public involvement in risk governance seems to be the two rounds of legally required NEPA comments on NASA's PEIS in 2022. This opportunity was available for two short windows of time, for 30 days from April 15 to May 15 (NASA, 2022, National Environmental Policy Act; Mars Sample Return Campaign (first round)), and for 45 days from November 4 to December 19 (NASA, 2022, National Environmental Policy Act; Mars Sample Return Campaign (second round)). Both opportunities were only publicised in local newspapers near the Utah Test and Training Range in the USA and the Kennedy Space Center (NASA, 2023. Mars Sample Return final PEIS : 4-1). Also as we see here, though NASA gave the public an opportunity to comment on its plans during these brief windows, they presented no opportunity for real dialogue.
As I described in the pitfalls section of this book, this ultra low key approach to discussing NASA's biosafety plan for Earth's biosphere can't continue in this way through to the 2030s in our modern world.
Establishing open dialog with the public seems as essential to this mission as the fuel budget for returning the samples from Mars.
But so far this topic is flying under the radar of most people and not getting much attention.
This leaves submitting papers for peer review as the way forward. I hope by submitting this book or papers based on it for peer review this can become once more a topic that is open to discussion with the general public.
I also remain hopeful somebody at NASA will listen to the concerns of myself and other commenters at some point. I am hopeful that at some point NASA will work to establish dialog with the general public on risk governance for their mission, as recommended in all the Mars sample return studies (Ammann et al., 2012, Mars Sample Return backward contamination–Strategic advice and requirements : 59). Perhaps NASA will need an external independent review of its plans before this happens.
I hope this description of issues I found trying to discuss the topic with anyone may encourage experts and space agencies to give careful consideration on ways to integrate dialog with the general public into risk governance for a Mars sample return mission. It seems to be important to actively encourage participation of experts of many disciplines in public discussions of NASA's plans both with each other and with the general public. There seem to be considerable barriers to be identified and removed to achieve the open dialogue with the public recommended in the Mars sample return studies.
With our modern internet connected world there are many new tools that could be investigated to help overcome these barriers. The WHO added to the approaches available with many innovative tools during the COVID pandemic that could help here. This could include
- fora set up at an international level and open to representatives of all countries, dedicated to the ethical and social aspects of risks and benefits of the mission
(Ammann et al., 2012, Mars Sample Return backward contamination–Strategic advice and requirements : 59) - "ask NASA/ ESA" pages where the public post questions, experts from space agencies respond and open comment and dialogue on the responses are encouraged (similar to the scoping comments but with no end date to submit the comments and with open dialogue and follow up questions encouraged)
- Q/A pages hosted by the space agencies that answer common questions from the public (again similar to the scoping comments), similar to the WHO many COVID-19 Q/A pages (WHO, n.d., Q&As on COVID-19 and related health topics)
- live Q/As similar to the WHO live Q/As announced in advance where the public use hashtags or comments on the streaming video to ask questions as the Q/A proceeds, with their ASKWHO series. (WHO, n.d., COVID-19 Q&A #AskWHO live series)
- Short videos on essential topics similar to the WHO Science in 5 (five minute summaries of the science). (WHO, n.d., COVID-19 Q&A #AskWHO live series)
- Chatbots to respond to questions globally in the user's native language - this can follow the model of the WHO question / answer chatbot established on social media early in the COVID pandemic giving answers to common questions about COVID in the user's own language, and directing the enquirer to appropriate
resources
(WHO, 2022, Chatbots against COVID-19: Using chatbots to answer questions on COVID-19 in the user’s language) - Public debate of any major ethical issues, which can't be resolved by scientists or engineers, for instance about the acceptable level of risk for large scale effects
[Because this mission may have an ethical dimension regarding accepting risk, there may be a diversity of views that can't be resolved using the methods of science, and then debate is appropriate as a way to air the differences, as a way to then work towards a consensus on what to do about it.] - and so on
However so far, none of this has been done.
How NASA's current approach of closing down dialogue with the public, scientists, planetary protection officers, and other agencies, though motivated by a wish to send humans to Mars quickly, may have the opposite effect
NASA's motivation for its change in approach to planetary protection based on its four novel findings seems to be to prepare the way for humans to go to Mars as fast as possible as we saw.
This isn’t the Planetary Protection of the past — we are doing things differently. …There’s still a lot of work to go as we start to pave the way to humans on Mars … we need that collective agency support to do that.
(NASA, 2023, SMA Leadership Profile: Nick Benardini)
From this quote, it is clear NASA's underlying motive is to make it easier to send humans to Mars faster. Its approach is to take the lead and expect other agencies to follow.
What is less clear is why NASA thought a new approach to planetary protection is needed for a Mars sample return mission and how it would facilitate that end goal, As for the specific changes in NASA's policy, James Bernardini has explained further in a paper published September 2023. It is to prepare for a future with human missions which can't be done with the same level of planetary protection as robotic missions. So the idea seems to be that this is a transitional stage with less planetary protection than before to prepare to a future with minimal planetary protection for human missions.
NASA's planetary protection policy is shifting from a prescriptive-based approach to a performance-based approach. The prescriptive-based approach defines both the detailed requirement (the what) along with the implementation approach to meet the requirement (the how) whereas the performance-based requirements approach defines the high-level requirement objective allowing maximum flexibility of the end user to establish the implementation approach.
…
While the current planetary protection risk posture for robotic missions is to proceed forward with a conservative approach to address harmful contamination and biological safety risks, it is recognized that we cannot leverage this same risk posture for crewed missions. Crewed missions are proceeding forward with a risk posture that we must control microbial contamination whilst preventing harmful contamination.
(Bernardini, 2023, Planetary Protection Policy and Technology Needs to Enable Future Robotic and Crewed Missions )
However, from the evidence of the PEIS itself, this new approach seems to involve
- Reducing engagement with the public and scientists and other experts outside NASA to a minimum
- Using reasoning that lacks scientific integrity and sometimes is logically invalid
- Removing external peer review (which would have picked up these issues at an early stage) except when legally required
- Abandoning the previous approach of regular reviews of the literature to update planetary protection requirements, size limit and level of assurance
- A belief based rather than evidence based approach to mission planning.
For the last of those, NASA's plans are based on an an intermediate goal of humans on the surface of Mars At this stage of our understanding of Mars' biosphere, if it has one, this intermediate goal is belief rather than evidence based, because we don't yet have evidence that it is biologically safe to land humans on Mars.
NASA might have made these changes in its policy prior to a Mars sample return mission out of a perception that it is urgent to return unsterilized samples to terrestrial labs. That might be a motivation to do this even before the risks are well understood, out of a belief based confidence that no risks will be found, combined with a wish to send humans to Mars quickly.
There are some reasons to suppose this could be NASA's motivation. If this is NASA's motivation, it is based on out of date material, and it could be another example of the drawbacks of relying on literature one to two decades old, and not doing a new literature survey.
NASA;s Mars Sample Return mission was originally approved with Safe on Mars (SSB, 2002, Safe on Mars: Precursor measurements necessary to support human operations on the Martian surface : chapter 5 : 38), a study from 2002, as the main motivation for the mission (Space Studies Board and National Research Council, 2012. Vision and voyages for planetary science in the decade 2013-2022 : 17)., It may be that NASA are still of the view that the approach outlined in Safe on Mars two decades ago is still a valid way to establish that it is safe to send humans to Mars.
Back in 2002 many experts thought we just need to return a sample to confirm Mars is sterile, then send humans in a follow on mission immediately. For why this might be NASA's motive and why we can no longer do it like this with our modern more complex understanding of Mars, see below:
With our modern more complex understanding of Mars we need far more than a few sample returns. For instance there could be low levels of present day life in the dust, or in microhabitats in Jezero crater that may take a fair bit of investigation to find. See:
through to
It may be useful to know NASA's reasons in detail for its change of policy closer scrutiny to see if NASA's plans can be achieved as well or better in other ways that may be safer for Earth. As already mentioned, I suggest in this book that their end goal of humans throughout the solar system may be better achieved, faster, and with less risk of stranded assets, with Mars’ innermost moon Phobos as a "Gateway" set up first from which we decide whether or not it is biologically safe for humans to land on Mars.
See:
Although motivated by a wish to send humans to Mars as soon as possible, NASA's new approach to planetary protection may well have the opposite effect. By reducing engagement with the public and scientists and other experts outside NASA to a minimum NASA has set up conditions that if not changed, could lead to a collision between NASA's objectives and general public, scientific and expert opinion including opinions of public health experts once the plans get intense scrutiny.
This could lead to deferral of the sample return until we understand the risks better, the approach recommended by Chester Everline.
This in turn could lead to deferral of NASA's plans to send astronauts to Mars since it will be clear to the public and to scientists and other experts outside NASA that if we don't have a biosafety plan to return half a kilogram of rock from Jezero crater we can't be in a position to safely return astronauts from Mars. NASA would then need to put in place a new approach to investigate biosafety of Mars for astronauts. This would be harder to do if NASA's scientific integrity is under question by then because of the issues with its biosafety plans.
How movement in the direction of more public engagement might accelerate NASA's goals for humans in space and lead to more realistic evidence based intermediate objectives
NASA's new approach to planetary protection may need closer examination in view of the effects it had on the PEIS and the reasoning used in the PEIS. Perhaps we need to move in the opposite direction of more public and expert and scientific engagement, more peer review, and greater scientific integrity for success of these missions. Just as movement in the direction of less engagement and less scientific integrity may lead to deferral of NASA's goals, movement in the opposite direction may help to accelerate NASA's end goals. Perhaps, paradoxically as it may seem at first, NASA's end goals of humans throughout the solar system could be achieved faster with more rigorous planetary protection at this stage.
Our new understanding of the complexity of Martian microenvironments and conditions, and of the potential interconnectedness through the dust storms leads to a similar assessment of habitability as for the naive vision of Mars of the 1970s. Sagan said we need to do a vigorous program of unmanned exobiology before we can determine if it is indeed biologically safe to send humans to the surface of Mars and the same applies today. But today we can do it with 100% sterile landers. See:
I'm as keen as anyone for astronauts in space :). But we do need to be safe too, I hope NASA agree, especially when Earth's biosphere is potentially at risk in the worst case. So it does seem necessary for such a novel approach to be reviewed.
The main thesis of this book is that we can do both, keep Earth safe and prepare for future human space exploration too in a better mission with more science return, as covered in this book under:
Our intermediate goal can be a Phobos gateway in orbit around Mars similar to the lunar gateway for the Moon. See the sections:
through to
At the start of the 21st century NASA was a world leader in planetary protection. Its Viking mission in the 1970s remains the gold standard for planetary protection for Mars, never duplicated by itself or any other agency. Its planetary protection office which it funded for 20 years from 1997 to 2017 was responsible in whole or part for much of the modern planetary protection literature. Both of its former planetary protection officers continue to write papers on the topic today.
Sadly as we saw, over a period of a decade or two, NASA lost much of its expertise relevant to a Mars sample return mission. See above:
However NASA do have the potential to return to their world leading role in planetary protection. I have written this book to encourage them to do that.
ESA will return the samples to Earth and potentially has a significant role for independent scrutiny of NASA's plans but instead de-emphaized planetary protection, removed its planetary protection officer, and its website redirects readers to NASA's plans
ESA like NASA has a history of careful work on planetary protection in the past. The European Science Foundation is the author of the most recent planetary protection study for a Mars sample return (Ammann et al., 2012, Mars Sample Return backward contamination–Strategic advice and requirements).
ESA also was a partner with NASA in its request for the 2015 review of forward contamination of Mars (SSB, 2015. Review of the MEPAG report on Mars special regions). which raised many issues with the 2014 study that NASA relies on extensively in this PEIS (Rummel et al., 2014. A new analysis of Mars “special regions”: findings of the second MEPAG Special Regions Science Analysis Group (SR-SAG2)). See above:
ESA is also a partner NASA relies on for this mission. According to current plans ESA are responsible for returning the samples from Mars to Earth (ESA, 2019. ESA - Earth Return Orbiter) and video: (ESA, 2020. How will Airbus bring the first samples ever from planet Mars - Introducing the Earth Return Orbiter). Without ESA's help, NASA would have to add extra missions which it wouldn't have the budget for in this decade which would push back the sample return to the 2040s..
This gives ESA a potentially significant role for independent scrutiny of these plans and to raise issues or questions with them if they have concerns NASA hasn't met. As we saw, NASA's PEIS doesn't mention the size limit reduction or cite the 2012 ESF study (Ammann et al., 2012, Mars Sample Return backward contamination–Strategic advice and requirements). This is something that ESA could raise as an issue, and ask why its recommendations were not mentioned in the PEIS. ESA could ask for NASA to support its assertion that a HEPA filter can comply with the ESF requirements.
See:
- Why NASA can't rely on HEPA filters for the ESF recommendation to contain 100% of ultramicrobacteria
Also NASA hasn't done the size limit and level of assurance review which the 2012 study said was essential. This is another matter that ESA could raise with NASA. See above:
Gerhard Kminek is still listed as ESA’s Planetary Protection Officer on their website (ESA, n.d. Planetary protection).. However he was replaced in 2021 by an "ESA Planetary Protection and Space Debris Mitigation officer" (ROSA, 2921, ESA: Planetary Protection and Space Debris Mitigation Officer). The new officer has many responsibilities, including:
- Planetary Protection;
- Space Debris Mitigation;
- Nuclear Safety for applications in space;
- Re-entry safety;
- Human Spaceflight Safety.
Without a separate planetary protection officer, ESA may not look at this in any detail. However, they don't seem to have worked out a new policy to explicitly prioritize preparation for humans landing on Mars as soon as possible over planetary protection in the way NASA did.
ESA countries have a somewhat different perspective on human space exploration from NASA. For instance, ESA's big project at one stage was the Moon village (Wilks, 2016. How to build a village on the Moon) while proposals for humans to Mars tend to come from the USA. This might make it politically easier for ESA to look closely at biosafety issues with a Mars sample return.
There might also be more scope for the public to raise the issue, which might perhaps lead ESA to commission a study or review of the planetary protection implications of their participation in the mission. It's possible this book and discussion of the issues may lead ESA at least to look closely at their planetary protection responsibilities for this mission.
As one possible action, it would be natural for ESA to commission an independent update of the size limit and level of assurance for a Mars sample return mission, as is certainly required by now according to recommendation 8 of the European Science Foundation study in 2012 (Ammann et al., 2012, Mars Sample Return backward contamination–Strategic advice and requirements : 48).
They could then ask for this update to include a review of of current air filter technology to see if current technology for air filters can comply with their revised size limit and level of assurance.
They could also ask the authors to look into issues of quarantine and latency period.
This ESF update could also examine NASA's Mars meteorite finding and the other three findings, with a request to the European Science Foundation to make a statement of their conclusions about them given their centrality to NASA's biosafety plans.
That then could lead to broader dialog on the topic of an appropriate biosafety plan for Earth's biosphere.
However, as for NASA, ESA also seems to have de-emphasized it's work on planetary protection significantly since it became a partner in the project. I don't find any statements on the ESA website of any change in direction for planetary protection, unlike the clear statements in the NASA website (NASA, 2023, SMA Leadership Profile: Nick Benardini). But the ESA planetary protection page still hasn't been updated to say that the former planetary protection officer Gerhard Kminek has been replaced. It also has no mention yet that they are participating in NASA's Mars sample return mission or of planetary protection for a Mars sample return so they haven't updated it to take account of this mission. See: (ESA, n.d. Planetary protection).
Also so far I have found no public discussion of ESA's policies for the samples returned from Mars.
It looks as though ESA has followed the lead of NASA and has de-emphasized planetary protection. As with NASA, so far to be impossible to enter into dialogue with anyone at ESA either on this topic of issues with NASA's planetary protection plans for the samples they will return from Mars.
As with NASA I hope that publication of this book will help start a process that will make it easier for the space agencies involved in the project, NASA and ESA, and experts both within and outside of those agencies to enter into a wide ranging open dialogue with the general public on this topic as recommended in all the major sample return studies by both those agencies.
See:
And on the current near impossibility of dialogue here:
NASA's PEIS seems vulnerable to legal action under NEPA with potential risks to the future success of the mission - a scenario to avoid
NASA's plans do seem to run considerable risk if they are ever taken to the courts under NEPA. See :
There is no provision for legal challenges within NEPA, so it is done through judicial review, usually on the basis that: (Congressional Research Service, 2021 ).
- the agency failed to consider some of the impacts
- the agency failed to properly consider the weight of the impacts under review
An agency can only be taken to the courts by someone with “standing”. For this, they need to take part in the public comments or debate in the NEPA process, and need to be directly affected by the proposed action.
The petitioner has to show they are particularly affected by it, which is normally understood to mean more so than by others. If the petitioner simply claims NASA overlooked a worst case risk of global effects NASA could try to block it on the basis that in their hypothetical scenario any particular petitioner wouldn’t be affected more than anyone else in the world and so don’t have standing.
There are organizations with the funding and the experience to do this, such as the Audubon bird society, which could potentially get involved because of potential effects on wild birds e.g. for an alien fungus, in collaboration with someone with standing. The petitioner to have standing must not only be affected but also needs to have raised their concerns during the NEPA process during the two periods when the PEIS was open for NEPA comments.
In the past, environmental cases have gone either way based on subtle legal arguments about whether environmental effects give the petitioner “standing” for the case (Birnbach., 1997. Newly Imposed Limitations on Citizens' Right to Sue for Standing in a Procedural Rights Case).
However, if NASA used that legal argument to try to prevent legal scrutiny of their EIS. It would be a seed for polarization between NASA and the public which this book identifies as one of the main pitfalls with potential to lead to cancellation of the mission or severe delays. See:
If it does get as far as the courts, the case is usually (Congressional Research Service, 2021, National Environmental Policy Act: Judicial Review and Remedies : section: Remedies in NEPA Litigation)
- referred back to the agency (such as NASA) for further proceedings
If that is all the court does, the agency can continue with the project while it does those proceedings. However at this point the court can also order “equitable relief”
- the court can order the agency to stop the project going ahead
- order some other action (in this case perhaps ordered to sterilize the samples first?).
So if a case is taken out and it’s successful, a justice decides the remedy. A common remedy is to stop the project completely, which could prevent NASA from submitting a new PEIS done correctly.
If the justice doesn't stop the mission, the polarization caused by publicity of the case could lead to an infodemic and uninformed public opposition which might also stop a well thought through PEIS.
This seems a scenario to avoid.
In the view of the author of this book, NASA does need to comply with the NEPA requirements, which are there for a reason. The legal route doesn't seem the best way to ensure compliance though, because of an unacceptable level of risk of total cancellation of the mission, or of an infodemic and polarization of the debate, the opposite of what we need to make progress here. This could cause problems for other attempts to return the samples based on sound planetary protection principles.
Potential opportunity for NASA to officially withdraw their final PEIS and seek independent peer review, or to recommend it for independent assessment under presidential directive NSC-25
When I drew a blank with the attempts to contact NASA or ESA directly, and the Open Letter seemed to have no chance to succeed, my next idea after that was to try to ask for endorsements. This is the page I set up for endorsements:
The rationale for the page:
A letter to ask NASA to withdraw the PEIS for anyone to sign:
Both had forms to fill in to make it easy to respond. and example letters.
I got hardly any replies out of over a dozen people I contacted. Of the less than a handful of replies I got, none were prepared to endorse any statements. The academics who did reply didn't say the statements I asked them to endorse were mistaken, they just said they weren't the right person to ask, or in one case said they'd look at it later but never did.
I realise on reflection this approach of endorsements, though it seemed promising at first, is not how things are done in academia. Academics naturally are used to peer review.
This led to the idea to complete this paper which turned into a book. The aim is to seek publication to get my own work peer reviewed. Then it also led to the idea of trying to contact NASA again and this time ask it to seek independent peer review of its plans since NASA itself says its plans have no peer review. So that is my current plan.
Recently I found out the official point of contact for the PEIS is Steve Slaten, NASA's NEPA expert for JPL. He is listed on the first page of the PEIS but I never thought to check there before Nobody else that I contacted suggested I contact him. But he is clearly the person I need to contact now.
I'm now going for a new approach. It is based on what NASA themselves say that their reasoning is novel to the PEIS and that it hasn't been peer reviewed as I mentioned above in:
In my draft letter to Steve Slaten, I will ask if they have considered withdrawing the PEIS, Agencies do sometimes withdraw even a final PEIS, I found 20 examples. I suggest they publish their novel reasoning and see what happens when it goes through peer review and deal with any issues raised by their reviewers before devising a new biosafety plan.
This would seem to be the easiest way for NASA to attempt to establish scientific integrity of the novel reasoning used in the PEIS. Scientific integrity is a NEPA for a PEIS.
However at present NASA seem unlikely to wish to do that or there may be no decision maker with the necessary authority. So that then leads to the idea that the leader of NASA could use NSC-2, the presidential directive requiring NASA to alert the Office of Science and Technology Policy of any experiment that may lead to allegations of large scale or protracted effects, including biological effects.
It is a particularly clear case for NSC-25 because though NASA say in the main document that any environmental effects would not be significant, NASA in their official response to public comments in the PEIS responded saying that the risk that humans might have to live in biomes to the end of time as a result of this experiment can't be demonstrated to be zero. They go on to say that's why they need to contain the samples. So they not only didn't deny but affirmed concerns by the public for a very low risk of large-scale and protracted biological effects. See my:
In my draft letter to Steve Slaten I ask him if NASA's leader has considered suggesting that the director of the OSTP recommends an independent peer review of its plans. Appropriate members of such a peer review committee could include NASA's first two planetary protection officers since both are world experts on planetary protection for a a Mars sample return and the members of NASA's interagency panel which operated through to 2007 with members from the NIH, CDC, DoA, etc., who would have the necessary expertise to do a review of NASA's biosafety plans.
There doesn't seem to be any mechanism by which the general public or anyone else can ask for this review, only the leader of NASA. So this is just asking for information.
I plan to publish the letter and any reply on my website and link to it here (depending on the outcome and what he says)..
For the details of how NSC-25 works see the Supplementary information section under:
How NASA's systemic planetary protection issues lead to major lapses for Apollo
The issues we will describe here are systemic and not the result of any decision by any particular administrator, engineer or scientist. The experts concerned are doing the best job they could within their competence and sphere of excellence.
Rather the issue is that NASA hasn't got anyone on its team with the necessary disciplines to prepare this PEIS as we will see at various points in this analysis. That is a requirement under NEPA:
The disciplines of the preparers shall be appropriate to the scope and issues identified in the scoping process
§ 1502.6
See Supplementary information under: Fails NEPA requirement to select preparers with disciplines appropriate to the Impact Statement's scope and issues
The historical perspective may help. NASA have never been an agency responsible for biosafety or for responding to health emergencies and have a different perspective from the agencies that do have those responsibilities in the USA. If we go back to the times of Apollo, NASA did have experts available to them. But they didn't listen to them. For instance this is what Vishniac of the National Academy of Sciences told NASA about its plans to tell the Apollo astronauts to open the Apollo 11 capsule door when it was bobbing in an open sea and then exit into a dinghy:
Opening and venting the spacecraft to Earth’s atmosphere after splashdown would, in his view, make the rest of Apollo’s elaborate quarantine program pointless.
(Meltzer, 2012, When Biospheres Collide : 452).
Vishniac believed it was “irresponsible to leave a large breach in the biological barrier in any part of the recovery procedure.” and tried to deal with one of the issues, asking NASA to install extra filters over the top of the vents that vented air from the command module before it was opened. But NASA was opposed to this, concerned about a possible danger to the divers trying to install the filters on top of the vents.
After that Vishniac said:
… the Apollo Program is moving at a pace which we [ICBC] can not stop. It is equally clear that this irresistible progress is being used to brush aside the inconvenient restraints which the Interagency Committee has considered to be an essential part of the Quarantine Program.
(Meltzer, 2012, When Biospheres Collide : 202).
If there had been life on the Moon it would have likely got into the lunar dust. This covered the surfaces of the lunar module, and floated in the air and got into the astronauts noses and sinuses. Neil Armstrong remembered it as "the smell of wet ashes" and Buzz Aldrin remember it as "like it was going to catch fire" ! (Hansen, 2012. First Man: The Life of Neil A. Armstrong : 531-2). Other lunar astronauts described the smell of the dust as like the smell of burnt gunpowder. The reason for this is not known yet. One possibility is that volatiles in the lunar dust actually did combust, very slowly in the oxygen of the capsule atmosphere (NASA, 2016, The Mysterious Smell of Moondust).
Although the dust eventually settled in the lunar module, the dust floated off the surface again as they entered zero g, and got into the air and into the command module. This is Pete Conrad’s account for Apollo 12 (Wagner, 2006. The Apollo experience lessons learned for constellation lunar dust management)
“However, something we found out later and not until we got back to the ship, was that the fine dust was on the suits and on almost all of the equipment that was contained inside the bags. The dust is so fine and in zero g it tended to float off the equipment and it must have permeated the whole command module. It floated out of those bags; it floated out of the contingency sample bag.
As Buzz Aldrin describes it (Aldrin et al, 2015):
"One of the frogmen helped us to stumble into the raft and another was right there with us from then on. Waves started rolling and splashing us, causing the hatch to slam into the head of one of the frogmen. He weaved for a moment as we all moved to catch him, but he recovered quickly and motioned us back down. Another handed us scrubbing cloths and detergent with which we had to thoroughly douse ourselves twice - once with one cleaning substance, the second with another, all to counteract any contamination we might have brought from the moon. The cloths we had used to scrub ourselves were tied to weights and dropped into the ocean.”
Text on graphic: Astronauts swiftly swabbed down with bleach - then the cloths were weighted and dropped into the ocean. Background image: Apollo 11 crew await pickup by a helicopter from the USS Hornet (NASA, 2013, Apollo 11 comes home)
In the dinghy are the Apollo 11 astronauts Neil Armstrong, Michael Collins, and Buzz Aldrin, and Navy Frogman Clancy Hatleberg
There was dust in the astronauts’ clothes. The astronauts donned biological isolation garments and exited into a life-raft bobbing in a heavy sea. The navy divers quickly swabbed the isolation garments with a bleach solution. They then weighted those swabs and dropped them into the sea. Finally they disinfected the raft with an iodine solution (Meltzer, 2012, When Biospheres Collide : 404) and sank the raft (Meltzer, 2012, When Biospheres Collide : 205).
This wouldn’t have sterilized the astronauts’ outer biological containment garments, or the raft, even with the science of their day. To take an example, as one can see from the video they were only swabbed briefly.
Video: Apollo 11 Splashdown footage highlighting Navy Frogmen's role starting at 4:30
It takes twenty minutes contact with 10% bleach to sterilize Bacillus anthracis (the agent of anthrax, and a highly resistant spore). 10% of anthrax spores remain viable after ten minutes contact with bleach and complete sterilization only achieved after 20 minutes immersion in bleach. (Heninger et al., 2009. Decontamination of Bacillus anthracis spores: Evaluation of various disinfectants).
We can surely agree with Vishniac with hindsight today, that if there had been any biologically compatible life in the lunar dust it would have had an excellent chance to get into the ocean at that point. When Neil Armstrong and Buzz Aldrin returned in the lunar module to the command module piloted by Michael Collins, the lunar dust floated off the surfaces in zero g and got into the command module.
As a result, there was lunar dust everywhere inside the Apollo capsule and there would have been fine dust suspended in the air. It's certain that some dust from the Moon got into the sea, the worst place to contaminate with extraterrestrial life, when the astronauts opened the capsule door.
The chairman of the Interagency Committee, David Sencer, from Public Health Service said these plans violated the concept of biological containment (Meltzer, 2012,When Biospheres Collide : 203). His view was summarized by Meltzer as:
… NASA’s plan to open the CM after splashdown, allowing its crew to egress while the module was still bobbing in the ocean, violated the concept of biological containment. NASA had not responded adequately to ICBC recommendations and did not apparently recognize the necessity of protecting Earth’s environment against any possibility of extraterrestrial contamination.
But NASA had set up its Interagency Committee on Back Contamination (ICBC) in such a way that they could override objections by other agencies.
… the regulatory agencies agreed “not to take any actions that might have an effect on the lunar program … without the ‘unanimous recommendation of the agencies represented on the [Interagency] Committee [on Back Contamination].”
… Since NASA was itself a member of ICBC, no actions could be taken without its approval.
(Meltzer, 2012, When Biospheres Collide : 193).
NASA used those powers to override this objection so that they could keep to the timeline they had set to send the astronauts to the Moon. The ICBC eventually reluctantly agreed to NASA's proposals but the chairman David Sencer registered a formal protest.
Carl Sagan put it like this (Sagan, 1973, The Cosmic Connection – an Extraterrestrial Perspective : 114)
“The one clear lesson that emerged from our experience in attempting to isolate Apollo-returned lunar samples is that mission controllers are unwilling to risk the certain discomfort of an astronaut – never mind his death – against the remote possibility of a global pandemic. When Apollo 11, the first successful manned lunar lander, returned to Earth – it was a spaceworthy, but not a very seaworthy, vessel – the agreed-upon quarantine protocol was immediately breached. It was judged better to open the Apollo 11 hatch to the air of the Pacific Ocean and, for all we then knew, expose the Earth to lunar pathogens, than to risk three seasick astronauts. So little concern was paid to quarantine that the aircraft-carrier crane scheduled to lift the command module unopened out of the Pacific was discovered at the last moment to be unsafe. Exit from Apollo 11 was required in the open sea.”
Meltzer's history finds that there was more to it than seasickness. Amongst other issues NASA was concerned about the astronauts overheating in the tropical conditions in a not very well vented module, about the cranes which weren't manrated, about wind blowing the ship to bear down on the command module, and about an issue with the command module penduluming against the ship if it was lifted from a heavy sea at the wrong moment. (Meltzer, 2012, When Biospheres Collide : 197 - 206).
Meltzer's conclusion however was similar to Sagan's. The breaches in containment were largely because of NASA's focus on crew safety.
Central to NASA's management of the Apollo program was, as discussed above, the importance given to astronaut safety. NASA simply would not put its spacecraft crews in danger in order to meet quarantine requirements. Decisions based on this policy sometimes, as in the case of the Apollo CMs, ran contradictory to optimal planetary protection actions.
It is important to note that when confronted with prioritizing either a very low-probability event with potentially global consequences (back contamination of Earth) or a much higher probability event with a limited potential consequence (injury to one or more of the three astronauts), NASA consistently chose to protect against the latter. Why was this? John Stonesifer thought it was because NASA did not believe that back contamination would actually occur, whether the Agency practiced planetary protection procedures or not. 271 The astronauts, on the other hand, could be placed in real danger if they stayed in the CM while the USS Hornet attempted to maneuver close enough to connect its crane to the module and haul it aboard.
The astronauts were people whom many NASA staff personally knew. What's more, many people around the world considered them heroes for conducting the voyage to the Moon. Protecting their lives was, not surprisingly, something that NASA staff strongly wanted to do. By comparison, back contamination was a theoretical danger in that it had never, so far as space scientists knew, ever happened to Earth. Back contamination did not have the recognizable face of Neil Armstrong or Buzz Aldrin; it was a concept, not a human being. It was thus not unexpected that NASA chose to defend against the known, proven danger to several of its friends, rather than against an extremely unlikely risk to strangers.
There was a solution with no risk to human astronauts or other humans or Earth's biosphere. That was to do telerobotic exploration of the Moon which the Soviet Union was already doing with its twp Lunakhod rovers and sterilize all samples returned to Earth. It would have been slower, but it wouldn't have taken many months or perhaps a year or two to establish that there can't be any life on the Moon because it turned out to be so uninhabitable. Then we could have sent humans to the Moon confident that there is no risk to Earth's biosphere.
However this solution was "above their pay grade", NASA and the other agencies were tasked with finding the safest way to send humans to the Mon and back from the point of view of biosafety.
We don't have a wider context here, we only have the case of our own Moon which was uninhabitable. However in the universe as a whole many moons may be habitable or there may be twin planets both habitable and with different forms of biology. If so, it may be that other intelligent species on occasion have attempted similar missions at the Apollo level of technology, without adequate biosafety plans, but were less lucky and returned life to their planet that caused major issues for their biosphere or their health.
In particular we can't deduce that Mars is harmless because it has a far higher potential for habitability than the Moon.
It is understandable for NASA to have different priorities from experts whose focus is on biosafety. So these tensions between NASA and the other agencies are also understandable.
However this difference of focus can lead to NASA not developing a biosafety plan adequate to protect the Earth as a whole against very low probability but serious adverse effects.
How systemic issues similar to those for Apollo led NASA to lose its world-leading expertise on planetary protection for a Mars sample return mission and close down its Interagency Panel
The systemic issues facing NASA today that led to them assembling a team to prepare a biosafety plan for Earth, with nobody on the team familiar with the literature on a Mars sample return are similar to those they had with Apollo. The main issue as before is that their experts weren't listening to the advice on biosafety and planetary protection that external experts gave them.
As a result these experts played less and less of a role in NASA's decision making, until eventually their advice was completely non functional. NASA dismissed these experts only after it reached a point where they were no longer fulfilling any useful purpose in the organization.
This makes this an issue that is far harder to solve than by just reversing the decisions to close down the panel and planetary protection office.
NASA had an interagency panel for a few years at the start of the 21st century called the Planetary Protection Advisory Council (PPAC). By its charter (SpaceRef editor, 2001, Planetary Protection Advisory Committee Charter) this panel included scientists from:
- Department of Agriculture
- Department of Energy
- Department of Health and Human Services
- National Institutes of Health
- Centers for Disease Control and Prevention
- Department of Interior
- Department of Transportation
- Environmental Protection Agency
- National Science Foundation
- Executive Office of the President
The panel also had at least four members knowledgeable in one or more of the fields of bioethics, law, public attitudes and the communication of science, the Earth’s environment, or related fields.
However, the experts at NASA listened to the interagency panel less and less and eventually NASA closed it down.
This is a brief summary of the history of the panel by the Space Studies Board, in short
- PPAC formed in 2000 as an independent committee as expected.
- PPAC only operated as an independent advisory board to 2007,
- PPAC was removed from the NASA Advisory Committee [NAC] in 2007
- PPAC was turned into a subcommittee that could only report to NAC indirectly
- this subcommittee was disbanded in 2017..
To be effective, planetary protection measures should be integrated into the engineering and design of any sample-return mission, and, for an oversight panel to be in a position to coordinate the implementation of planetary protection requirements, it should be established as soon as serious planning for a Mars sample-return mission has begun.
… After a NASA task force reiterated the need for this type of committee, the Planetary Protection Advisory Committee (PPAC) was formed in 2000
… PPAC’s charge was to advise the OPP [Office of Planetary Protection], review proposed missions at an early stage, and assign a planetary protection category to them. The OPP then informed the mission team as to the necessary planetary protection requirements to be met.
In 2006, the NAC [NASA Advisory Council.] was reorganized and the scientists [from PPAC] then serving on the Council, including the chair of PPAC, were removed [from the NAC]. The PPAC became a subcommittee under the NAC’s Science Committee. This change caused concern among the PPAC members because, by being subordinated under a committee that focused on the science missions, the new planetary protection subcommittee lacked the level of independence necessary for effective planetary protection. During this period, neither the PPAC nor the Planetary Protection Subcommittee received the attention needed for renewing and refreshing the membership.… By 2016, the committee had become completely moribund, and it was formally disbanded in late 2017.
(SSB, 2018, Review and Assessment of Planetary Protection … : 26 - 27).
Finally, NASA closed down its planetary protection office, which had some measure of independence from NASA, able to review NASA's decisions. It operated from 1997 to 2017 (Voosen, 2017, With planetary protection office up for grabs, scientists rail against limits to Mars exploration).
NASA's current planetary protection engineer is now an employee in its Office of Safety and Mission Assurance, with no independence from NASA. He says says NASA's priority for planetary protection is to prepare the way for humans to go to Mars as fast as possible, and NASA wants other agencies to help support this goal. NASA posted this on 1st January 2023, 12 days after the end of the second period of public comments under NEPA was closed.
This isn’t the Planetary Protection of the past — we are doing things differently. We have a different approach and philosophy.
There’s still a lot of work to go as we start to pave the way to humans on Mars — we’ve never done that, it’s a new precedent, so we’ll need that continued support to help with managing those knowledge gaps, including management support, engineering support and of course funding support.
The rubber is hitting the road; it’s time to get it done and we need that collective agency support to do that.
(NASA, 2023, SMA Leadership Profile: Nick Benardini)
However from their recent history, though NASA wants other agencies to support its goals, they are not in dialogue with the other agencies to achieve these goals together. Its plans would surely be very different if they were worked out together with their second planetary protection officer and the interagency panel, and if the interagency panel had authority to call up NASA on mistakes that it identified and require them to fix them.
This seems systemic, not an individual decision, simply that NASA's mission planners and engineers collectively paid less and less attention to the other agencies and to the planetary protection advisors, until they no longer served any useful function. As I understand it, that came first before the decision to close them down.
This is surely an important factor in what happened. Another factor may be a belief based rather than evidence based approach to planetary protection.
NASA's goal to land humans on Mars is only possible if it is biologically safe to do that. NASA has set this as its next big goal for humans in space, an intermediate goal towards their end goal of humans throughout the solar system. However this goal can only be achieved in its current form if it is safe for humans to travel back and forth between Mars and Earth. Since it is impossible to sterilize humans or break the chain of contact in a spaceship that humans travel in, that is only possible if any martian life is safe for Earth.
It is so much simpler, costs much less and means no problems for NASA's end goal for humans on Mars if there is no need to do planetary protection and it is as safe to send humans to Mars as for the Moon. Since NASA is certain this is what we will find out to be the case a decade or two from now, it is a natural next step to believe that it is surely safe already, and we just need to prove that there are no issues for life returned from Mars to Earth or for terrestrial life on Mars. With this background as a belief, it is a natural step to see this sample return as a transitional mission with less planetary protection than before but still some symbolic measures of planetary protection until we finally discover, as they expect, that it is safe to send humans to Mars and back again both ways.
This is perhaps combined with the natural tendency of engineers to have general confidence in their capabilities. They are used to surmounting almost any problem with ingenious engineering, and indeed NASA and humans generally have achieved extraordinary engineering accomplishments in the last century. Engineers may reason that surely this can be surmounted too, that there has to be an engineering solution for a way to send humans to Mars and back again safely, and they just have to find out how to do it.
However this is messy biology rather than engineering and so far we don't know what we will find on Mars because we haven't looked since the on and only time we looked for life there with the Viking missions.
It has to be a possible answer that we find Mars is biologically unsafe and that we can never land there. That after all is why we do planetary protection, because we don't know that it is safe.
This is why this book suggests NASA changes its intermediate goal to one that is evidence rather than belief based. NASA's end goal remains the same.
We can actually achieve the same end goal of sustained human presence throughout the solar system with a different intermediate goal with humans in orbit around Mars and on the Martian moons, exploring Mars by telepresence with sterile robotic avatars on Mars. The astronauts would have an interesting and exciting mission goal, to complete a rapid astrobiological survey of Mars, at the end of which we can hope to find out enough about Mars to know whether or not it is biologically safe to land. After that, it is well possible that we make the same decision in the end that it is safe to land humans on Mars, but it will then be evidence rather than belief based. Because this approach is evidence based, we also need to be prepared for the eventuality that the answer is that it is not biologically safe to land.
This exploration with telerobotic avatars from orbit achieves more science return about Mars, faster, than missions with humans in a base on the surface, according to studies like the HERRO study. It also avoids the risk of stranded assets on Mars if we find it is biologically unsafe to land. We look at this below:
NASA's novel conclusion that any effects would be local to the Utah Test and Training Range, and not significant
As we've seen the consensus in the planetary protection literature is that there is potential for large scale global harm though the risk of this is likely very low. See above:
NASA is the outlier here. From the outset, NASA reduced the scope of the Programmatic Environmental Impact Statement to the Utah Test and Training Range (UTTR) by specifying this as the Affected Location instead of Global as one would expect from previous studies. They leave sample containment in the Sample Receiving Laboratory to tier 2,to be sorted out internally in discussions with the CDC and NIH once they have decided on a location for the biosafety lab.
Affected location: Utah Test and Training Range
(NASA, 2023. Mars Sample Return final PEIS : 1)
NASA elaborates on this in the PEIS, assuring the public that there will be no significant impacts expected at the landing site and no impacts outside of it of any breach of the sample container during the impact into the desert sands.
No significant adverse impacts are anticipated at the UTTR or DPG.
(NASA, 2023. Mars Sample Return final PEIS : S-13)
(DPG = Dugway Proving Ground)
Within the context of the Proposed Action, there are no environmental justice concerns associated with mission preparation or EES landing and recovery operations as these activities would all occur within the confines of the UTTR South Range and DPG boundary. There are no anticipated effects outside this area; therefore, there would be no environmental justice concerns associated with activities at the UTTR or DPG.Analysis of similar activities at the UTTR and DPG were found to have no significant impacts on environmental justice communities (see Table 1.1-1).
(NASA, 2023. Mars Sample Return final PEIS : 3-63)
There the table 1.1.1 of “Similar” activities include return of samples from asteroids and a comet, and the launch of the James Web space telescope. The asteroid and comet samples were all classified as unrestricted sample return missions by COSPAR and as a result, none of them required Earth’s biosphere to be protected from the samples.
Experts have no concerns about sample returns from Bennu or other Near Earth Objects including Near Earth Comets. That's by Greenberg's natural contamination standard, the same reason as for Phobos sample returns” (Greenberg,et al., 2001. Macroscope: Infecting Other Worlds ).
But as we’ve seen, the same reasoning doesn’t apply to the Mars samples. These are classified as restricted category V which means Earth’s biosphere does have to be protected.
The plan is to let the capsule slow down to terminal velocity without a parachute and then fall onto the sands much like any large object falling from a great height and the capsule is designed to withstand the stress of impact (Mitcheltree, 1998. A passive Earth-entry capsule for Mars sample return) (Andrews, 2020, Rocks, Rockets and Robots: The Plan to Bring Mars Down to Earth, Scientific American).
The UTTR sands are where the capsule containing the Mars samples will hit the ground with NASA's proposed mission plan:
Text on graphic: Affected Location: Utah Test and Training Range (UTTR), Utah
[ crossed out replaced by:]
Global (all major studies)
Considerations for the Mars Receiving Facility are relegated to Tier II with details to be worked out in consultation with the NIH and CDC later, long after the scoping process which the public takes part in.
As discussed in Chapter 1 (Purpose and Need for the Proposed Action), this PEIS analyzes the potential impacts of the MSR Campaign both programmatically (flight and ground elements) and site specifically (Earth-based launch elements and landing of the EES at the UTTR). Appropriate transportation, storage, and curation protocols for the Mars samples, including transportation from the UTTR landing site, are currently under investigation, with details incomplete at this time.8 This PEIS identifies and evaluates, from a programmatic perspective, the conceptual transportation methods and representative SRF options (i.e., new construction, existing facility, modular, or hybrid) that are most likely applicable to this future recovery and curation action; however, those elements of the Proposed Action cannot be analyzed from a site-specific perspective at this time.
Subsequent Tier II National Environmental Policy Act (NEPA) analyses will address site-specific impacts associated with sample transportation off the UTTR and type, location, development and operation of an SRF.
(NASA, 2023. Mars Sample Return final PEIS : 2-3)
Biocontainment will be accomplished per Biosafety in Microbiological and Biomedical Laboratories (CDC 2020) and NIH Guidelines for Research Involving Recombinant or Synthetic Nucleic Acid Molecules (NIH Guidelines)
(NASA, 2023. Mars Sample Return final PEIS :S-4)
Because the SRF will be a high- containment laboratory, the requirements for sample handlers will follow similar proven processes developed by the NIH and CDC’s Biological Surety Program, which includes the Personnel Reliability Program.
(NASA, 2023. Mars Sample Return final PEIS : 4-9)
This can be controversial in Environmental Impact Statements, and can risk litigation if the matters that are relegated to tier II are important ones that should have been considered at Tier I and should have been placed before the public during the scoping process.
Relegating important matters to Tier II can be a legally risky decision.
Distrust of Tiered Process. The tiered process can be viewed by agencies and stakeholders as a means of deferring consideration of sensitive issues until it is too late for meaningful involvement. In those cases, the tiered approach can become controversial and can even become the subject of litigation
(PB Americas, Inc. and Perkins Coie LLP, 2009. Guidelines on the Use of Tiered Environmental Impact Statements for Transportation Projects : 28 - 29)
An agency would normally only do this if confident these are matters that don't need discussion at Tier I. NASA has that confidence but based only on its own novel conclusions.
The reason for this decision is explained in Table 2.4-1 and is based on NASA's two novel conclusions. These are the most important entries:
Health and Safety: Programmatic: Significant adverse impacts associated with EES transportation to an SRF are not anticipated. The travel and handling procedures for the EES and the security and functionality of the SRF would be based heavily on the proven techniques used for safely handling biological toxins and known infectious agents used in Earth-based research labs. Potential impacts associated with SRF development and operation would be related to the location of the facility, as well as the type and size. Tier II analyses for determination of impacts associated with health and safety would consider the location of the proposed facility and surrounding community/land use type, health and safety system requirements associated with a BSL-4 equivalent facility, and risk analysis involving failure of containment systems that results in a release within the facility.
[MY COMMENT IN RED TEXT[
Based on NASA's novel conclusion that any health impacts from a lab leak from a Mars Receiving Facility would be negligible
(NASA, 2023. Mars Sample Return final PEIS : 2-30)
Biological Resources: Programmatic: Transportation of the EES to an SRF would not be expected to have an interaction with biological resources. Operation of an SRF would not be anticipated to impact biological resources; the main impact driver for this resource is the development of an SRF.
(NASA, 2023. Mars Sample Return final PEIS : 2-33)
[MY COMMENT IN RED TEXT[
Based on NASA's novel conclusion that the potential environmental impacts would not be significant
This is not based on new peer reviewed studies. Instead this is based on the non peer reviewed arguments in the PEIS itself.
All its arguments build up to a conclusion that any environmental effects would not be significant.
The relatively low probability of an inadvertent reentry combined with the assessment that samples are unlikely to pose a risk of significant ecological impact or other significant harmful effects support the judgement that the potential environmental impacts would not be significant.
(NASA, 2023. Mars Sample Return final PEIS : 3-16)
See below:
Also
… the risk to the public [from lab leaks] is negligible
(NASA, 2023. Mars Sample Return final PEIS : 3-14)
See below:
To get to these conclusions, NASA used the four apparently plausible findings for the harmlessness of martian life which we have already looked at.
That with certainty or near certainty
- if there is life on Mars it already got here in meteorites
- Mars is uninhabitable
- any martian life would be unable to harm humans
- any life on Mars wouldn't be able to survive terrestrial conditions
These would be major new findings in planetary protection if true. But these are not found in the planetary protection literature. Instead they are all rebutted in the literature.
See:
So this restriction to the Utah Test and Training Range is s based on NASA's own non peer reviewed reasoning based on arguments and its invalid final deduction step from low risk of significant environmental effects to a judgement that any environmental effects would not be significant and from our ability to contain known pathogens in biosafety labs to a conclusion that the public risk from novel biology from another planet is negligible.
This is a NEPA failing because PEIS never mentions the majority view that the worst case is global harm. This fails requirements on identifying the affected environment:
NEPA clarifies that reasonably foreseeable in section 1502 includes low likelihood events with catastrophic consequences which would certainly cover a low likelihood of harm to the entire biosphere of Earth or to human health globally.§ 1502.15 Affected environment
The environmental impact statement shall succinctly describe the environment of the area(s) to be affected or created by the alternatives under consideration, including the reasonably foreseeable environmental trends and planned actions in the area(s).
(d) For the purposes of this section, “reasonably foreseeable” includes impacts that have catastrophic consequences, even if their probability of occurrence is low, provided that the analysis of the impacts is supported by credible scientific evidence, is not based on pure conjecture, and is within the rule of reason.
§ 1502.21 (c) and (d)
The conclusions of studies by the ESF and NRC were supported by credible scientific evidence and are within the rule of reason. So this seems an exceptionally clear case of a "reasonably foreseeable" potential for global catastrophic consequences in the sense of this NEPA definition, even though the probability of occurrence is considered by all to be low.
See Supplementary information under Fails NEPA requirement to include reasonably foreseeable environmental effects in discussion of the affected environment
The ESF study in 2012 says it is reasonable to assume that the Sample Receiving Facility is the most likely cause of an accidental release (Ammann et al., 2012, Mars Sample Return backward contamination–Strategic advice and requirements 33):
See below:
However there are issues involved with the capsule re-entry itself.
So we do need to look at this phase of the operation too.
NASA's plan to return a sample without a parachute based only on the Genesis mission which broke apart when its parachute failed to deploy and with no experience of containing samples from comets or asteroids to prevent contamination of Earth's biosphere
NASA agree that the capsule has to be treated as a biosafety hazard(NASA, 2023. Mars Sample Return final PEIS :3-35) as we'll see in the next section.
NASA tell the public that NASA and ESA have extensive experience with very similar activities over the last three decades and that its plans have had independent review by external experts. This is true up to a point, but with some important caveats.
- this experience is with samples that didn't need to be contained, only protected from terrestrial contamination,
- NASA and ESA have no previous experience of returning samples without a parachute except one mission where the parachute didn't open and the capsule broke open.
- the external experts that looked at NASA's current plans don't include anyone with expertise in biosafety and biocontainment; it lost that expertise in 2006 when it closed the interagency panel.
NASA's team give this reply in response to this aggregated question from the general public in the first round of public comments asking for a target probability of containment for the mission:
When the consequences of a failure are so great, a 100% guarantee should be required. ... Just how low is “low likelihood”? Is NASA’s goal specification to prevent accidental release of the Mars samples 1 in a thousand? 1 in a million? 1 in a billion?
(NASA, 2023. Mars Sample Return final PEIS : 4-8)
NASA doesn't reply on the goal specification, but just says it has independent review by external experts.
No outcome in science and engineering processes can be predicted with 100% certainty The safety case for MSR safety is based on redundant containment supported by rigorous testing and analysis, the extensive experience of NASA and ESA with very similar activities over the past three decades, as well as independent reviews of program plans by external experts
(NASA, 2023. Mars Sample Return final PEIS : 4-8)
NASA do eventually answer the original question but only in a reply to Chester Everline in round 2, published in the final PEIS, the answer is they don't have a campaign level target probability. See Supplementary information under:
When NASA say it has independent reviews of program plans by external experts, this does not include experts in public health, biosafety or biocontainment. NASA leave this area of expertise for tier 2.
One of the areas most in need of this expertises is the design of the Mars Receiving Facility. The authors of the 2022 paper make it clear that nobody in the MSPG2 team that prepared reports for the PEIS in 2022 has this expertise. They defer those considerations to a later team that will implement the facility.
Beyond the scientific capabilities outlined above, there are myriad building and engineering requirements needed to complete the design of the SRF and thus support the overall science goals of MSR. The specifics of these requirements are outside the expertise of MSPG2 members and are thus incomplete in their identification in the proposed SRF Design Requirements table and in the discussion sections below. The building and engineering requirements needed to complete the design of the SRF should be addressed by the SRF implementation team and subsequent building contractors.
(Carrier et al., 2022. Science and Curation Considerations for the Design of a Mars Sample Return (MSR) Sample Receiving Facility (SRF) : S-232)
See:
So, yes the plans have been looked at by external experts but only to look at the science requirements, not to check biosafety or biocontainment.
In that same reply to the general public, NASA say that along with ESA, it has extensive experience with very similar activities to this sample return mission.
No outcome in science and engineering processes can be predicted with 100% certainty The safety case for MSR safety is based on redundant containment supported by rigorous testing and analysis, the extensive experience of NASA and ESA with very similar activities over the past three decades, as well as independent reviews of program plans by external experts
(NASA, 2023. Mars Sample Return final PEIS : 4-8)
However, this experience is for samples already assessed to pose no risk to Earth's biosphere before the sample return, from comets or asteroids. The only concern was to protect the samples from contamination by terrestrial organics. There was no attempt to protect Earth's biosphere from any of these samples during the landing or after transport to the labs,
Also all these sample returns used parachutes for a gentle landing. One of those capsules, the Genesis capsule, actually did break open during the landing, which NASA doesn't mention in this passage. This is the only previous experience NASA or ESA have of a hard landing of a sample returned in a comet.
NASA knew that it can't rely on parachutes before the Genesis crash. The plan instead is to build a capsule designed to crash, designed to withstand impact into the sands at terminal velocity. This doesn't mean that it will crash at kilometers per second as the capsule will be slowed down by the atmosphere and will be similar to the impact of debris falling from a plane. However it will need careful design as an impact at terminal velocity can still lead to considerable damage as the example of the Genesis probe showed:
Text on graphic: The Genesis capsule crashed because it failed to deploy a parachute.
This is why NASA/ESA plan to design their capsule to withstand impact into the sand without a parachute.
There was no risk to Earth's biosphere from Genesis and no space agency has ever had to contain a returned sample since Apollo.
Photo from (NASA, 2006, Genesis: Learning from Mistakes)
INSET: Mars samples test drop without parachute
INSET: video frame from (NASA, n.d. Dropped in the Name of Science, Mars Sample Return Design Holds Up)
The Genesis parachute failed to deploy, due to a hardware mistake. It used electronics designed for a previous mission which hadn't been integrated it into Genesis properly. The gravity-switches (or g-switches) to release the parachute flipped but didn't make the connections needed to start the parachute deployment.
Then, gravity-switch devices inside the capsule had to sense the braking caused by high-speed entry into Earth’s atmosphere and initiate the timing sequence that led to deployment of the drogue parachute and parafoil, approximately nineteen miles above ground.
...
The box that held the g-switches for Genesis was different than the one for Stardust, so the electronics had to be placed in a different configuration to make them fit. The new design for Genesis was analyzed and tested to ensure the switches still flipped, but it was not tested to ensure the switches would start the process for parachute deployment.
...
So when one team verified that the switches did what they were supposed to—which to them meant flipping from one orientation to another—another team thought full testing had been done, ensuring the switches flipped and made the correct connections.
(NASA, 2006, Genesis: Learning from Mistakes).
This didn't lead to any potential risk to Earth's biosphere because the Genesis capsule returned particles from the solar wind streaming out from the sun. There is no possibility of life in the solar wind (NASA, 2004, Genesis Sample Return Press Kit : 24-25).
The other ESA and NASA sample returns were from Near Earth Objects and there is no risk from those either because of a natural influx of material (the meteorite argument works for them).
With all these missions, the issue was just to protect the samples from contamination by terrestrial organics.
Because we do have to protect Earth's biosphere from the Mars sample return, NASA can't risk a parachute failure. So NASA's plan is that their sample return capsule won't use a parachute. This is a design choice they made already before the Genesis capsule mishap, so they were already aware of parachute failure as a potential issue
Instead, it will be designed so it can just hit the sands at terminal velocity, slowed down by friction with the atmosphere. Leaving out the parachute also saves on the mass of the capsule which has to be sent all the way to Mars and back.
The primary requirement on the Earth-entry capsule is to assure containment of the Mars samples during the intense Earth entry, descent, and impact phase of the mission. This planetary protection concern led to the consideration of a parachute-less entry since exclusion of that descent subsystem eliminates a major vehicle failure mode and reduces mass and complexity of the vehicle.
(Mitcheltree, 1998. A passive Earth-entry capsule for Mars sample return)
NASA's will design its sample return capsule to hit the sands without parachute, slowed down to terminal velocity by friction with the atmosphere. It’s expected to hit the sands at around 40 meters / second (NASA, n.d. Artist's Concept of the Earth Entry System for Mars Sample Return). That’s 90 miles per hour, or 144 kilometres per hour or the speed of a high speed car crash. NASA tested it by dropping it from 1,200 feet, or 365 meters (NASA, n.d. Dropped in the Name of Science, Mars Sample Return Design Holds Up)
By leaving out the parachute, NASA removes one potential failure point. This also saves on the mass of the capsule which has to be sent all the way to Mars and back. (Mitcheltree, 1998. A passive Earth-entry capsule for Mars sample return ).
This is a novel approach which is more robust in terms of containment, but never tried before for a returned sample. The design seems good but we certainly can't say NASA and ESA have extensive experience of this approach. As Jorge Vago put it, ExoMars project scientist at ESA:
It just comes in, and, wham, it hits the ground. That’s going to be an interesting one.”
(Andrews, 2020, Rocks, Rockets and Robots: The Plan to Bring Mars Down to Earth, Scientific American)
The National Academy of Sciences 2009 Mars Sample Return planetary protection study mentioned the Genesis sample return as examples to show the importance of scrutinizing this stage of the sample return:
The experiences from Genesis and Stardust sample return mission have demonstrated the increased importance of scrutinizing the entire sample-handing and containment chain, including the landing site characteristics, ground recovery and transport to ground facilities, not just the quarantine or containment laboratory per se (Stardust and Genesis didn't have quarantine laboratories).
...
To avoid jeopardizing mission success, there is a strong need to incorporate all aspects of an SRF and sample handling at the earliest stage of Mars sample return mission planning.
(SSB, 2009, Assessment of planetary protection requirements for Mars sample return missions : 59)
Here are some suggestions for possible pathways for release:
- unsterilized microbes on the exterior of the capsule - the exterior of the capsule will be exposed to the Martian environment briefly, and some dust could land on it, NASA plan to use ultraviolet to sterilize it but a single dust grain can cut out orders of magnitude of UV from a microbe that happens to be directly beneath it and possibly more for a microbe imbedded in the dust grain, and any martian life might be adapted to transport in dust storms, might resemble a dust grain with special biocoatings to protect against UV. If Mars has a second genesis the life there might also be naturally more resistant to UV.
See below: Ultraviolet light is significantly blocked by a single grain of dust and so may not be adequate for a high level of confidence to sterilize any possible microbes even on the exterior of the Orbiting Capsule - Flaws in the design, components, assembly or construction of the capsule as for the Genesis capsule – the Genesis capsule had its G sensors installed upside down so they didn’t detect that the capsule was decelerating when it hit the atmosphere and so never deployed the parachutes (Nusca, 2009. Design flaw lead to Genesis probe crash) (NASA, 2005, GENESIS mishap investigation report)
The Mars sample return capsule is designed to re-enter without a parachute, it also has to be as light-weight as possible and as a first mission of its kind it could still have a design flaw leading to damage or breakup from the impact when it hits the ground or deceleration, pressure changes or heating during the re-entry - Penetration of the capsule by a micrometeorite or space debris on the way back to Earth
- Capsule tips over during re-entry and doesn't land nose first as the capsule is only designed to land nose first..
- Damage to the capsule due to heavy impact with the Earth perhaps hitting a small rock or some debris on the ground
- Damage to the capsule in an incident involving the recovery vehicles or personnel due to an accident or fire or other incident
So we can't rely on the capsule design to protect the contents during re-entry.
Potential limitations in NASA's plan to use chlorine dioxide and aldehyde to sterilize the sands around the landing site
NASA agree that the capsule has to be treated as a potential biohazard. All personal protective gear would be treated as potentially hazardous biological waste.
For the soils its proposed solution is to decontaminate the landing site
After removal of the EES, the entire landing site (consisting of the impact area and extent of ejecta) may be decontaminated as a precautionary measure
Because the EES should be treated as though potentially hazardous until demonstrated otherwise, the EES would be handled under BSL-4 equivalent protocols and the recovery team would be wearing appropriate personnel protective equipment. The recovery team would handle the landing event as though containment has been compromised and ensure proper containment of the EES. After removal of the EES, the entire landing site (consisting of the impact area and extent of ejecta) may be decontaminated as a precautionary measure.
The process of retrieving the EES and placing it into the vault would be assumed to generate potentially hazardous biological waste until demonstrated otherwise. As described earlier, the process of placing the EES into containment and then inserting it into the vault would be conducted as in past missions. All the systems used, including personnel protective gear, would be assumed to be contaminated and would either be decontaminated or simply discarded as hazardous waste. Wastes could include plastics and clothing. Any liquids used in the decontamination process would be absorbed onto solids prior to disposal.
(NASA, 2023. Mars Sample Return final PEIS :3-35)
NASA believes that it can decontaminate the soils with chlorine dioxide such as is used in drinking water and aldehydes, a disinfecting fumigant :
The standard decontamination of biohazards in soil typically involves applying chemical sterilants as liquid or fumigants (such as chlorine dioxide or aldehyde) in place (EPA 2017).
NASA believes these types of decontaminates would be effective given the assumption that any putative Mars life forms would be similar to “life as we know it” with a water-mediated carbon-based biochemistry, and that there would not be any “unique” biohazards associated with the Mars samples.
Chlorine dioxide is a disinfectant. When added to drinking water, it helps destroy bacteria, viruses and some types of parasites.
...
Aldehydes are highly effective, broad-spectrum disinfectants, which typically achieve sterilization by damaging proteins. Aldehydes are effective against bacteria, fungi, viruses, mycobacteria and spores.
(NASA, 2023. Mars Sample Return final PEIS :3-35)
This surely needs more thorough study for the special case of extra-terrestrial life from Mars before we can know if such methods are feasible. Extraterrestrial life might well be similar to "life as we know it" but that doesn't mean it is identical in its response to chlorine dioxide or aldehyde.
Also this method has limitations even for sterilization of terrestrial soils.
From their cite, this shows the effect of 24 hours of high concentrations of Chlorine Dioxide. It has almost no effect on top soil below a depth of one inch below the surface. It is much more effective on clay or sand with a 100 million fold reduction
(EPA. 2017. Assessment of the Decontamination of Soil Contaminated with Bacillus anthracis Spores Using Chlorine Dioxide Gas, Methyl Bromide, or Activated Sodium Persulfate :3-35)
The soils of the UTTR consist of salty silt, clay and sand. So this may be reasonably easy to sterilize to significant depth, at least to that 100 million-fold reduction standard.
The Playas are found on lake plains that are relatively barren, undrained basins subject to repeated inundation by water and salinization by evaporation of accumulated water. The soil material in the Playa is strongly calcareous, stratified silt, clay, and sand containing sufficient amounts of salt to limit or prohibit the growth of vegetation. The Playas-Saltair and Saltair-Playas soils are very deep and poorly drained soils also found on lake plains. This soil type is formed in alluvium and lacustrine sediments derived from mixed rock sources. The Playa water capacity is very low, while the Playas-Saltair water capacity is very low to low. Mudflat soils are poorly drained, strongly saline soils that consist of the Playas-Saltair Association. These soils are found on nearly level lake plains or basins that are subject to repeated salt water flooding and salinization by evaporation of accumulated salt water. Mudflat soils are usually smooth, crusted with salt, and patterned by cracks when dry. These soils are also strongly calcareous and have a silty clay, silty clay loam, or silt loam texture.
(Hill Air Force Base. 2007. Operations and Environmental Conditions at the Utah Test and Training Range as of December 31, 2007Operations and Environmental Conditions at the Utah Test and Training Range as of December 31, 2007 : page 31, section 2.3.4)
However, this is for reduction in “colony forming units” in other words cultivable spores. Many microbes are uncultivable.
Also any martian life would be adapted to surface conditions with high concentrations of perchlorate as well as high levels of UV, adapted and evolved there for billions of years. It might well be more resistant to chlorine dioxide than terrestrial life. For instance any spores may have extra coatings to protect them. See below:
This also depends on the target probability and estimates for how many viable spores there may be in the released material. E.g. for a 1 in a million level of containment, a 100 million fold reduction is adequate provided that the released material has less than 100 viable spores in it.
The ESF set a target of 100% containment at 0.05 microns.
- Why NASA can't rely on HEPA filters for the ESF recommendation to contain 100% of ultramicrobacteria
NASA doesn't have a target as we saw.
Also, what are the contingency plans if Martian life has got into the microbiome of a human involved in the sample recovery? That leads to the issues with lab leaks.
Also if we need to protect Earth to a high standard, we need to consider such things as a contaminated insect that flies away from the site after exposure to the dust, before the fumigation begins.
Also it might be a windy day. So what is the plan to contain any martian dust that gets blown away from the site in the atmosphere? The martian dust is as fine as cigarette smoke. If some gets dislodged it could be blown around with the gentlest of brezes.
It may be a challenge to contain the samples even at the Utah test and training ground to secure a high level of containment.
Impact of limited scope for tier 1: NASA only publicised its PEIS and scoping meetings in newspapers local to the Utah Test and Training Range and Kennedy Space Center
We see the effect of this limited scope for tier 1 in the very limited publicity for the PEIS. As a result of their decision that any effects would be local to the Utah Test and Training Range, NASA felt they fulfilled the obligations to alert the public under NEPA with advertisements in local newspapers in the region of the ground itself
Scoping: NASA published advertisements in local newspapers near the Utah Test and Training Range and Kennedy Space Center two weeks prior to the scoping meetings.
Draft PEIS Review NASA placed a notice in the Federal Register, made an announcement on NASA's NEPA website, and published advertisements in local newspapers announcing the availability of the Draft PEIS along with its public review and comment period.
(NASA, 2023. Mars Sample Return final PEIS : 4-1)
Text on graphic: Only publicised local to the Utah test and Training Range not nationally.
I personally only found out about this because of an online friend who knew I was writing a paper on the topic. Most people would only have found out from the few journalist articles written after the process was completed.
NASA's novel deduction that there would be NO significant ecological impacts, based only on its assessment that samples are UNLIKELY to pose a risk of significant ecological impact
In some ways this is the most important sentence in the whole report.
NASA: The relatively low probability of an inadvertent reentry combined with the assessment that samples are unlikely to pose a risk of significant ecological impact or other significant harmful effects support the judgement that the potential environmental impacts would not be significant.
(NASA, 2023. Mars Sample Return final PEIS : 3-16)
So NASA concluded that there would be NO significant impacts from release of the samples to Earth's biosphere. If NASA's conclusion here is right, there could be impacts but if there are any they won't be significant.
As we saw this goes against the consensus in the planetary protection literature that there is potential for large scale global harm from release of unsterilized materials from Mars, though the risk of this is likely very low. See above:
It is this novel deduction that lets NASA deduce that any effects would be limited to the Utah Test and Training Range, as we saw in the last two sections.
- NASA's novel conclusion that any effects would be local to the Utah Test and Training Range, and not significant
- Impact of limited scope for tier 1: NASA only publicised its PEIS and scoping meetings in newspapers local to the Utah Test and Training Range and Kennedy Space Center
I asked NASA for a cite for their conclusion. NASA said it is based on its own novel reasoning in the PEIS itself.
In the screenshot , I quote this sentence from the draft PEIS which remains unchanged in the final PEIS and (as we've seen) goes against the conclusions of the NRC study from 2009 which says the risk of large scale effects appears to be low but not demonstrably zero.
I then alert NASA that this sentence isn't cited to any other source:
Robert Walker: This sentence is not cited. However ... [I then go on to summarize some of the material in the 2009 report]
(NASA, 2023. Mars Sample Return final PEIS : B-68)
Here they are quoting from my attachment: (Walker, 2023. So many serious mistakes in NASA's Mars Samples Environmental Impact Statement it needs a clean restart) (docx) : 41)
In their comment reply to me shown in the screenshot, NASA tells me this is a conclusion it reach from arguments within the Environmental Impact Statement itself:
NASA: The sentence cited in this comment (“The relatively low probability of an inadvertent reentry combined with the assessment that samples are unlikely to pose a risk of significant ecological impact or other significant harmful effects support the judgement that the potential environmental impacts would not be significant.”) is a NASA conclusion based on the analyses presented in the PEIS
—the reference is the PEIS itself. Based on the credible scientific evidence cited in the PEIS (samples are unlikely to pose a risk of significant ecological impact), it is reasonable to conclude that there would be no significant impacts from the Proposed Action.
The term “unlikely” accounts for the fact that the risk is not zero.
(NASA, 2023. Mars Sample Return final PEIS : B-69)
So, this conclusion that "that the potential environmental impacts would not be significant" is a novel deduction by NASA rather than a conclusion from the previous planetary protection literature. NASA doesn't contest what the National Research Foundation said in 2009, that the risk of large-scale effects appears to be low but is not zero. Instead it uses this premise to deduce that any environmental effects would not be significant.
However, this reasoning NASA presented to me is not a valid risk assurance deduction from this premise.
To see why this deduction is invalid, you can say of a typical house that this house is unlikely to pose a risk of a significant house fire, but it is not reasonable to conclude that there will be no significant fire in this house. There is a low risk of a house fire in a typical house, which is why we need precautions to protect against it.
It may help to do a logical analysis:
NASA's premise:
"samples are unlikely to pose a risk of significant ecological impact"
NASA's deduction from this premise:
"there would be no significant impacts" [NOT VALID]
There I quote directly from its reply to me as shown in the screenshot. The analogy of a house fire may help to see why this reasoning isn't valid.
It is not valid to deduce from the premise:
this house is unlikely to go on fire
to the conclusion
there would be no significant impacts from house fires in this house [NOT VALID]
That is because it is not reasonable to deduce "no significant impacts" from a low risk of significant impacts.
It may help to clarify the reasoning to see what happens when we replace "significant" by "global" as an example of a potential scenario from a Mars sample return mission.
From the premise:
"samples are unlikely to pose a risk of global ecological impact"
the conclusion is
a global impact is possible but low risk [VALID]
Not
"there would be no global impacts" [INVALID]
Similarly, NASA can logically conclude from the 2009 NRC statement:
that the potential environmental impacts are unlikely to be significant [VALID].
Analogously to:
this house is unlikely to go on fire [VALID]
But it can't logically get from the NRC's statement to its conclusion
that the potential environmental impacts would not be significant [NOT VALID]
It IS reasonable to set a target probability, indeed this is standard risk assurance
This is the normal way to handle lab leaks, and other risks we can't reduce all the way to zero.
With normal risk assurance the first thing we need to do is to decide what is the appropriate target probability, bearing in mind the effects of the worst case scenarios we need to avert.
EPA's air office uses target probabilities between 1 in 10,000 and 1 in 10 million depending on the situation. See the Supplementary information section below:
We have never before encountered a situation where the risk is introduction of novel alien biology to Earth.The closest to this may be synthetic biology. Synthetic biologists have discussed target probabilities as low as 1 in 100,000,000,000,000,000,000 for synthetic life e.g. mirror life because of the magnitude of the effect of an irreversible change to Earth's biosphere in their worst case. They use multiply redundant ways to make sure that synthetic life can't escape the laboratory, many of them based on designing their synthetic biology so it can't reproduce in the wild in multiply redundant ways that it can't evade through evolution. See the Supplementary information section below:
As we saw, the ESF set a target probability of 1 in a million of release of a single Gene Transfer Agent sized particle in the lifetime of the facility.
Those are ethical decisions. If, for instance, NASA engineers do decide a target probability, they have to do it as ethicists rather than engineers, making decisions for others about ethically acceptable levels of risk. This is one of the reasons planetary protection experts have said all along that the public need to be involved at an early stage. All the major studies of planetary protection for a Mars sample return say that the general public and scientists (outside of NASA) must be broadly in agreement about what counts as acceptable levels of risk for success of this research effort. See above:
If NASA follows the processes of planetary protection as done in the past, it also needs an updated study of planetary protection for a Mars sample return. The one 14 years ago from 2009 can't be considered still adequate today because of all the new research since then. Also if it uses the normal process for deciding the level of assurance it needs to do the update recommended by the ESF study in 2012 which certainly is needed by now a decade later. Also, as recommended by the ESF in 2012, it needs to involve the public to achieve that necessary broad acceptance. This could lead to a new level of assurance required as well as a new size limit. See below:
As we will see, it is possible to sidestep all these ethical issues legitimately. That is where my miniature lab above GEO comes in. It is one way to avoid the need to make such decisions by eliminating the risk to no realistic risk not even at the levels that concern synthetic biologists if we choose appropriate levels of sterilization for any samples returned to Earth. See the Supplementary information section below:
- ⸽ The life detection lab above GEO achieves no realistic risk to Earth's biosphere, so is resilient to any future change in recommended levels of assurance
- The ESF in 2012 ruled out the prohibitory precautionary principle, because it was asked to find a way to return unsterilized samples, but a study with a broader mandate might require no appreciable risk for unprecedented harm
f NASA adopts a solution like that, with no samples returned unsterilized to Earth's biosphere with this mission and if it never returns them unsterilized until it has a clear idea of what is in them by way of alien biology, if any, it doesn't need to set a target probability for release of unsterilized samples and it doesn't need to work on risk assurance. It still needs to engage with the public and to ensure there is broad acceptance of its proposal and it needs to ensure that the method of sterilization used has broad acceptance as a way to keep Earth's biosphere safe. See below:
However with the current approach NASA does have to do risk assurance and it is clear that NASA's current team doesn't include experts in risk assurance as it is normally done.
NASA do have experts on risk assurance but for some reason haven't added that expertise to this team. That is clear already from the invalid reasoning discussed in this section. It is also clear from the way they focused on best case rather than worst case scenarios as we saw above:
This lack of expertise is also clear from their confused answers to one of their own risk assurance experts, Chester Everline who is co-author of their handbook on probabilistic risk assurance. They were clearly out of their depth, making responses that didn't address the major issues he raised in his questions They still have no target probability but they made no change to the PEIS to change the material he as an expert found confusing. They still don't say in the PEIS that there is no campaign level probability or try to justify this approach in the PEIS. See Supplementary information under
Similarly the deduction we are discussing here from a low risk of significant ecological impacts to no significant impact is novel reasoning by NASA itself. Presumably due to lack of familiarity, NASA's team has produced reasoning it found plausible but is in fact invalid as a way to reason about risk. This is another example shows NASA didn't ensure disciplines of preparers were appropriate for issues identified in the scoping process.
The disciplines of the preparers shall be appropriate to the scope and issues identified in the scoping process
§ 1502.6
See Supplementary information under :
NASA clarified in a reply to me that in their view this novel reasoning fulfills their requirement under NEPA to consider low risks of catastrophic effects.
This is the complete section of that part of their reply.
When I challenged NASA on this, it replied correctly that NEPA doesn't require a "worst-case" analysis and it also quotes a NEPA passage that says an agency as to include impacts that have catastrophic consequences even if the probability of occurrence is low.
However they think they have fulfilled this requirement.
It then continues with NASA's novel deduction of no significant impact from a low risk of significant impact which we just looked at.
This is clearly intended as their answer to the NEPA requirement to consider catastrophic consequences even if their probability of occurrence is low, if they are supported by credible evidence not based on pure conjecture and within the rule of reason:
(d) For the purposes of this section, “reasonably foreseeable” includes impacts that have catastrophic consequences, even if their probability of occurrence is low, provided that the analysis of the impacts is supported by credible scientific evidence, is not based on pure conjecture, and is within the rule of reason.
§ 1502.21 (c) and (d)
But their response to this requirement isn't valid reasoning, to deduce no significant impact from a low risk, and so to escape the need under NEPA to consider low risk catastrophic impacts by deducing that low risks are not significant. If this was valid reasoning the NEPA requirement in § 1502.21 wouldn't be needed, because this same reasoning from a low risk of catastrophic consequences to no catastrophic consequences could be applied to any situation that falls under that section.
Then they don't seem to have noticed my use of the National Academy of Sciences as the source for the assessment of a low risk of catastrophic effects.
Robert Walker: However in the discussion of large scale effects, the 2009 National Research Foundation study they use as a source elsewhere says that it is simply not possible to discount such effects in the distant past from Martian life transferred to Earth. (Board et al, 2009:48):
Here NASA
NASA: While 40 CFR § 1502.21(d) defines “reasonably foreseeable” as including impacts that have catastrophic consequences, even if their probability of occurrence is low, the analysis of the impacts must be supported by credible scientific evidence, must not be based on pure conjecture, and must be within the rule of reason.
(NASA, 2023. Mars Sample Return final PEIS : B-68)
At least NASA never refer to the National Academy of Sciences reasoning from 2009 in their reply. This book looks at their reasoning in detail along with quotes from other recent Mars sample return studies to compare, the 1997 study from the NRC and the 2012 study from the ESF. See the end of the section discussing the Great Oxygenation Event as an illustration of what in principle a single novel species of microbe could do to the biosphere of a planet (below):
The studies by the National Academy of Sciences and the European Science Foundation deduce a potential for large-scale harmful effects from microbes from Mars supported by credible scientific evidence. These deductions are of the highest scientific integrity and within the rule of reason. So they have to fulfill the NEPA requirements, if this doesn't fulfill the requirement what ever could?
So no, it doesn't seem that NASA fulfills the NEPA requirements here. The NEPA requirements are there for a reason. NASA has failed them, so it's PEIS is not adequate and this book concludes that it needs to be withdrawn. It doesn't recommend any legal action against NASA which seems likely to be counterproductive but rather either a call from independent review of NASA's plans by the ESF or NRC or both, or for NASA to withdraw the PEIS from its own side to find another way ahead.
For details of why NASA's analysis here fails NEPA requirements in § 1502.21 see below:
Previously, we saw that NASA's other arguments which lead to a conclusion of a near zero risk were also invalid, based on major failings of scientific integrity rather than any new research.
- NASA's four apparently plausible findings already rebutted in 2000
[and following sections]
Now we see that they are also using invalid reasoning in this final step from near zero risk to no catastrophic effects, which they needed to comply with§ 1502.21 and which as we saw above they also needed to reach the conclusion that they could restrict the affected location in tier 1 to the Utah Test and Training Range, see above:
Interestingly, NASA's team doesn't use this novel reasoning in its responses to members of the public who raise concerns of unprecedented harm. Instead they just agree with them that there is a risk of large-scale harm, and say the risk is likely low but non zero, and say this is why they have to contain the samples.
NASA's standard response to public comments raising concerns of unprecedented levels of global harm from this mission, even potential for mass extinction: "the risk cannot be demonstrated to be zero" jars with their conclusion in the main body of the statement that the potential environmental impacts would not be significant and any public health impact negligible
First it's important to say that there is no particular reason to suppose that this is an issue unique to NASA. NASA has previously been seen as the space agency globally that is most careful about planetary protection. We don't have any environmental impact statements from other space agencies yet to compare this PEIS to. Other agencies might use similar arguments. Any such arguments will be examined closely once the wider public look at the biosafety plans in detail proposed by space agencies.
In the main body of the PEIS we saw that NASA argues that the PEIS that the potential environmental impacts would not be significant.
The relatively low probability of an inadvertent reentry combined with the assessment that samples are unlikely to pose a risk of significant ecological impact or other significant harmful effects support the judgement that the potential environmental impacts would not be significant.
(NASA, 2023. Mars Sample Return final PEIS : 3-16)
This is not standard risk assurance, and though it seemed plausible to NASA's team, it is not valid reasoning. It's like arguing that a house fire is not significant because the risk is low see above:
However they don't use this reasoning in response to the public comments. Instead they simply respond to all these concerns no matter how severe they are, saying "the risk cannot be demonstrated to be zero".
The main concerns raised by the public were similar to the concerns Sagan raised, a risk of large-scale or even unprecedented impacts on public health or the biosphere. This is one example of many:
I am extremely concerned that this proposed action could potentially contaminate native life forms on Mars and/or bring back alien virus, bacteria, or other life forms from Mars to Earth.
I understand that there are planetary protection protocols. However, Murphy's Law says that if something horrible could happen, it eventually may indeed occur. History is filled with examples where Acts of God and/or human arrogance caused otherwise unforeseen disasters.…
The Earth is already dealing with increasingly serious problems from invasive or alien species being transported to new locations, and viruses mutating and causing deadly pandemics. We have not been able to solve many of these problems.
What happens if a Mars life form escapes containment and, without evolving in Earth's ecosystems, spreads uncontrollably and devastates Earth's species including us humans? There might be no way to reverse or even mitigate for that devastation.
I support scientific research when it is safe and in the public interest. However, I oppose research when there is no absolute guarantee of safety and when the risks outweigh the potential benefits.
(Spotts, 2022, Comment Submitted by Richard Spotts)
I provide direct links to all the comments submitted in the final round of public comments with a brief summary of the level of concern for each one here:
NASA's response to Spotts was:
"Refer to the previous response for HS-002"
(NASA, 2023. Mars Sample Return final PEIS : B-5)
HS-002 is their answer to another similar question from Greger:
Greger:Are you certain that in any way, this mission won’t end with the total annihilation of the entire planet, or force us to live in biomes for the rest of time?
NASA: As discussed in Section 3.2 of the PEIS, the exact nature of the Mars sample constituents regarding biosignatures and potential biological activity is currently unknown.
The PEIS cites several sources supporting the position that contamination of Earth by Martian microorganisms is extremely unlikely to pose a risk of significant harmful effects. However, the risk cannot be demonstrated to be zero (see Response ID HS-001 for information regarding containment measures).
As a result, a comprehensive quantitative analysis of the potential impacts of a sample release in the event of an off-nominal landing and the effects of Mars samples on Earth’s environment cannot be accomplished with current data; any such analysis would be theoretical at best, involving substantial speculation and supposition. For this reason, the emphasis of the MSR approach is on sample containment(NASA, 2023. Mars Sample Return final PEIS : B-43)
So even in response to a member of the public who asked NASA if it is possible that one consequence would be that we have to live in biomes on Earth for the rest of time or total annihilation of the planet (presumably meaning extinction of all terrestrial life) NASA were not able to rule this out as a possible consequence of their mission.
Instead NASA responds by saying that the emphasis is on sample containment, since they can't predict consequences if the samples are not contained. But in that case how can the Mars Receiving Facility be tier 2?
As we saw at the start, expert opinion is that the risk of such scenarios is very low, and the analogy of a house fire and a smoke detector fits them well. But we take great care to protect our houses from the very low risk scenario of a house fire. See above:
So in its replies to the public comments NASA seem more aligned with what Mars sample return studies say than in its conclusions within the PEIS itself.Let's use the technique of illustrative scenarios in the interest of keeping these discussions grounded on science rather than abstract ideas or science fiction
The closest I've found to the possibility of living to the end of time in biomes is the likely ultra low-risk scenario of life on Mars independently evolved from mirror organics and able to use ordinary organics mentioned above.
This wouldn't happen instantly but it would be a permanent change from our current biosphere to a biosphere with a mix of ordinary and mirror life. As mirror life radiates and spreads through Earth's biosphere, we'd then need to work to rescue our ecosystems and after trying many other solutions, in the worst case we might find the only viable long term solution is to use large dome-like biomes covering our forests, coral reefs, etc.
In the worst case, if we can't modify most terrestrial life to make use of the mirror organics in the soil, water and oceans, possibly after decades or centuries for the life to radiate through our ecosystems, we might need barriers in the air, soil and sea, and need to take measures to sterilize the interiors of mirror life, and find ways to keep it out. This might well be for a future centuries from now, with technological capabilities we can't imagine at present, but it is a legacy we wouldn't want to leave to future generations.
Using a scenario like this rather than abstract ideas may help to make it clear that a non zero though likely ultra low risk of large-scale harm to Earth's biosphere such as this, which could lead to humans having to live in biomes for the rest of time is NOT identical to NASA's conclusion in the PEIS that any environmental effects would not be significant.
Chester Everline, a co-author of NASA's handbook on probabilistic risk assurance (NASA, 2011, Probabilistic risk assessment procedures guide for NASA managers and practitioners) who commented on the last day of public comments put it like this:
Chester Everline: Given our lack of scientific insight into possible life on Mars, relics of life we may return from Mars, or simply organic substances from Mars that could interact with certain life forms on Earth, how can we possibly assert with confidence that MSR [Mars Sample Return] poses an acceptable risk to Earth's biosphere, even if the incredibly difficult target of a 99.9999% target for successful containment is satisfied? Given that sample return missions of the type proposed for MSR have never been attempted before, is it even feasible to do enough testing to assure that a 99.9999% target can be achieved?
(NASA, 2023. Mars Sample Return final PEIS : B38)
NASA responded to him as they did to Spotts, and to myself and anyone else who asked similar questions referring him back to their response to Greger.:
Please see Response IDs HS-001 and HS-002 regarding risks to Earth’s biosphere and NASA’s approach to addressing that. With regards to the assurance case (HS- 017), no outcome in science and engineering processes can be predicted with 100% certainty. NASA’s extensive testing activities serve to support the assurance case
(NASA, 2023. Mars Sample Return final PEIS : B38)
As we saw above, NASA's statement "no outcome in science and engineering processes can be predicted with 100% certainty" is not actually a valid premise.
In this case we can predict with 100% certainty that if NASA doesn't return the samples that Perseverance is currently caching on Mars, there is no risk to Earth;s biosphere or inhabitants from them. We can also achieve essentially 100% sterilization by thoroughly sterilizing any samples that contact Earth's biosphere.
We are not required to take ANY risks with Earth's biosphere. Whether to take such a risk isn't a technical decision for engineers to make for us using reasoning like this. The methods of reasoning used in science and engineering can't be applied to questions like this, so they are left trying to use scientific-like reasoning to deal with moral issues. It doesn't work; there's a mismatch between the logical tools they use and the subject they apply them to. We see this throughout the PEIS and the responses to the public comments.
As the theologian Richard Randolph put it, it isn't a technical question for scientific reasoning, it is a moral question concerning accepting risk.
Without being overly dramatic, the consequences might well include the extinction of species and the destruction of whole ecosystems. Humans could also be threatened with death or a significant decrease in life prospects
In this situation, what is an ethically acceptable level of risk, even if it is quite low? This is not a technical question for scientists and engineers. Rather it is a moral question concerning accepting risk
(Randolph, 2009, Chapter 15, God’s preferential option for life: a Christian perspective on astrobiology : 292)
We go into this question in more detail in the Supplementary information, see:
- Worst case scenarios introduce novel ethical and legal questions – is a 1 in a million level of risk acceptable for a scenario that could adversely affect the biosphere of Earth in the very worst case?
[and following sections]
Chester Everline continues:
Does NASA intend to impose a threshold for acceptable risk (i.e., a value above which the mission is considered too risky to proceed)?
A possible consequence of unsuccessful containment is an ecological catastrophe. Although such an occurrence is unlikely, NASA should at least be clear regarding what level of risk it is willing to assume (for the biosphere of the entire planet)
NASA's reply was that they aren't required to set such a target probability and don't plan to do so. See Supplementary information under
They are not legally required to set a target probability. However many in the general public may not be satisfied by these response and wish to look for alternatives that don't have even the smallest chance that our descendants may have to live in biomes for the rest of time.
That is the main focus of this book, to look for alternatives that don't depend on our ability to contain life of unknown properties and capabilities, focusing on the alterative of a miniature life detection lab above GEO as one possible way to do it worked out in some detail.
We find that this is a solution that NASA could adopt which doesn't require them to produce a biosafety plan or to engage in this ethical and moral reasoning with issues of acceptable levels of risk.
- Miniature life detection lab above GEO: a teleoperated alternative that plays to NASA's strengths, modelled on its proposed in situ Europa life detection lander
- This proposed alternative submitted to NASA under NEPA uses a centrifuge for Mars gravity in an excellent Mars simulation chamber, which also means instruments can operate under Mars gravity
- Modern miniature life detection instruments that could fly to above GEO, or to Mars
NASA's response also doesn't include any attempt to use analogies like my suggestion of a smoke detector metaphor (which I suggested they use in my public comment) or risk maps, or any other method of helping the public to try to appreciate what is meant by a low risk here. It also doesn't have any attempts to involve the general public in the decision making and risk governance.
NASA's current team are not aware of any lack of expertise in this area of public communication and dialog on risk governance. Their response to my comment alerting them to this issue is that this requirement is satisfied by NASA's membership of international organizations like COSPAR that have some team members with the necessary expertise.
With the alternative suggested here, there is much less need for NASA, other space agencies and private space companies to engage with the general public on risk governance though there is still a need to work on a globally acceptable level of sterilization for any samples returned to Earth.
Some readers may be interested to jump ahead to the conclusions to see where the reasoning in this book is headed at this stage before continuing:
Why NASA can't ensure negligible public impacts from alien microbes using standard biosafety level 4 procedures: how a biosafety lab differs in requirements from a Mars Receiving Facility
If it is agreed we do need to contain the samples and that there is a risk of large-scale harm as the worst case, the next question is, how do we do it? The ESF study in 2012 says it is reasonable to assume that the Sample Receiving Facility is the most likely cause of an accidental release:
Based on failure of containment of pathogenic material in the past, it is reasonable to assume that the most likely cause of a release would be due to human error or a deliberate human act following the introduction of the material into the laboratory.
(Ammann et al., 2012, Mars Sample Return backward contamination–Strategic advice and requirements 33)
As we will see, NASA's team is confident it can contain any hazard from alien life in the samples using standard Biosafety Level 4 procedures designed to contain known infectious diseases.
However if we go to the planetary protection literature, a Mars Receiving Facility has novel design and operational requirements we never need for terrestrial diseases. The Mars Receiving Facility takes up 10 out of 80 pages of the National Academy of Sciences study (SSB, 2009, Assessment of planetary protection requirements for Mars sample return missions : chapter 7). Also this facility is the main focus of the 45 page 2012 European Science Foundation study. Most of its discussion is about how it came to its recommendations on the size limit and level of assurance for a Mars Receiving Facility, which as we've seen go well beyond capabilities of a BSL-4 laboratory (Ammann et al., 2012, Mars Sample Return backward contamination–Strategic advice and requirements : 24). We cover this below in:
- Why NASA can't rely on HEPA filters for the ESF recommendation to contain 100% of ultramicrobacteria
All the main studies are also clear on the potential for global harmful effects on human health, although with a likely very low level of risk.
NASA doesn't consider the design for the Mars Receiving Facility in this PEIS. Instead, the PEIS relies on biosafety level 4 labs designed by other agencies and their final Environmental Impact Statements to conclude that "the risk to the public [from lab leaks] is negligible":
While not completely analogous, the results of previous NEPA analyses for BSL-4 facilities have concluded that the hazards associated with the operation of BSL-4 facilities are expected to be minimal.
…
Analyses performed in support of recent NEPA documents [by other agencies for previous BSL-4s] conclude that the risk from accidental release of material from a BSL-4 even under accident conditions that include the failure of protective boundaries (e.g., reduced effectiveness of ventilation filtration systems) are minute and can be described as zero (NIH/DHHS 2005).
An alternative release path resulting from the contamination of workers leading to direct contact with others (members of the public) was also analyzed [Not analyzed by NASA and not analysed for potential alien life]. Qualitative risk assessments for this mode of transmission [for the two previous EIS's for ordinary BSL-4 labs] have shown that the risk to the public [from lab leaks] is negligible (NIH/DHHS 2005, DHS 2008).
(NASA, 2023. Mars Sample Return final PEIS : 3-14)Cites:
NIH/DHHS. (2005) = (NIH, 2005. Final Environmental Impact Statement, National Emerging Infectious Diseases Laboratories)DHS. (2008) = (DHS, 2008. Final Environmental Impact Statement for the National Bio and Agro-Defense Facility with later supplements listed in (NBAF, n.d., Document Library) )
------------------------------This deduction is for terrestrial life with known containment methods.
The big issue for alien life is that it would be unknown and it would require an unknown quarantine period which could be as long as decades, which Carl Sagan refers to as "the vexing question of the latency period" not looked at here.
This also doesn't consider containment for ultramicrobacteria which don't need to be contained in BSL-4 facilities but do need to be contained for alien life.
[My comments in RED]
The PEIS says the design for the Mars Receiving Facility is for discussion later in tier II, which is normally intended for matters of less environmental consequence than tier 1. That is what is meant by this sentence in the abstract.
Also in the abstract:
Future tiered analyses are planned to address site-specific impacts associated with sample transportation and development and operation of an SRF.
(NASA, 2023. Mars Sample Return final PEIS : 1)
It says little more about this. It just says that Tier II NEPA analsysis would be simlar to those for existing BSL-4s.
Should the Proposed Action be chosen, Tier II NEPA analyses of the proposed SRF would include analysis similar to those performed for existing BSL-4 facilities.
(NASA, 2023. Mars Sample Return final PEIS : 3-14)
None of these biosafety labs are designed to contain unknown pathogens from another planet with unknown capabilities and possibly also based on non terrestrial biology.
NASA may have been influenced here by the Biological Safety Report's finding of near zero risk of any martian pathogens, which however doesn't match other assessments in the literature.
Also a Mars Receiving Facility, unlike a biosafety lab, needs to be designed as a clean room to protect the samples from contamination by terrestrial life.
The team trying to open the Bennu asteroid samples face this same issue. They were able to collect some material that got onto the outside of the container but have not been able to open it because of the crash landing of the probe, which led to two of the fasteners getting jammed. The procedures are complicated by the need to keep the sample pristine by handling with gloves in a nitrogen atmosphere.
After multiple attempts at removal, the team discovered two of the 35 fasteners on the TAGSAM head could not be removed with the current tools approved for use in the OSIRIS-REx glovebox. The team has been working to develop and implement new approaches to extract the material inside the head, while continuing to keep the sample safe and pristine
All curation work on the sample – and the TAGSAM head – is performed in a specialized glovebox under a flow of nitrogen to keep it from being exposed to Earth’s atmosphere, preserving the sample’s pristine state for subsequent scientific analysis. The tools for any proposed solution to extract the remaining material from the head must be able to fit inside the glovebox and not compromise the scientific integrity of the collection, and any procedures must be consistent with the clean room’s standards.
(NASA, 2023. NASA’s OSIRIS-REx Achieves Sample Mass Milestone)
Any terrestrial Mars Receiving Facility will require clean room handling like that, but at the same time combined with containment in the other direction to prevent anything from the samples reaching Earth's biosphere. The situation is far simpler if the material is returned to a robotic satellite above GEO as the barrier of space both protects the samples and Earth, the solution proposed in this book as one way to do this with zero risk to Earth's biosphere. See below.
Our asteroid samples such as the ones from Bennu don't need to be contained to protect Earths biosphere, is not necessary with Bennu because the samples are safe by Greenberg's “Natural contamination standard” (Greenberg,et al., 2001. Macroscope: Infecting Other Worlds). Earth has a much larger continuous natural influx of similar material, a similar argument to the same reasoning for Phobos see below: /p>
- No concerns for Bennu or other Near Earth Objects because of natural contamination standard - and no other sample returns that might need protection until Ceres, Enceladus or Europa not likely until the 2040s
- Any life in martian meteorites DOES get here better protected and faster than in samples returned from the top two centimeters of the MOONS of Mars: Phobos or Deimos
But samples from Mars will need to be both contained, to protect Earth's biosphere, and also kept free of contamination by terrestrial life as much as possible to protect the scientific value of the samples.
This clean room requirement is hard to combine with sample containment. As an example,
- keeping the samples under negative pressure helps contain the samples,
- positive pressure helps keep them clean
The literature has a great deal of discussion of novel designs to combine those two normally conflicting requirements into one coherent design. The three main designs proposed for a Mars sample receiving facility consist of (Uhran et al., 2019, Updating Planetary Protection Considerations and Policies for Mars Sample Return : table 2):
- a clean room inside a biosafety level 4 facility
(personnel not well protected from samples) - a biosafety level 4 facility inside a clean room
(samples not well protected from personnel and terrestrial life), - a biosafety level 4 facility surrounded by a vacuum barrier inside a clean room in a novel triple wall facility
(protects both sample and personnel)
(Figure 4 of (Carrier et al., 2019. Science-Driven Contamination Control Issues Associated with the Receiving and Initial Processing of the MSR Samples) adapted from (Rummel et al., 2002, A draft test protocol for detecting possible biohazards in martian samples returned to Earth : Figure 1)
The last of those three is the only one that protects both the personnel and the samples optimally and involves a novel triple wall structure which has never yet been built (Carrier et al., 2019. Science-Driven Contamination Control Issues Associated with the Receiving and Initial Processing of the MSR Samples) adapted from (Rummel et al., 2002, A draft test protocol for detecting possible biohazards in martian samples returned to Earth : Figure 1)..
NASA's 2022 sketch for a Sample Receiving Facility: assumes samples are rapidly shown safe to remove unsterilized and leaves considerations of biocontainment for tier 2, outside expertise of MSPG2 members - as for all its studies prepared in 2022
This is the only sketch I can find for the proposed MRF (Carrier et al., 2022. Science and Curation Considerations for the Design of a Mars Sample Return (MSR) Sample Receiving Facility (SRF) : S-223).
One can't conclude much else from this sketch or this report which is focused on the science and doesn't discuss biosafety or how the biocontainment is done. It is not even clear which of the three methods of containment in the literature NASA plans to use. Are the samples under positive pressure to protect them from terrestrial contamination? Or are they under negative pressure to protect the technicians? Or will they use a novel 3-wall configuration to protect both, which so far is theoretical and never used in practice?
For these three methods see above:
The authors say the design is based on an assumption that the samples are soon proven to be safe to remove unsterilized.
It is important to note that the planning described above is for a minimalist facility, based on the contingency that no martian biohazards are present in the samples. In this scenario, it is assumed that unsterilized samples would be able to be transferred to uncontained facilities after they have been determined to be non-hazardous. The instruments in the SRF would be a small subset of all the instruments that would eventually be applied to the samples. However, in the unlikely scenario where martian life or a non-living bio- hazard is discovered, at least some unsterilized samples would need to stay in the SRF indefinitely, and a somewhat broader set of instrumentation would need to be brought to bear.
(Carrier et al., 2022. Science and Curation Considerations for the Design of a Mars Sample Return (MSR) Sample Receiving Facility (SRF) : S-232)
However as we'll find out, it won't be possible to prove that the samples are safe to be removed unsterilized with these samples With the proposed safety assessment framework they will all go to "Hold and Critical Review" which can likely only be resolved through further in situ study on Mars. See the sections below:
through to
Earlier in the PEIS NASA explains that a BSL-4 is the highest level of containment and that NASA might build a new BSL-4 lab or modify lower level labs to be equivalent to BSL-4 for the purposes of the samples:
The specific requirements for an SRF are currently in development; however, this PEIS applies BSL-4 equivalent facility protocols as being representative of construction and operating standards that may be adopted in the future to manage the storage and curation of Mars samples. As a result, analysis of potential impacts associated with development and operation of an SRF are identified and analyzed programmatically in this PEIS. By applying the BSL-4 framework, NASA is able to identify and analyze reasonably foreseeable environmental impacts of its Proposed Action (e.g., the air emissions from a representative existing BSL-4 facility) and evaluate, from a programmatic perspective, whether the environmental effects may significant.
… Since BSL-4 provides the highest level of containment, the scope of any potential SRF assumes BSL-4 equivalency as a minimum requirement; however, modification or updates to other lower-level BSL facilities to achieve equivalent BSL-4 containment may be potential alternatives for consideration in the development of a proposed action and alternatives under Tier II analysis.
: 2-15 to 2-16)
However as we have just seen ALL the main Mars sample return studies explain that BSL-4 labs are NOT representative of a Mars Sample Receiving Facility. NASA's team shows no awareness of these studies in its PEIS. At least, the PEIS doesn't reference the literature on the design requirements for a Mars Receiving Facility, just the literature on BSL-4 labs.
All NASA says about the Mars Receiving Facility design is:
NASA’s concept for the SRF is to build a facility that can be characterized as a BSL-4 equivalent facility. The facility would nominally incorporate the designs and procedures of a BSL-4 facility (which has significant security requirements) and possibly, as yet undefined, additional cleanliness and protective measures
(NASA, 2023. Mars Sample Return final PEIS : 3-13)
Those extra "cleanliness and protective measures" increased the cost more than four-fold in the designs submitted to the 2010 decadal review. They also lead to a long timeline of 13 years to complete the labs due to the novel design requirements and the need to train the staff in how to operate them. We look at this in more detail below:
This is not a simple matter. The 2009 study anticipated that the design, construction and operation of the facility would require coordination of multiple teams of experts over a decade or more of planning. It also said that the facility would need continuing interagency advice and oversight from the CDC and others. It says we need to make sure to avoid the mistakes of Apollo when planetary protection concerns and considerations were often overruled or ignored when they conflicted with other aspects of mission operations.
As already noted, the design, construction and operation of the SRF [Sample Return Facility] will require the coordination and work of multiple teams of experts, spanning a decade or more of planning. It will be important for various layers of scientific and technical oversight to be in place early in the planning process to ensure continuity through the lengthy and complex Mars sample return mission planning process.
In addition to the establishment of a body to provide scientific and technical advice relating to an SRF, there is also a need for higher-level oversight of all planetary protection requirements associated with Mars sample return. It is clear to the committee that NASA will need to obtain continuing interagency advice (e.g. from the Centers for Disease Control and Prevention and relevant biosecurity agencies and organizations) on planetary protection compliance, ...
Indeed, the history of the Apollo program, for example, is replete with occasions on which planetary protection concerns and considerations were overruled or ignored when they conflicted with other aspects of mission operations. Appropriate organizational arrangements should be made to avoid such conflicts.Recommendation: Because of the lengthy time needed for the complex development of a sample-receiving facility (SRF) and its associated biohazard-test protocol, instrumentation, and operations, planning for an SRF [Sample Receiving Facility] should be included in the earliest phases of the Mars sample return mission.
(SSB, 2009, Assessment of planetary protection requirements for Mars sample return missions : 67 - 68)
By that recommendation in the 2009 Space Studies Board study for planetary protection for a Mars Sample Return mission, NASA should have stated work on the design of the facility at the earliest phases of its planning for the Mars sample return mission over a decade ago to give them plenty of time to work on the difficult technical requirements of the facility and train staff in appropriate protocols.
Instead, as we saw, in this final PEIS published in 2023, NASA defers the planning to tier II of their PEIS, with work starting later. Also they are of the view they can use standard protocols of a biosafety lab, a view not supported by the literature with all the studies saying new protocols will be needed. See above:
- NASA's novel conclusion that any effects would be local to the Utah Test and Training Range, and not significant
- Impact of limited scope for tier 1: NASA only publicised its PEIS and scoping meetings in newspapers local to the Utah Test and Training Range and Kennedy Space Center
The authors of the 2022 paper on the Sample Receiving Facility (SRF) make it clear that biosafety and biocontainment is not their area of expertise and would need to be addressed later .
Beyond the scientific capabilities outlined above, there are myriad building and engineering requirements needed to complete the design of the SRF and thus support the overall science goals of MSR. The specifics of these requirements are outside the expertise of MSPG2 members and are thus incomplete in their identification in the proposed SRF Design Requirements table and in the discussion sections below. The building and engineering requirements needed to complete the design of the SRF should be addressed by the SRF implementation team and subsequent building contractors.
...
Current draft requirements state that samples returned from Mars would be placed in BSL-4 equivalent containment until they are deemed safe to be released to outside laboratories, either by analysis or by sterilization. ...
(Carrier et al., 2022. Science and Curation Considerations for the Design of a Mars Sample Return (MSR) Sample Receiving Facility (SRF) : S-232)
By MSPG2 they are not just referring to the authors of this particular report. It means there was nobody in the MSPG2 team that prepared the other reports in 2022 in preparation for the PEIS who had expertise in biosafety or biocontainment.
They plan this as a minimalist facility to hold the samples until they are proven to be safe. It would be expanded if martian life is found.
It is important to note that the planning described above is for a minimalist facility, based on the contingency that no martian biohazards are present in the samples. In this scenario, it is assumed that unsterilized samples would be able to be transferred to uncontained facilities after they have been determined to be non-hazardous.
…
However, in the unlikely scenario where martian life or a non-living biohazard is discovered, at least some unsterilized samples would need to stay in the SRF indefinitely, and a somewhat broader set of instrumentation would need to be brought to bear.
(Carrier et al., 2022. Science and Curation Considerations for the Design of a Mars Sample Return (MSR) Sample Receiving Facility (SRF) : S-232)
This suggests MSPG2 involved no experts from the CDC, DoA, NIH, etc. They lost this expertise when they closed down the interagency panel. See above:
With this background it is not surprising to find no details for how NASA plans to construct a Mars Receiving Facility for biosafety or biocontainment. It simply hadn't given the matter any consideration yet as of 2022.
The Sample Receiving Facility study has a short historical introduction which mentions earlier Sample Receiving Facility studies but without mentioning or discussing their recommendations. In particular it has
- Nothing on the size limit of particle to contain
- Nothing on the level of assurance needed
- Nothing on filter technology, what to use, how to replace them.
- Nothing on air pressure, presumably the samples would be contained under positive pressure to prevent contamination by terrestrial materials but the details are not discussed
- Nothing on the size limit or level of assurance for terrestrial contamination to be kept out of the samples.
This is the complete list of papers (Kminek et al., 2022. Mars Sample Return (MSR):Planning for Returned Sample Science) :
- Overview: Mars Sample Return Planning Group 2 (MSPG2) final report (Meyer et al, 2022, Final Report of the Mars Sample Return Science Planning Group 2 (MSPG2))
- Overview: Preliminary planning for MSR Science Program (Haltigin et al, 2022, Rationale and Proposed Design for a Mars Sample Return (MSR) Science Program)
- Science value: Work flow for science studies, assumes the samples will be proved safe to release unsterilized after preliminary assessment (Tait et al, 2022, Preliminary Planning for Mars Sample Return (MSR) Curation Activities in a Sample Receiving Facility (SRF))
- Science value: Experiments that need to be done early on, such as analysing headspace gases and the samples before they are exposed directly to Earth's atmosphere, assumes that samples will be proven safe to release unsterilized after a few months of study per sample (Tosca et al, 2022, Time-Sensitive Aspects of Mars Sample Return (MSR) Science)
- Science value: Studies related to past or present day life are sterilization sensitive and need to be done on unsterilized samples, includes a recommendation to use (Velbel et al, 2022, Planning Implications Related to Sterilization-Sensitive Science Investigations Associated with Mars Sample Return (MSR))
- Science value: Need for a dedicated atmosphere sample including risk that all the headspace gases are lost to space and outgassing from the sample tubes and sample (Swindle et al., 2022. Scientific Value of Including an Atmospheric Sample as Part of Mars Sample Return (MSR))
See below: Proposal for bonus samples collected in 100% clean containers by the NASA Sample Retrieval Lander to help tease out fine details relevant to astrobiology - Science value: On the value of dust on the outside of the tubes and in regolith samples (Grady et al., 2022. The Scientific Importance of Returning Airfall Dust as a Part of Mars Sample Return (MSR))
See below: Proposal for bonus samples collected in 100% clean containers by the NASA Sample Retrieval Lander to help tease out fine details relevant to astrobiology - Backwards contamination: A framework to investigate safety of the unsterilized samples which relies on proving there is no life in them, which also concluded that there is likely is no way with current understanding and technology to prove that life from Mars is safe if detected, so safety assessment relies on proving there is no life in the samples (Kminek et al, 2022, COSPAR Sample Safety Assessment Framework (SSAF)).
However as we'll see the relatively high levels of forward contamination in the tubes (for astrobiology) mean all the Perseverance samples will fail this preliminary assessment and go to a"Hold and Critical Review" which couldn't be resolved without more studies on Mars. We will also see that even with 100% clean containers for the bonus samples, the suggested framework, wouldn't protect against small numbers of viable microbes which might be present in dust if viable martian life can be transported through the atmosphere, or if Perseverance samples material close to a local microbial oasis on Mars
See below: Why the COSPAR Sample Safety Assessment Framework can't be used to prove safety of unsterilized Perseverance samples - Backwards contamination: Potential requirements and capabilities needed for a Sample Receiving Facility (the study their sketch of a SRF comes from) (Carrier et al., 2022. Science and Curation Considerations for the Design of a Mars Sample Return (MSR) Sample Receiving Facility (SRF) : S-223)
See below: Proposal for bonus samples collected in 100% clean containers by the NASA Sample Retrieval Lander to help tease out fine details relevant to astrobiology - Forward contamination of the Orbiting Sample Container - whether it should be cleaned to the same levels as the interior of the sample tubes or the lower level of sterilization used for the exterior of the tubes, which were just cleaned to normal clean room cleanliness, concludes that a similar level of cleanliness to the exterior of the tubes would be sufficient with an aspiration to clean it more if it is feasible in terms of engineering. (Cockell et al, 2022, Recommendation on Orbiting Sample Cleanliness)
I have not discovered any papers since then on preliminary designs for a Sample Receiving Facility.
As we have seen throughout the PEIS, the text of the PEIS shows no familiarity with the planetary protection literature on a Mars Sample Return mission for the topic being discussed, the Sample Receiving Facility. Nor does the literature prepared for the PEIS in 2022 cover this topic. Also none of the material prepared for this PEIS in 2022 seems to have involved experts in disciplines such as public health, invasive species, epidemiology, infectious diseases, biosafety, biocontainment or the design of a biosafety lab.
We see the effect of the two tier division here. All those considerations are deferred to tier II. See Supplementary information under: Fails NEPA requirement to select preparers with disciplines appropriate to the Impact Statement's scope and issues
NASA's text in the PEIS is also not taking account of the need for a facility to contain ultramicrobacteria which are beyond the capabilities of HEPA filters, a new requirement from the ESF study from 2012 which we cover in the next section.
- Why NASA can't rely on HEPA filters for the ESF recommendation to contain 100% of ultramicrobacteria
It also doesn't take account of issues of quarantine of novel pathogens which the PEIS doesn't discuss. See below:
This fails the NEPA requirement on when tiering is appropriate. Tiering is meant to help agencies focus on issues that are ripe for decision. But the issue of whether it is possible to build a Mars Receiving Facility at all with current technology and what level of containment can be achieved is an issue for tier 1 not for later discussion.
It could be suitable to use tiering if NASA is right that no changes are needed to current biosafety laboratories and if they had established there was no risk of global effects.
But in that case NASA still have a requirement under NEPA to discuss the majority consensus point of view that major developments of new technology are required for a Mars sample receiving facility and then explain why they believe this majority view is incorrect. This was not done in this PEIS.
NASA's PEIS shows no awareness of the previous literature on the issues associated with a Mars sample receiving facility designed to contain any conceivable extraterrestrial biology and unknown pathogens that may have novel modes of action. The Council on Environmental Quality in its explanation of when tiering is appropriate says:
Tiering is appropriate when the sequence of statements or analyses is:
- From a program, plan, or policy environmental impact statement to a program, plan, or policy statement or analysis of lesser scope or to a site-specific statement or analysis.
- From an environmental impact statement on a specific action at an early stage (such as need and site selection) to a supplement (which is preferred) or a subsequent statement or analysis at a later stage (such as environmental mitigation). Tiering in such cases is appropriate when it helps the lead agency to focus on the issues which are ripe for decision and exclude from consideration issues already decided or not yet ripe.
(CEQ, 2007, A citizen’s guide to the NEPA: Having your voice heard : 49)
This is especially important since potential effects of leaks from the Mars Receiving Facility are global according to all the peer reviewed studies. This makes it especially important to discuss the facility because misidentifying this as a tier 2 decision could have major impacts in the assessment of the affected area for environmental effects which risks invalidating the entire PEIS.
See below:
Why NASA can't rely on HEPA filters for the ESF recommendation to contain 100% of ultramicrobacteria
This is what NASA say:
Nevertheless, out of an abundance of caution and in accordance with NASA policy and regulations, … NASA and its partners would use many of the basic principles that Biosafety Level 4 (BSL-4) laboratories use today to contain, handle, and study materials that are known or suspected to be hazardous.
(NASA, 2023. Mars Sample Return final PEIS : S-4)
The science of 1999 did set requirements that though challenging are within the range of what could be achievable by a BSL-4 – that the probability that a single particle of 0.2 microns or larger is released into Earth’s environment is less than 1 in a million
The starting point of this activity was the requirement used since the late 1990s specifying that: ‘the probability that a single unsterilised particle of 0.2 micron diameter or greater is released into the Earth environment shall be less than [one in a million] ’.
…
The value for the maximum particle size was derived from the NRC-SSB 1999 report "Size Limits of Very Small Microorganisms: Proceedings of a Workshop", which declared that 0.25 ± 0,05 μm was the lower size limit for life as we know it (NRC, 1999)
(Ammann et al., 2012, Mars Sample Return backward contamination – Strategic advice and requirements : 3)
However, the ESF in 2012 updated this to a recommendation for the Mars Receiving Facility to contain all particles of 0.05 microns and upwards to keep out ultramicrobacteria after a discovery that these can pass through 0.1 micron nanopores.
"The release of a single unsterilized particle larger than 0.05 μm is not acceptable under any circumstances"
(Ammann et al., 2012, Mars Sample Return backward contamination - Strategic advice and requirements : 48).
They also said that the probability of release of a single particle of 0.01 microns or less needs to be less than one in a million. Not a million fold reduction, a 1 in a million probability of release of a single particle of that size in the lifetime of the facility.
This was after a discovery that the very small gene transer agents can transfer novel capabilities to distantly related archaea far more rapidly than previously thought, overnight in sea water.
However this requirement could be relaxed to a figure larger than 0.01 microns, if expert review determined that a larger lit is the best size that can be achieved at resonable cost and if expert review decides release of such very small particles is tolerable, after independent review.
The probability that a single unsterilized particle of 0.01 μm diameter or greater is released into Earth's environment shall be less than 10-6 [less than one in a million]
If the size requirement cannot be met with out decreasing the overall level of assurance for the non-release of such a particle, the release of a single unsterilised particle of up to 0.05 μm can be considered as a potentially tolerable systems-level adjustment, assuming that it has been demonstrated that this size is the lowest achievable at a reasonable cost.
In such a case, the actual maximum particle size potentially released (as planned from design) would have to be independently reviewed by interdisciplinary groups of inter- national experts to determine:
- whether this size value is the best reasonably achievable at a reasonable cost,
And, if yes
- taking into consideration the latest scientific developments in the fields of astrobiology, microbiology, virology and any other relevant discipline, whether the release of such a particle can be considered as tolerable.
(Ammann et al., 2012, Mars Sample Return backward contamination - Strategic advice and requirements : 48)
Screenshots from (Ammann et al., 2012, Mars Sample Return backward contamination–Strategic advice and requirements : 21 and 48)
Their recommendation of 100% containment at 0.05 microns was the result of reviewing reports that ultramicrobacteria are still viable after passing through a 0.1 micron nanopore (Ammann et al., 2012, Mars Sample Return backward contamination–Strategic advice and requirements : 15). They cited two studies, in freshwater from Greenland (Miteva al., 2005 Detection and isolation of ultrasmall microorganisms from a 120,000-year-old Greenland glacier ice core) and 20 different sites in Switzerland (Wang et al., 2007, Quantification of the filterability of freshwater bacteria through 0.45, 0.22, and 0.1 μm pore size filters and shape-dependent enrichment of filterable bacterial communities).
This has been confirmed many times since then.
Text on graphic: Size limit 1999 to 2012: 0.2 microns
ESF Size limit (2012): 0.05 microns
The European Science Foundation study in 2012 reduced the limit from 0.2 microns to 0.05 microns after the discovery that these ultramicrobacteria are viable after passing through 0.1 micron nanopores
Next size limits review might reconsider ribocells – theoretical size limit 0.01 microns
ESF limit = ~⅛ of the wavelength of violet light
Background graphic: SEM of a bacterium that passed through a 100 nm filter (0.1 microns), larger white bar is 200 nm in length (Liu et al., 2019, Passage and community changes of filterable bacteria during microfiltration of a surface water supply)
violet bar for shortest wavelength of violet light (380 nm or 0.38 microns)
Mars might well have tiny microbes because
- can escape grazing by larger grazing amoebas which don’t notice them
- can use nutrients better in nutrient poor conditions, and so take up nutrients more efficiently because such tiny microbes have a far larger surface area to absorb the nutrients compared to their small volume.
[E.g. if a thousanth of the volume, they have ten times the area to volume ratio, so assuming the same permeability, can absorb ten times as much per unit volume]
See: (Ghuneim et al., 2018, Nano-sized and filterable bacteria and archaea: biodiversity and function)
These ultramicrobacteria are harmless to us, and a normal biosafety lab can't contain them. But ultramicrobacteria could introduce novel capabilities or a novel alien biology to Earth's biosphere and so has to be kept out.
Their 1 in a million containment at 0.01 microns or larger is because of a discovery that the even smaller Gene Transfer Agents (GTAs) transfer properties to unrelated microbes in the domain of archaea rapidly overnight in sea water, tested with GTAs for antibiotic resistance (McDaniel et al., 2010, High frequency of horizontal gene transfer in the oceans) (summarized in (Maxmen, 2010, Virus-like particles speed bacterial evolution)).
The European Science Foundation study summarizes the research like this:
Surprisingly, it is now estimated that GTA transduction rates are more than a million times higher than previously reported for viral transduction rates in marine environments (McDaniel et al., 2010)..
Clearly, GTAs are a major source of genetic diversity in marine bacteria.
(Ammann et al., 2012, Mars Sample Return backward contamination–Strategic advice and requirements : 19)
This is horizontal gene transfer - after receiving the antibiotic resistance capability via the GTAs, these microbes would confer the novel capability to their descendants.
In my preprint I focused attention on the requirement for 100% containment at 0.05 microns upwards.
A HEPA filter isn't designed to contain ultramicrobacteria with 100% effectiveness:
- ESF recommendation:
"The release of a single unsterilized particle larger than 0.05 μm is not acceptable under any circumstances"
(Ammann et al., 2012, Mars Sample Return backward contamination – Strategic advice and requirements : 48). - HEPA filters:
certified for a 4000-fold reduction in particles at 0.3 microns (99.975 containment).
(WHO, 2003. Laboratory Biosafety Manual Second Edition (Revised) : 35)
In addition, the technology doesn't yet exist\t to contain ultramicrobacteria. The smaller particles in a HEPA filter are smaller than the mesh size. They are contained as a result of Brownian motion, random jostling of particles by air molecules which will knock most of the smaller particles out of the air flow. This leads to a most penetrating particle size just below the minimum mesh size, and smaller particles are jostled more and nearly all of those are trapped depending on the air velocity. But a small number get through.
Very small particles like ultramicrobacteria are contained at nearly 100% level because the air molecules jostle them so much but they never quite achieve 100%. The ESF requirement is for all particle sizes GREATER THAN 0.05 microns. Even if the aim was only to contain ultramicrobacteria, they can clump, they also can be attached to larger dust grains or inhabit water droplets. In addition, mesh based filers such as HEPA filers are less effective for elongated small particles like ultramicrobacteria often are.
The 100% requirement would seem to need some new breakthrough technique rather than incremental changes such as more layers of filters or varying the spacing as those couldn’t get it all the way to 100% containment of such small particles.
It may be possible to achieve 100% containment of 0.05 micron particles in water under high pressure. A 2020 review of the literature found several studies that achieve a million fold reduction or more of small viruses in water ( Singh et al., 2020. Nanofiltration technology for removal of pathogens present in drinking water : 3) That doesn’t quite meet the target but Singh et al. found one study using carbon nanotubes loaded with silver that achieved 100% removal of very small viruses such as the polio, noro and Coxsackie viruses (Kim et al., 2016. A nanofilter composed of carbon nanotube-silver composites for virus removal and antibacterial activity improvement) (Singh et al., 2020. Nanofiltration technology … : 3).
This experimental filter could keep out 100% of polioviruses and the poliovirus is only 0.03 microns in diameter (Hogle, J. M., 2002. Poliovirus cell entry: common structural themes in viral cell entry pathways). However these filters for smaller nanoparticles for water treatment are easily damaged, through chemical and biological deterioration by aging, scratches by particle like substances, or fouling of the membrane (Singh et al., 2020. Nanofiltration technology … : 8)
The technology doesn't seem to exist yet to satisfy the ESF requirement. I have been unable to find any reference to anyone working on the technology of 100% filtration for air filters at any size.
Also there is an issue with testing filters over this very small size range, down to an eight of the wavelength of violet light. The filters are tested with challenge aerosols such as dioctylphthal (DOP) generated on the intake side of the filter, and measured with a photometer on the discharge side (Richmond et al., 2000. Primary containment for biohazards: selection, installation and use of biological safety cabinets), These photometers have limited sensitivity to nanoaerosols below the 100 nm limit. In a study of a DOP aerosol using TSI model 8130 Automated Filter Tester in 2008 (Eninger et al., 2008. What does respirator certification tell us about filtration of ultrafine particles? : table III), particles below 100 nm (0.1 microns) constituted 10% of the count of particles in the test aerosol, and 0.3% of the mass. However they provided almost none of the light scatter in the testing photometer (less than 0.01%). (Eninger et al., 2008, What does respirator certification tell us about filtration of ultrafine particles?)
Air incinerators are not certified to contain alien ultramicrobacteria yet - could they be a solution?
One astrobiologist has suggested adding an airflow incinerator in place of the second HEPA filter to try to bring a BSL-4 standard up to the requirements of the ESF study (private communication). Everyone agrees extraterrestrial life from Mars would be sterilized by a few minutes of heating to 500°C (Rummel et al., 2002, A draft test protocol for detecting possible biohazards in martian samples returned to Earth : 10)
This idea is similar but it’s to use an air-flow incinerator instead of an oven. Would an air incinerator be sufficient to contain the very small ultramicrobacteria, possibly ribocells, and extraterrestrial biology?
Sometimes an air incinerator is used in place of the second HEPA filter for a BSL-4 facility. A BSL-4 needs to use biosafety class III cabinets, and then the air from the cabinet must be passed through two HEPA or ULPA filters. In this arrangement, workers in the laboratory are protected by glove boxes. The cabinet provides a sealed workplace under negative pressure to keep any harmful microbes inside it. This negative pressure is maintained by removing air from it faster than it is drawn in. The HEPA filters are used to protect the biosphere and humans and animals outside the laboratory, with double HEPA filters on the exhaust air flow.
Graphic from: (WHO, n.d. Biological safety cabinets and other primary containment devices : 29)
Exhaust air must pass through two HEPA/ULPA filters in series, before discharge to the outdoors (NSF, 2019. NSF/ANSI 49 – 2019 Biosafety Cabinetry: Design, Construction, Performance, and Field Certification Informative Annex 1 : 29)
What interests us here is that NIH guidelines for research involving recombinant or synthetic nucleic acid molecules say that technicians can use air incinerators instead of a second air filter to contain biological hazards. These have to be tested with at least 100 million hardy spores per cubic foot of airflow and the test has to prove that all the infectious agents are sterilized. Though not quite the same as 100% containment this is very close to 100%.
If an air incinerator is used in lieu of the second high efficiency particulate air/HEPA filter, it shall be biologically challenged to prove all viable test agents are sterilized. The biological challenge must be minimally 1x10 8 [100 million] organisms per cubic foot of airflow through the incinerator.
It is universally accepted that if bacterial spores are used to challenge and verify that the equipment is capable of killing spores, then assurance is provided that all other known agents are inactivated by the parameters established to operate the equipment.
Test spores meeting this criterion are Bacillus subtilis var. niger or Bacillus stearothermophilus. The operating temperature of the incinerator shall be continuously monitored and recorded during use.
(NIH, Recombinant DNA Research, Volume 19 : 747)
However
- the test species are inevitably terrestrial life, and it is only a guideline for known agents.
- Normal ultramicrobacteria of terrestrial biology are not a risk to humans or livestock, and we haven’t develop any methods tested to contain these.
One might think that ultramicrobacteria are easier to contain than larger spores with an air incinerator. However, what if they are protected by being embedded in a larger particle?
- Can an air incinerator destroy ultramicrobacteria embedded in dust grains which would give extra protection?
Then any life from Mars would have millions of years of evolution in extreme conditions on Mars which terrestrial life has never encountered. It may have:
- evolved to resist perchlorates, hydrogen peroxide, UV, ionizing radiation, low pressure and low humidity,
- spores may have evolved additional protective layers that make it more resilient to incineration.
Since normal ultramicrobacteria of terrestrial biology are not a risk to humans or livestock we haven’t develop any methods tested to contain these.
- Can an air incinerator destroy ultramicrobacteria embedded in dust grains?
Then the air incinerator may have to contain life based on a different biology which may be more heat resistant than terrestrial life. As an example, PNA, has been proposed as the backbone in place of DNA and RNA for replicating biomolecules in a PNA-world “soup” before terrestrial life(Nelson et al., 2000. Peptide nucleic acids rather than RNA may have been the first genetic molecule). This is significantly more heat resistant than RNA (Jasinski et al., 2019. Thermal stability of peptide nucleic acid complexes). These figures are for lower temperatures than 500°C but they do show that PNA is more resistant to heat than RNA.
- In one experimental test of PNA heated for 150 to 200 ms (Jasinski et al., 2019. Thermal stability of PNA : Fig 10).
- All RNA - RNA base pairs are UNSTABLE beyond 350°C
- All PNA - PNA base pairs are STABLE up to 420 °C
- When heated for 200 ms, half the double strands melt, i.e. separate, at (Jasinski et al., 2019. ">(Thermal stability of PNA : Table 1)
- 314.6°C for RNA - RNA
- 347°C, for PNA - PNA
- In a test of melting for 200 ms, (Jasinski et al., 2019. Thermal stability of PNA : Fig 4).
- NEARLY ALL of the RNA - RNA strands separate at 340°C
- ALMOST NONE of the PNA - PNA strands separate at 340°C
Why more is needed than "NASA does not concur" for the issue of ultramicrobacteria containment
We've seen that it is a major challenge to contain particles at 0.05 microns and upwards. See above:
- Why NASA can't rely on HEPA filters for the ESF recommendation to contain 100% of ultramicrobacteria
NASA essentially don't see this requirement as a challenge, they think HEPA filters can already achieve it. My comment
Robert Walker: Draft PEIS OMITS the 2012 European Science Foundation study which reduced the size limit to 0.05 microns from the previous value of 0.2 microns - a serious omission since containment at 0.05 microns is well beyond the capability of BSL-4 facilities
…
ESF study: “the release of a particle larger than 0.05 μm in diameter is not acceptable in any circumstances”
The 2012 European Science Foundation study says its 0.05 micron size limit needs to be reviewed regularly – this alone is sufficient reason to halt this PEIS process until the new size limits review is done.(NASA, 2023. Mars Sample Return final PEIS : B-62 - B63)
[emphasis added in red, they may not have noticed the " larger than 0.05 μm" qualification or the requirement to review the size limit regularly]
[Note the ellipsis … repeated twice. in the screenshot - it combines some of the text from pages B-62 and B-63 on one page ](Walker, 2023. So many serious mistakes in NASA's Mars Samples Environmental Impact Statement it needs a clean restart) (docx) : 146, 147, 148, 49)
[Blue text shows the page numbers from my attachment for the text they quoted. The way the extract from my attachment is shown in the PEIS is a bit jumbled - it repeats the quotes from pages 147 and 148 a second time]
NASA's team responded to this statement saying
NASA: NASA is aware of the ESF Mars Sample Return backward contamination study. NASA does not concur that 0.05-micron (50 nm) particles cannot be managed; standard High Efficiency Particulate Air (HEPA) filters like those used in biosafety facilities are tested for effectiveness at or near the Most Penetrating Particle Size (MPPS), which is typically 0.12 micron … . (Perry et al, 2016, Submicron and nanoparticulate matter removal by HEPA- rated media filters and packed beds of granular materials). “Particles both larger and smaller than the MPPS (including bacterial spores and viruses) are removed with greater efficiency.”
(NASA, 2023. Mars Sample Return final PEIS : B-62)
I.e. they referred me to the HEPA standard, which is to filter out 99.97% at the most penetrating particle size (WHO, 2003, Laboratory Biosafety Manual Second Edition (Revised)).
From their reply here, NASA's team doesn't dispute the ESF's recommendation to contain 100% of particles of 0.05 microns and larger. It is just confident that HEPA filters already fulfil this requirement.
NASA's team is so confident in the correctness of its answer to my comment here, that it still doesn't mention the ESF recommendation in the main body of the final PEIS.
It is clear from this that
- NASA are not challenging the European Science Foundation's recommendation. They accept it but it is their opinion that HEPA filters can already achieve this level of containment.
- HEPA filters are certainly not CERTIFIED for 100% containment at any particle size so they plan to use them for a task they are not certified to work for and hope they would work.
- NASA didn't supply a source that said HEPA filters can archived 100% containment at all sizes above 0.05 microns
Beyond that point it is a little hard to follow their logic. I have however come across a similar confusion before. Whether or not this is how NASA's team were thinking, it is something that we should look at briefly to make it clear what the ESF recommendation does NOT mean.
- They could have been of the view that the ESF requirement was to achieve 100% for isolated single particles at 0.05 microns only
They
- may have missed the "larger than 0.05 microns" qualification and been of the opinion that the authors of the ESF study were only concerned about 100% containment of ultramicrobacteria and not of 100% containment of larger particles.
This is only conjectural. It's a mistake an engineer could make, looking at the graph and seeing that it achieves 100% or so close as to seemingly make no difference at the 0.05 microns level.
If so, it is an understandable mistake from engineers not familiar with biosafety methods and looking at a graphic in a paper. It would however show no understanding of the rationale behind the ESF recommendation to contain ultramicrobacteria that can pass through 0.1 micron nanopores.
First, the HEPA filters are not tested down to such small particle sizes and though theoretically they contain nearly 100% they can't be guaranteed to contain them all as a built in limitation in the design of the filters. Also, even if the requirement was only to contain ultramicrobacteria, they would be likely to be attached to dust particles or form clumps of microbes, and it is just as important to contain larger microbes as smaller microbes, including the maximum penetrating size of particle.
In more detail:
This is figure 3 from NASA's own cite to show the issue.
Text on graphic in dark blue (added by me). [Diffusion regime] Particles much smaller than gaps between fibers. Many hit the fibers by random jostling but always a few get through.
Too small to detect with photometry
(Perry et al, 2016, Submicron and nanoparticulate matter removal by HEPA- rated media filters and packed beds of granular materials : Figure 3)
HEPA filters are required to trap 99.97% of particles of 0.3 microns in diameter and 99.99% of particles of greater or smaller size (WHO, 2003, Laboratory Biosafety Manual Second Edition (Revised)). These standards don’t require 100% containment at any size.
This is a summary of the ESF recommendation
Text on graphic: ESF recommendation
Above 0.05 microns: 100% containment of Mars sample materialBelow 0.05 microns: 100% containment preferred. 99.9999% containment may be permitted on careful review.
HEPA filter most penetrating particle size specifies 99.975% containment
ULPA U17 goes up to 99.9999%
Graphic: (NIOSH, 2003, Guidance for Filtration and Air-Cleaning Systems to Protect Building Environments from Airborne Chemical, Biological, or Radiological Attacks : 11, Figure 4) using vectorization / text adaptation by Д.Ильин
A requirement of 100% containment for all particles larger than 0.05 microns includes a requirement for 100% containment at the most penetrating particle size, typically around 0.12 microns according to NASA's cite, the size where most particles get through the filter by definition.
No HEPA filter achieves 100% containment at the most penetrating particle size.
NASA are correct when they say that smaller particles (at least up to a point) are contained more easily than the ones at the most penetrating size.
From their figure 3, the smaller particles are trapped by diffusion, i.e. by brownian motion. They are far smaller than the gaps between the fibers and are small enough to be jostled around by air molecules. How many hit the fibers depends on the rate of air flow, particle size and fiber spacing. But this is a random process and can never achieve 100% containment.
Their own cite doesn't say 100% even for the very smallest airborne particles. It says nearly 100%.
The key implication to emphasize is that HEPA filters are nearly 100% efficient at capturing the spectrum of particles down to the very smallest airborne particles.(Perry et al, 2016, (Submicron and nanoparticulate matter removal by HEPA- rated media filters and packed beds of granular materials : 7)
The best way you can increase the efficiency of a HEPA filter is by reducing the air flow.
As a rule of thumb, reducing the velocity by half not only reduces the pressure drop by half but also decreases particle penetration through the filter media by nearly an order of magnitude.
(Perry et al, 2016, (Submicron and nanoparticulate matter removal by HEPA- rated media filters and packed beds of granular materials : 7)
A HEPA filter can't achieve 100% containment at any size.
Then there's the question of how well experiment matches theory. Though I found papers saying filters can achieve high levels of containment of small particles I didn't find actual filters designed, tested and shown to be able to achieve close to 100% containment even for such very small particles, ignoring the issue of larger particles for now.
In the US, HEPA filters are tested down to 0.1- 0.2 microns (depending on the class of filter, some are tested only at 0.3 microns). In Europe they are tested at the most penetrating particle size which may vary depending on the filter. In both cases, the filters are tested according to probabilities (Zhou et al, 2007, Comparison Of HEPA/ULPA Filter Test Standards Between America And Europe). (EMW, n.d., ISO 29463 - New test standard for HEPA Filters).
There is a higher standard than HEPA available. ULPA level 17 filters are rated to filter out 99.999995 percent of particles (BS, 2009, High efficiency air filters (EPA, HEPA and ULPA), Part 1: Classification, performance testing, marking : 2) in the range 0.12 microns to 0.25 micron (BS, 2009, High efficiency air filters : 4) according to BS EN 1822-1:2009, the British implementation of the European standard. But this is still not 100%.
But none of these standards require tests of containment at 0.05 microns. For instance what are the effects of the shape of the particles at those small sizes, and do Van der Waals forces become significant, and if so what effects do they have?
For details of the issues with testing containment at these very small sizes below the optical resolution of microscopes see the end of:
- Why NASA can't rely on HEPA filters for the ESF recommendation to contain 100% of ultramicrobacteria
Then, suppose theoretically you could achieve 100% containment at 0.05 microns but not at any larger size, what use would such a filter be to contain ultramicrobacteria?
Indeed it's a likely scenario in a sample of Mars, that ultramicrobacteria could be huddled away from the UV in tiny cracks in larger dust grains. They can also form clumps of cells that are larger.
Also, it's just as important to contain larger microbes than the smallest ultramicrobacteria. 100% containment at 0.05 microns and upwards means 100% at all sizes not just at 0.05 microns.
The main way smaller particles are contained is through brownian motion. Smaller particles are more easily disturbed by random jostling of air molecules. They are far smaller than the gaps between the fibers but as particles get smaller they are jostled around more easily and so a higher % of them hit the fibers of the filter. But there are many more small particles than large particles for the same mass. Also this is a random process and some will always get through if you are relying on the jostling of particles by air molecules to trap them.
So, no, it is not possible to achieve 100% containment at 0.05 microns, never mind at all sizes above 0.05 microns using standard HEPA filters.
Whatever the reason for the mistake, it is a clear example of issues that can arise when engineers use figures from a paper without a basic background in the concepts described in those papers.
NASA go on to tell me that the details of how NASA will use HEPA filters to comply with the ESF requirement to contain 100% of particles at 0.05 microns will be resolved at Tier 2.
Requirements associated with the SRF [Sample Receiving Facility] (to include management of particle size under the conditions required for sample curation) would be addressed in the Tier II phase of the NEPA process.
(NASA, 2023. Mars Sample Return final PEIS : B-62)
If the technology doesn't exist yet this can't be resolved at tier 2.
NASA didn't respond to my comment alerting them to the ESF recommendation to do an updated size limit review. They quoted what I said but didn't mention it in their reply.
Robert Walker: The 2012 European Science Foundation study says its 0.05 micron size limit needs to be reviewed regularly - this alone is sufficient reason to halt this PEIS process until the new size limits review is done.
(NASA, 2023. Mars Sample Return final PEIS : B-63)
This comment reply wasn't based on any new research they found relevant to the use of HEPA filters to contain ultramicrobacteria. They cited a paper that describes how HEPA filters work similarly to the ones I used in the attachment they responded to.
From this we see NASA has nobody working on this project familiar with how HEPA filters work or else they were unable to see the significance of the ESF recommendation. Either way it shows a lack of the expertise needed to design a biosafety laboratory as this would be basic 101 for anyone with the necessary expertise.
NASA's MSPG2 team which advised NASA on the design of the Sample Receiving Facility had nobody with expertise on human health, biosafety or biocontainment. The team that worked on the design of a Sample Receiving Facility said of the biocontainment and biosafety measures
"The specifics of these requirements are outside the expertise of MSPG2 members"
(Carrier et al., 2022. Science and Curation Considerations for the Design of a Mars Sample Return (MSR) Sample Receiving Facility (SRF) : S-232)
They left it for engineers and other experts at tier 2, after the process of public consultation.
See
We don't expect this to be an area of excellence for NASA, but access to experts with an understanding of HEPA filters is essential for any agency that wishes to design a biosafety laboratory adequate to contain samples from Mars. It is also essential when working on a biosafety plan from the earliest stages as the Space Studies Board has said many times. See above:
Why NASA should have commissioned a review of the size limit and level of assurance first, as recommended by the 2012 ESF study
Also before developing any such technology we need a review of the size limits and level of assurance - the ESF in 2012 said we need to do this regularly
Based on our current knowledge and techniques (especially genomics), one can assume that if the expected minimum size for viruses, GTAs or free-living microorganisms decreases in the future, and this is indeed possible, it will be at a slower pace than over the past 15 years.
However, no one can disregard the possibility that future discoveries of new agents, entities and mechanisms may shatter our current understanding on minimum size for biological entities. As a consequence, it is recommended that the size requirement as presented above is reviewed and reconsidered on a regular basis
(Ammann et al., 2012, Mars Sample Return backward contamination–Strategic advice and requirements : 21)
RECOMMENDATION 8: Considering that (i) scientific knowledge as well as risk perception can evolve at a rapid pace over the time, and (ii) from design to curation, an MSR [Mars Sample Return] mission will last more than a decade, the ESF-ESSC Study Group recommends that values on level of assurance and maximum size of released particle are re-evaluated on a regular basis
(Ammann et al., 2012, Mars Sample Return backward contamination–Strategic advice and requirements : 48)
A decade later this review is needed first before we can design any air incinerators or filters to the new standard. Amongst the topics it might look at are ribocells and prions. See Supplementary information under :
- A new size limit review could look at recent research into the very tiny ribocells
- A new size limit review could look at prions because of the recent discovery of prions in all three of the domains of life
NASA quoted the comment where I mention this recommendation but don't comment on it and seem to be of the view that HEPA filters could comply with any future requirements, or they didn't notice what I said.
Robert Walker: The 2012 European Science Foundation study says its 0.05 micron size limit needs to be reviewed regularly – this alone is sufficient reason to halt this EIS process until the new size limits review is done
NASA: [statement already given that they don't concur that this goes beyond the capabilities of a HEPA filter]
… Requirements associated with the SRF (to include management of particle size under the conditions required for sample curation) would be addressed in the Tier II phase of the NEPA process(NASA, 2023. Mars Sample Return final PEIS : B-62)
Recommendation of Mars sample return studies to involve public in risk governance as soon as possible, and NEPA requirement for interdisciplinary approach integrating social with natural sciences
One of the NEPA requirements is to use an interdisciplinary approach integrating the natural and the social sciences:
Agencies shall prepare environmental impact statements using an interdisciplinary approach that will ensure the integrated use of the natural and social sciences and the environmental design arts (section 102(2)(A) of NEPA).
§ 1507.2
To do this they also need preparers with the appropriate disciplines to do the integration and bring the insights of social sciences.
The disciplines of the preparers shall be appropriate to the scope and issues identified in the scoping process
§ 1502.6
Mars sample return studies emphasize the need to involve the public early on, not just in the USA, but through fora open to representatives from all countries globally because negative impacts could affect countries beyond the ones involved directly in the mission. This is a general recommendation for all space agencies and companies involved in sample return missions, and in terms of NEPA, this would relate to the rule for integrated use of social sciences
RECOMMENDATION 3
Potential risks from an MSR are characterised by their complexity, uncertainty and ambiguity, as defined by the International Risk Governance Committee’s risk governance framework. As a consequence, civil society, the key stakeholders, the scientific community and relevant agencies’ staff should be involved in the process of risk governance as soon as possible.
In this context, transparent communication covering the accountability, the benefits, the risks and the uncertainties related to an MSR is crucial throughout the whole process. Tools to effectively interact with individual groups should be developed (e.g. a risk map).
RECOMMENDATION 4
Potential negative consequences resulting from an unintended release could be borne by a larger set of countries than those involved in the programme. It is recommended that mechanisms and fora dedicated to ethical and social issues of the risks and benefits raised by an MSR are set up at the international level and are open to representatives of all countries
(Ammann et al., 2012, Mars Sample Return backward contamination–Strategic advice and requirements : 59)
This wasn't done. The public weren’t involved early on in that way. Nor is there any suggestion in the PEIS to set up such fora or methods of communication with the public.
I drew NASA's attention to this recommendation of the 2012 ESF study in a public comment.
The draft EIS shows clearly the results of not setting up any advanced planning and oversight agency with experts in legal, ethical and social issues tasked with interfacing NASA decisions and the general public’s questions as the top priority – as recommended in numerous papers on Mars sample return missions.
Rummel et al recommend a planning agency set up in advance with experts in legal, ethical and social issues - Uhran et al recommend an advanced planning and oversight agency set up two years before the start of the legal process – and the ESF recommends an international framework should be set up, open to representatives from all countries - NASA don’t seem to have done any of this yet
(NASA, 2023. Mars Sample Return final PEIS : B-67)
However from NASA's reply they saw NASA's participation in various committees such as COSPAR as fulfilling this requirement.
The Committee on Planetary Protection, International Council for Science, Committee on Contamination by Extraterrestrial Exploration (CETEX), and Committee on Space Research (COSPAR) all serve this purpose. It is not within the scope of the NEPA document to mandate implementation of planning and oversight agencies, only to analyze the potential environmental impacts associated with a proposed action
(NASA, 2023. Mars Sample Return final PEIS : B-67)
This doesn't show a clear appreciation of what is required or the reason for it, to enable their own communications with the public to work better.
In the section of my preprint which NASA replied to there, I gave an issue to show this absence of experience or expertise in communicating with the public about risk.
I gave this example of one of NASA's replies to an aggregated question from the public:
When the consequences of a failure are so great, a 100% guarantee should be required. ... Just how low is “low likelihood”? Is NASA’s goal specification to prevent accidental release of the Mars samples 1 in a thousand? 1 in a million? 1 in a billion?
(NASA, 2023. Mars Sample Return final PEIS : 4-8)
NASA replied in this way, which hasn't been changed in the final PEIS:
No outcome in science and engineering processes can be predicted with 100% certainty. The safety case for MSR safety is based on redundant containment supported by rigorous testing and analysis, the extensive experience of NASA and ESA with very similar activities over the past three decades, as well as independent reviews of program plans by external experts
(NASA, 2023. Mars Sample Return final PEIS : 4-8)
So they just say no outcome can be predicted with 100% certainty (not strictly true, for instance there is zero risk if the samples are not returned) and don't give any goal specification for target probability.
We will find out that the reason is they don't have such a goal specification. Also it's not true that NASA and ESA have conducted any similar activities, not since Apollo. The missions they refer to are sample returns from asteroids and comets where there is no risk of harm to Earth's biosphere or inhabitants and so the objective was just to protect the samples from contamination by terrestrial organics, there was no attempt to protect Earth from whatever might be in the samples because we have an infall of similar organics from space every year (the meteorite argument is valid for samples for Near Earth Objects).
We'll look at this reasoning in the Supplementary information under:
But let's return to the communications issue in this comment.
Perhaps an analogy will help to explain why it is not reassuring to the general public to answer in this way. Suppose a member of the public is a nervous flyer and asks the pilot
"what is the chance that this plane will crash? Is it 1 in a thousand? 1 in a million? 1 in a billion?"
The pilot answers:
"No outcome in science and engineering processes can be predicted with 100% certainty. But the safety case for this plane has been established by rigorous testing and analysis and we have been flying planes like this for three decades."
The pilot hasn't reassured the questioner that the risk is low or said anything about how low it might be. Indeed the pilot will seem evasive and this likely would add to the concern of the nervous flier.
In the section of my attachment which NASA responded to with this reply, I suggested it uses the example of a smoke detector as a way to communicate to the public that we are talking about low risk here, low risk but of large scale harm.
A good analogy, it's more of the order of building a house without a smoke detector - but a house you share with nearly 8 billion people - than setting off outdoor fireworks in the kitchen. This smoke detector analogy is from Margaret Race from her contribution "No Threat? No Way" in the Planetary Report " (Rummel et al., 2000, Opinion: No Threat? No Way : 5). In this cite, she is responding to Robert Zubrin, president of the Mars society who thinks we don’t need to protect Earth from a Mars sample return. She wrote in 2000:
" ... If he were an architect, would he suggest designing buildings without smoke detectors or fire extinguishers?
(Walker, 2023. So many serious mistakes in NASA's Mars Samples Environmental Impact Statement it needs a clean restart) (docx) : 122)
I use this analogy near the start of this book to help communicate the low level of risk clearly to the public from the outset:
However NASA's team didn't understand the point I was making there. This is the only relevant part of it that they quote in the final PEIS:
The draft EIS shows clearly the results of not setting up any advanced planning and oversight agency with experts in legal, ethical and social issues tasked with interfacing NASA decisions and the general public’s questions as the top priority – as recommended in numerous papers on Mars sample return missions. Rummel et al recommend a planning agency set up in advance with experts in legal, ethical and social issues - Uhran et al recommend an advanced planning and oversight agency set up two years before the start of the legal process – and the ESF recommends an international framework should be set up, open to representatives from all countries - NASA don’t seem to have done any of this yet.
(NASA, 2023. Mars Sample Return final PEIS :B-67)
NASA is replying there to the long title of one of the sections of my attachment here: (Walker, 2023. So many serious mistakes in NASA's Mars Samples Environmental Impact Statement it needs a clean restart) (docx) : 120 - 122) [copy of the paper I uploaded on the last day of public comments]
NASA replied
The Committee on Planetary Protection, International Council for Science, Committee on Contamination by Extraterrestrial Exploration (CETEX), and Committee on Space Research (COSPAR) all serve this purpose. It is not within the scope of the NEPA document to mandate implementation of planning and oversight agencies, only to analyze the potential environmental impacts associated with a proposed action
(NASA, 2023. Mars Sample Return final PEIS :B-67)
Of course none of those Committees serve this purpose of interfacing NASA decisions and the general public’s questions that I mentioned. And yes it is not within the scope of the NEPA document because this should have been setup earlier as a decision about how NASA would handle dialogue with the public, it wouldn't be enough to mention it in the document as a requirement.
We see these issues in the response of the NASA scientists and engineers to the questions from the public concerning risk. They respond in stark scientific language and they just say "the risk cannot be demonstrated to be zero".
No matter what the concern is, even if a member of the public is concerned about possible eradication of all terrestrial life or that we would need to live in biomes for the rest of time as a result of the sample returns, NASA makes the same standard answer. See above: NASA's standard response to public comments raising concerns of unprecedented levels of global harm from this mission, even concerns about mass extinction: "the risk cannot be demonstrated to be zero" jars with their conclusion in the main body of the statement that any environmental risk would not be significant and any public health impact negligible.
Clark in his paper says even if there were no effects or mild effects from the sample return, the public might make connections that are not real between the returned samples and other events that are accidentally correlated with it.
Much worse, the effect on future programmatic support could result in a far greater negative impact.
The public has already been conditioned to the dangers and potential horrors of "biological warfare" by terrorist groups or rogue nations.
Clear examples of negative public sentiment gone overboard has surrounded asbestos removal programs (even for low-risk varieties of the mineral), banning of generation of electrical power by nuclear methods, and extremely low acceptable limits on many chemical pollutants.
Even if martian organisms were not harmful in any way but could not be unequivocally proven to be non-infectious, there could be strong concern. Many people might react very negatively if told by scientists (perceived as self-serving) that there was no need to worry about possible future effects of inadvertently released alien matter. The public is very aware, for example, of the killer bee fiasco.
Another effect that is highly important in the context of a globally conscious society is the ecological issue. Large expenditures in resources are routinely accrued or promised for the future preservation of the current ecological state, or at least the reduction of the impact on same by the activities of humans. Any spread of alien organisms could be viewed as serious in this scenario, no matter how mild and difficult to measure adverse consequences
(Clark, 2002, Martian meteorites do not eliminate the need for back contamination precautions on sample return missions : 1597)
Clark also raised the issue that the general public could make connections that are not scientifically valid.
Two examples he gives are (Clark, 2002, Martian meteorites do not eliminate the need for back contamination precautions on sample return missions : 1598)
- nukes and climate: in the 1950s it was popularly thought that the nukes caused changes in the climate .
- In the other direction many think that CO2 doesn't cause global warming .
For a recent example of the public making connections that are scientifically impossible:
- in a poll by the economist 1 in 20 of US citizens 1 in 20, are sure that the US government is using COVID vaccines to track its citizens via a microchip.
- 1 in 5 think it is either definitely or probably true that the US government tracks its citizens in this way, using COVID vaccines
(YouGov, 2021, The Economist/YouGov Poll : 132).
In these passages Clark is talking about potential public reactions with conspiracy theories to effects that happen after the samples are returned. But similar reasoning could arise about NASA's intentions before the samples are returned, and could lead to cancellation of the mission even if it is harmless.
This book proposes that we return the sample to a biosafe orbit above GEO. That might seem to eliminate such issues of concern for the general public, scientifically it should but in practice, it may not if the public dialogue isn't handled carefully.
Even if we sterilize samples returned from the satellite, this has to be communicated clearly and effectively and concerns of the public listened to. Otherwise there is a strong that the public will make similar connections that are not scientifically valid to return of even sterilized material that is thoroughly sterilized beyond any possibility of contamination of Earth's biosphere with living organisms.
NASA do say accurately it is not the mandate of the PEIS team to set up such committees. NASA should have set up the necessary processes as soon as possible by recommendation 3 of the ESF study (Ammann et al., 2012, Mars Sample Return backward contamination–Strategic advice and requirements : 59)
So, this is an issue with the disciplines of those involved in preparing the PEIS itself and presenting it to the public, and omitted preparations NASA should have started long before the PEIS. It is not an issue with the disciplines of any members of COSPAR and other committees that NASA is a member of.
This reply does however state correctly that it is not within the scope of the PEIS document to mandate such expertise in communications or the other recommendations of the planetary protection studies. NEPA requires this expertise to be in place already, before the preparation of the PEIS document starts:
Agencies shall prepare environmental impact statements using an interdisciplinary approach that will ensure the integrated use of the natural and social sciences and the environmental design arts (section 102(2)(A) of NEPA). The disciplines of the preparers shall be appropriate to the scope and issues identified in the scoping process (§ 1501.9 of this chapter).
One would expect the scoping process to turn up the recommendations in the planetary protection literature for this expertise and these tools for interfacing with the public.
These issues with the disciplines of the preparers would seem by themselves to be sufficient to require a restart of the PEIS.
The many mechanisms to communicate effectively with the public and listen to public concerns that previous sample return studies mandated would be included in that phrase: integrated use of the natural and social sciences and the environmental design arts.
This is how John Rummel, NASA’s first planetary protection officer, put it in 2002:
John Rummel: As part of sample return planning, it will be important to develop an organized communication plan which will lay a strong foundation in public understanding and acceptance prior to the mission, and allow for an open dialogue with all sectors of the public. Such a plan should include consideration of the diverse questions, concerns, and issues likely to be raised, including those related to the mission and spacecraft operations, the sample return and Biohazard testing, the administrative and legal matters associated with the effort, and to the potential implications of discovering extraterrestrial life.
… Such a discovery would likely trigger a review of sample return missions, and plans for both robotic and human missions. Legal questions could arise about ownership of the data, or of the entity itself, potentially compounded by differences in laws between the United States and the countries of international partners. In any event, ethical, legal and social issues should be considered seriously. Expertise in these areas should be reflected in the membership on appropriate oversight committee(s).
…“Broad acceptance at both lay public and scientific levels is essential to the overall success of this research effort.”
(Rummel et al., 2002, A draft test protocol for detecting possible biohazards in martian samples returned to Earth : 99)
In short, the NEPA requirements are there for a reason, and it is especially important to comply with them in a project like this which is
- unlike anything we have ever done before and which
- has so many unknowns at our current stage of understanding of Mars and of exobiology.
As we saw, the sample return studies go further than NEPA. As described in the Mars sample return studies, it is important to prepare to involve the public in this way as soon as possible. This wasn't done,
Margaret Race said scientists are likely to focus on (Race, 1996, Planetary Protection, Legal Ambiguity, and the Decision Making Process for Mars Sample Return)
- technical details
- mission requirements
- engineering details
- costs of the space operations and hardware
General public are likely to focus on
- risks and accidents
- whether NASA and other institutions can be trusted to do the mission
- worst case scenarios
- whether the methods of handing the sample, quarantine and containment of any Martian life are adequate
The basic engineering details for NASA's mission plan are worked out. But NASA haven't made a start on most of the matters of most concern to many members of the general public.
For this particular mission those issues that most concern the public require as much attention as the engineering issues for success. Without sorting out those issues NASA can't expect to be able to do a valid PEIS or to succeed in this mission.
Rummel in his 2002 study just quoted summarized a point that is stressed in all the major Mars sample return studies.
“Broad acceptance at both lay public and scientific levels is essential to the overall success of this research effort.”
(Rummel et al., 2002, A draft test protocol for detecting possible biohazards in martian samples returned to Earth : 99)
That broad acceptance is as essential for the success of this mission as getting the calculations right about the amount of rocket fuel you need to launch the samples into orbit from the Mars surface.
See also the Supplementary information under :
This is not just something that NASA needed according to planetary protection experts and to comply with the NEPA requirements on a PEIS. It needs it for itself too as all these experts have said these things for a reason.
With its current plans,NASA is certain to get many questions from the public concerned about a risk of unprecedented harm to human health or the environment as the mission progresses and gets closer to an actual sample return. The general public may not find their answers acceptable.
In short, NASA need to prepare already now for the concerns of the public as we approach the sample return in the 2030s. NASA should have prepared for this a decade ago. See above: NASA's standard response to public comments raising concerns of unprecedented levels of global harm from this mission, even concerns about mass extinction: "the risk cannot be demonstrated to be zero" jars with their conclusion in the main body of the statement that any environmental risk would not be significant and any public health impact negligible.
Even the most well prepared labs can have lab leaks - Sagan's insoluble "vexing question" of the quarantine period for a novel alien pathogen
We also return to a matter that hasn't seen attention since the Apollo era. Carl Sagan put it like this: (Sagan, 1973, The Cosmic Connection - an Extraterrestrial Perspective : 130)
There is also the vexing question of the latency period. If we expose terrestrial organisms to martian pathogens, how long must we wait before we can be convinced that the pathogen-host relationship is understood? For example, the latency period for leprosy is more than a decade. Because of the danger of back-contamination of Earth, I firmly believe that manned landings on Mars should be postponed until the beginning of the next century, after a vigorous program of unmanned Martian exobiology and terrestrial epidemiology.
(Sagan, 1973, The Cosmic Connection - an Extraterrestrial Perspective : 130)
Any biosafety laboratory with human technicians has to have emergency procedures in place for lab leaks
Emergency/incident response: An outline of the behaviours, processes and procedures to be followed when handling sudden or unexpected situations, including exposure to or release of biological agents. The goal of an emergency/incident response is to prevent injuries or infections, reduce damage to equipment or the environment, and accelerate resumption of normal operations
(WHO, 2020, World Health Organization Laboratory biosafety manual, 4th edition : 14)Even the most well prepared laboratory may experience unintentional or intentional incidents or emergencies despite existing prevention or risk control measures.
(WHO, 2020, World Health Organization Laboratory biosafety manual, 4th edition : 87)
There is no specific requirement for quarantine. Instead for biological spills, the manual just says:
Personnel must immediately vacate the affected area.
Exposed persons should be referred immediately for medical evaluation.
(WHO, 2020, World Health Organization Laboratory biosafety manual, 4th edition : 66)
However this advice is for a biological spill of a known pathogen or type of organism. There may be no method of medical evaluation that could detect if a technician harbours an alien pathogen. We will cover this in the next section:
It also says
An incident response protocol should be written and followed to ensure proper reporting, and to facilitate investigation, root-cause analysis, corrective action and process improvement.
(WHO, 2020, World Health Organization Laboratory biosafety manual, 4th edition : 87)
However after some future release of extraterrestrial life from Mars it would be too late to contain it by root-cause analysis, corrective action etc.
In the Apollo era faced with the possibility of a pathogen of unknown capabilities and origins the only emergency response they had available to contain the pathogen was quarantine. This seems to be likely to be the same situation today. But that then leads to the issue of the latency period, Carl Sagan's vexing question.
We now know that leprosy can take 20 years or more to show symptoms (WHO, 2019, Leprosy, Key facts).
In the Apollo era, NASA knew they couldn't keep out an alien or novel pathogen of unknown properties with quarantine.
Richard Bryan Erb was the manager of the Lunar Receiving laboratory from 1969 to 1970 (Carroll, 2019, The Early History of Canadian Planetary Exploration). He explained that the Apollo quarantine procedures were just intended to try to stop a pathogen with a short incubation period of less than 3 weeks
Erb: You never know whether something might show up in thirty years. There are viruses and things that will show up long after the fact, but the theory was that if you can go through a quarantine for three weeks, which was the time set, without adverse effect, then you're obviously not dealing with something that is rapidly reacting and dangerous, so you would have time to prepare a remedial action. It was a good trade, I think, between a hazard, which was not very likely, but a risk of perhaps life on Earth, which was immense.
(Butler, 1999, Edited Oral History Transcript).
No quarantine period long enough to quarantine a "Typhoid Mary": a life-long symptomless carrier of extraterrestrial biology such as martian soil fungi or mirror life
[FIXCITES]
Carl Sagan could also have used the example of Typhoid Mary, a lifelong symptomless carrier of typhoid, (Korr, 2010, Mary Mallon: First Asymptomatic Carrier of Typhoid Fever) (Marinelli et al., 2013, Mary Mallon (1869-1938) and the history of typhoid fever)
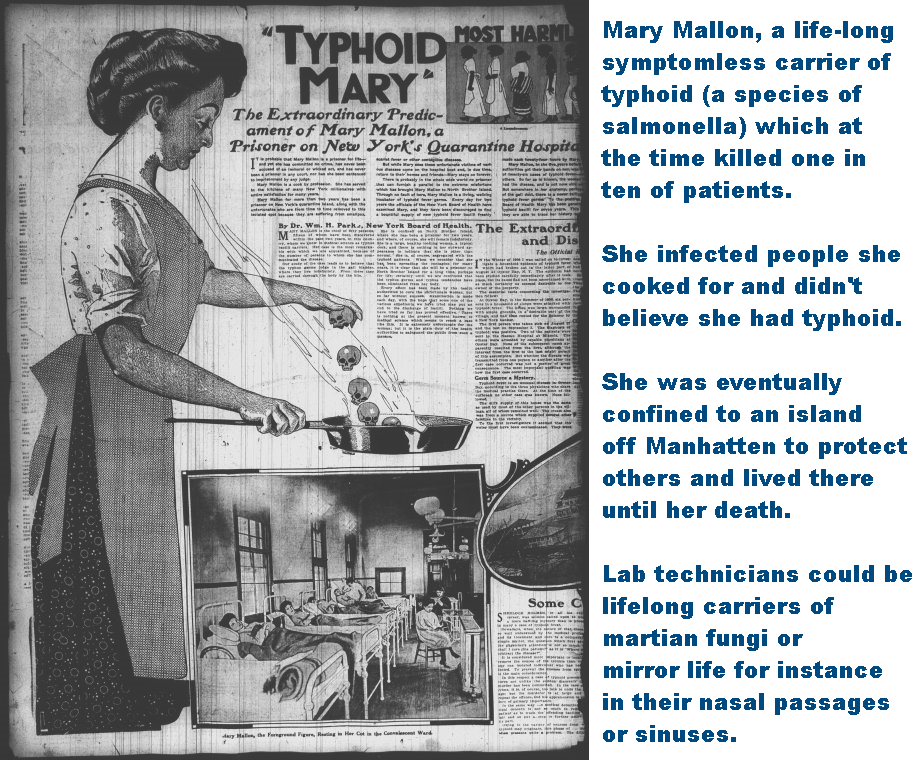
Text on graphic: Mary Mallon, a life-long symptomless carrier of typhoid (a species of salmonella) which at the time killed one in ten of patients.
She infected people she cooked for and didn't believe she had typhoid.
She was eventually confined to an island off Manhattan to protect others and lived there until her death.
Lab technicians could be lifelong carriers of martian fungi or mirror life for instance in their nasal passages or sinuses.
Graphic from (General Research Division, The New York Public Library, 1909. "Typhoid Mary" )
Most people have small amounts of viable fungi in their nasal passages and sinuses. A few will have a stable colony, or one that is slowly growing. This can grow as colonies in nasal crusts, or can form a harmless fungal ball in the sinuses, which the patient is unaware of, or it may grow to the extent that it causes problems, or they may have allergic reactions to it, or in some cases the fungi may spread from the sinuses in an invasive fashion and be life threatening, often as a result of an other disease weakening the immune system or because the patient needs to be treated with immunosuppressants (Soler et al., 2012, The role of fungi in diseases of the nose and sinuses).
So, if a martian fungus is able to colonise the nasal passages of a human being, it might be possible for it to persist for years at low levels in some individuals similarly to Typhoid Mary. However, unlike the fungi that are already common in the environment, this would be a fungus from Mars that has never reached our biosphere before and which we must prevent from spreading through the biosphere after a lab leak of a lab containing samples from Mars.
Fungi can also colonize the skin and toenail infections are very common. Up to 14% of the population may be affected by fungal toenail infections according to the CDC (CDC, n.d., Fungal Nail Infections), One estimate they cite is that 6.5% of the Canadian population have fungal toenail infections (Gupta et al., 2000, Prevalence and epidemiology of onychomycosis in patients visiting physicians' offices: a multicenter canadian survey of 15,000 patients). These infections often include species that are not adapted to infect the skin such as Aspergillus (Shenoy et al. 2014. Fungal nail disease (Onychomycosis); Challenges and solutions)
For a vivid illustration, the ISS has a preflight “Health stabilization program” which uses vaccination and a 14 day quarantine to help prevent upper respiratory infection and gastroenteritis (Health Stabilization Program V1 4.4.2.4) but of course this can’t keep out fungi that are harmless to young healthy astronauts. Two of the Zinnia plants died from a fungal pathogen probably brought there by one of the astronauts.
Text on graphic: Mold growing on a Zinnia plant in the ISS. The mold fusarium oxysporum likely got to the ISS in the microbiome of an astronaut (Draft genome sequences of two Fusarium oxysporum isolates cultured from infected Zinnia hybrida plants grown on the international space station). (How Mold on Space Station Flowers is Helping Get Us to Mars)
This fungal disease fusarium oxysporum is also an occasional opportunistic pathogen of humans (Genomic Characterization and Virulence Potential of Two Fusarium oxysporum Isolates Cultured from the International Space Station)
This is just intended as an illustration to show that it's impossible to use quarantine to keep out a fungal disease. In this case, the fungus didn't kill the plants by itself. First, the plants got too much water, then four got mold. After a change to a higher fan speed two of the four infected plants recovered but two of them died (How Mold on Space Station Flowers is Helping Get Us to Mars) The strains isolated from the ISS weren’t able to infect healthy Zinnia plants (Genomic Characterization and Virulence Potential of Two Fusarium oxysporum Isolates Cultured from the International Space Station : 13). Other strains of fusarium oxysporum were found in isolates from the dining table in the ISS. (Genomic Characterization and Virulence Potential of Two Fusarium oxysporum Isolates Cultured from the International Space Station). Also we can’t know how this fungus got to the ISS, but it could be brought there on an astronaut’s microbiome.
However it's a vivid example to show how we can't keep fungi out of the ISS and it would be the same for any Martian fungi that can live in or on humans, most likely we wouldn't be able to keep them inside a quarantine facility. There are many other fungi on the ISS including Aspergillus fumigatus (Characterization of Aspergillus fumigatus Isolates from Air and Surfaces of the International Space Station) which is not likely to cause problems for healthy young astronauts and is commonly found in buildings.
There would be no way to do medical evaluation that could detect with certainty whether or not a technician harbours a small colony of mirror life, an alien fungus or some other novel organism of unfamiliar biology that may have got into a technician's microbiome, including for instance into their lungs, nasal passages, etc. This might include
- pathogens harmless to the technician but harmful to some other humans as for typhoid Mary,
- pathogens that are slow acting such as fungal balls that may grow slowly,
- only harmful when the immune system is weakened by another disease or immunosuppressants,
- harmless to humans and able to harm livestock, wildlife, plants, insects, marine phytoplankton, etc,
- that could spread through ecosystems displacing familiar terrestrial biology, as for microbes based on mirror organics.
We can't assume that the pathogen or alien life has been identified in the samples yet at the time of a lab leak. If it has been identified, we still may not have tests that can detect it in a human, see below:
If we can detect it, it might be possible to remove most of it with surgery. But even if we can identify it and know it is there, it is not likely to be possible to eliminate an alien fungus or other soil pathogen or mirror life completely from the nasal passages and sinuses of a human technician to the extent that one can be sure there is no possibility of it spreading it to Earth's biosphere ever.
A novel fungal genus from Mars or perhaps a novel fungus based on alien biology, might also be naturally resistant to our antifungals as they target specific cell processes that may not exist in the novel genus or biology. It typically takes 12 years to develop and test a new antifungal treatment and though this would surely be accelerated in an emergency situation as for the COVID vaccines it may still take significant time. We look at this in the Supplementary information under:
I can’t find any previous discussions of asymptomatic carriers in the planetary protection literature. However, infectious disease specialists such as the WHO experts are certain to raise this issue once they become involved in the legal review process. Typhoid Mary (Korr, 2020) is a famous example of an asymptomatic carrier of a disease. She was healthy, symptomless, but transmitted typhoid to the people she cooked meals for. She was a symptomless carrier, or she could be called a life-long “symptomless super-spreader”. She had to be isolated to protect others.
Public health challenges responding to the accidental release of an extraterrestrial pathogen of unfamiliar biology
Pugel et al look at the public health response to an escape of extraterrestrial life. This is focused on responses to exposure at the re-entry crash site as for the PEIS but it also gives an idea of some of the issues that need to be discussed when it comes to preparing for lab leaks from a Mars Receiving Facility.
They raise the issue that an extraterrestrial organism and might fall outside any known classification, likely leading to knowledge gaps on incubation periods, how we can be exposed to it, and how to disinfect it.
Normal infectious disease response mechanisms rely on knowledge surrounding incubation, exposure, and disinfection. A novel pathogen from extraterrestrial material is likely to be wholly unique and possibly fall outside of conventional microorganism taxonomy
Hospital preparedness scenarios depend on laboratory testing and medical management. However, existing methods for rapid development of diagnostic tests for terrestrial pathogens might not be suitable for an extraterrestrial pathogen based on an unfamiliar biology.
(Pugel et al., 2020. Restricted and Uncontained: Health Considerations in the Event of Loss of Containment During the Restricted Earth Return of Extraterrestrial Samples : 9).
There would be likely to be knowledge gaps involved in developing diagnostic tests.
An extraterrestrial pathogen lacks existing diagnostic testing and medical management protocols
(Pugel et al., 2020. Restricted and Uncontained: Health Considerations in the Event of Loss of Containment During the Restricted Earth Return of Extraterrestrial Samples : 9).
They mention that hospitals responding to cases of people sick with an alien pathogen wouldn't be able to use standard procedures but would need advice from the Federal agencies including advice on matters such as disinfection methods, and length of isolation but this advice may not be available in a timely fashion for a novel pathogen of alien biology.
Normal infectious disease response mechanisms rely on knowledge surrounding incubation, exposure, and disinfection. A novel pathogen from extraterrestrial material is likely to be wholly unique and possibly fall outside of conventional microorganism taxonomy.
Hospitals have existing surveillance protocols within their infection prevention and control programs but will depend upon guidance given from federal agencies.xxvi This would include guidance on exposure definitions and infection control, which would include PPE, negative pressure requirements, disinfection/isolation practices length of isolation).
However there may be knowledge gaps that can't be filled quickly about how to respond to a pathogen based on alien biology.
The strength of this guidance in the context of surveillance was based upon established pathogen guidelines, which may not exist in a timely fashion for a potential extraterrestrial pathogen. Such information gaps could pose a challenge for willingness to work, as well as compliance to temporary isolation guidelines.
(Pugel et al., 2020. Restricted and Uncontained: Health Considerations in the Event of Loss of Containment During the Restricted Earth Return of Extraterrestrial Samples : 9).
A hardy microbe based on alien biology might also challenge the methods hospitals use to sterilize surfaces and equipment.
A novel space pathogen also challenges the ability to properly disinfect and sterilize equipment and surfaces in the healthcare environment. Current practices following the Spaulding classification method categorizing instruments and other patient care equipment as critical, semicritical, and noncritical based upon the degree of infection risk involve when the item is used.xxxi Will the Spaulding approach to disinfection and sterilization be applicable to critical medical equipment used to care for infected patients in this unique circumstance?
(Pugel et al., 2020. Restricted and Uncontained: Health Considerations in the Event of Loss of Containment During the Restricted Earth Return of Extraterrestrial Samples 10).
They suggest that our most hardy organisms such as b. subtilis could be a starting point for developing this guidance.
There are currently known organisms that are environmentally hardy, like Bacillus anthracis and Clostridium difficile spores, which already challenge existing infection control efforts but could be a starting point for which disinfection and sterilization recommendations to be made for a space pathogen
(Pugel et al., 2020. Restricted and Uncontained: Health Considerations in the Event of Loss of Containment During the Restricted Earth Return of Extraterrestrial Samples 11).
This issue also applies to disposal of waste and contamination of objects and surfaces generally.
This equally applies to disposal of waste when disinfection or sterilization cannot be performed on items associated with extraterrestrial pathogen exposure.;
Beyond sterilizing medical equipment, the environmental contamination of objects and surfaces requires disinfecting guidelines. The ability for existing disinfection practices and products to be effective is unknown and a considerable knowledge deficit.
(Pugel et al., 2020. Restricted and Uncontained: Health Considerations in the Event of Loss of Containment During the Restricted Earth Return of Extraterrestrial Samples : 9).
Pagel et al. mention the idea of testing the samples with terrestrial organisms in parallel with the quarantine period.
Apollo 11, 12, and 14 astronauts spent 21 days in quarantine. In parallel, lunar samples, underwent 30-day test periods under containment to look for evidence of replicating microbial life via a range of methods, including tests for viral replication in vertebrate and invertebrate systems.
(Pugel et al., 2020. Restricted and Uncontained: Health Considerations in the Event of Loss of Containment During the Restricted Earth Return of Extraterrestrial Samples : 9-10).
However, the COSPAR Sample Safety Assessment Framework found that there is no way to use this approach to predict the effects of a novel extra-terrestrial organism on ecosystems.
During the Working Group’s deliberations, it became clear that a comprehensive assessment to predict the effects of introducing life in new environments or ecologies is difficult and practically impossible, even for terrestrial life and certainly more so for unknown extraterrestrial life.
…
Unfortunately, we have only a limited ability to predict the effects of terrestrial invasive species, emerging pathogens, and uncultivated microbes on Earths' ecosystems and environments. This is true even for cultured and fully genome-sequenced terrestrial organisms and more so for potential extraterrestrial life. Thus, conducting a comprehensive sample safety assessment with the required rigor to predict harmful or harmless consequences of potential martian life for Earth is currently not feasible.
(Kminek et al, 2022, COSPAR Sample Safety Assessment Framework (SSAF))
Pugel et al discuss issues with pathogens with long incubation periods. The Apollo astronauts had a year of surveillance. But that may not be practical if a large number are exposed. They also had no movement restrictions. It is important to note that the Apollo astronauts had no restriction on travel after release from quarantine.
Advances in research since Apollo have uncovered a wealth of dormant species and slow metabolizers, which reveals a wide range for potential incubation periods and challenges older quarantine protocols.
Medical surveillance of Apollo astronauts continued for one calendar year after leaving quarantine, but response efforts to a larger exposure with novel organisms pose unique challenges for prolonged surveillance/quarantine that may be impractical to implement.
(Pugel et al., 2020. Restricted and Uncontained: Health Considerations in the Event of Loss of Containment During the Restricted Earth Return of Extraterrestrial Samples : 10.
See below:
This also wouldn't detect pathogens with a latency period longer than the quarantine period and tests on human analogue organisms can't fully predict effects on humans either.
Pugel et al. expect Federal agencies to update their requirements from time to time during the outbreak as their understanding develops, if a pathogen escapes containment.
Like the hospital response during SARS-CoV outbreaks and the Texas Ebola virus disease cluster in 2014, protocols and recommendations will evolve with the strength of the correlation between exposure and disease.xxxv The relationships among all parties, from federal agencies to local hospitals, will be critical as guidelines and processes are developed in real-time.
(Pugel et al., 2020. Restricted and Uncontained: Health Considerations in the Event of Loss of Containment During the Restricted Earth Return of Extraterrestrial Samples : 11).
Judging from the discussions during the Apollo era this issue of isolation periods for a lab leak is likely to get considerable discussion. But in some cases such as mirror life, or alien fungi as I mentioned early on, there may be no resolution of the issue in a lab run by human technicians.
Emergency plans for a fire or a life threatening condition: Apollo technicians or astronauts would immediately be released from quarantine and if very sick moved to intensive care in a hospital
If a technician or astronaut became seriously ill and needed urgent treatment that wasn’t available within the quarantine facility, NASA’s stated plan for the Apollo program was to immediately take them out of quarantine and to a hospital. Its plans also say that it would respond in the same way if an astronaut or technician had a major injury, or if the quarantine facility went on fire: immediately take them out of quarantine.
The MSC’s Integrated Quarantine Operations Plan stipulated that if an astronaut suffered a medical emergency that could not be handled at the LRL, “the quarantine may be broken and the individual transported to the nearest appropriate medical facility.” This meant that if a pathogen was dangerous enough to make an astronaut truly sick, NASA would remove that astronaut from quarantine and allow the pathogen to escape.
,,,
An LRL training handbook, for example, stipulated that in the event of a major injury, emergency personnel could enter and exit the Sample Operations Laboratory without following decontamination procedures. The same handbook outlined procedures whereby the entire LRL—including the sections behind biological barriers—would be evacuated in the event of a fire.
(Degroot, 2023. One Small Step for Man, One Giant Leap for Moon Microbes? Interpretations of Risk and the Limits of Quarantine in NASA’s Apollo Program).
That then risks hospital-acquired infections (nosocomial infections) which are often the main way an infectious disease spreads, for instance this is one of the main ways MERS spreads. (Hui et al., 2018, Middle East respiratory syndrome coronavirus: risk factors and determinants of primary, household, and nosocomial transmission)
Lab leaks are often due to human error or equipment failure with numerous breaches of containment for the Lunar Receiving Laboratory
Any precautions for lab leaks also have to take account of human error. There are many examples, such as a SARS outbreak in 2003 in Taiwan which happened because a technician skipped the standard procedure after a spill, because it would make him late for a conference (Demaneuf, 2020. The Good, the Bad and the Ugly: a review of SARS Lab Escapes)
Other escapes could happen from equipment failure. Human error and equipment failure were the two most common reasons for quarantine in the Apollo program.
NASA had 6 breaches of containment for Apollo 11 in the sample receiving lab between 23rd July and 8th August 1969 (excluding the breaches of containment of the quarantine facility).
- July 25, Technician directly exposed to lunar dust
- July 25, Sample bag leaked, spacecraft storage room overflowed
- July 30: Peracetic acid (sterilizing agent) found in lunar sample
- July 31: Two spacesuit gloves ruptured in vacuum chamber
- August 2: Technician punctured biocabinet glove with instrument
- August 5: Technicians sprayed with contaminated liquid from autoclave
See: (Degroot, 2023. One Small Step for Man, One Giant Leap for Moon Microbes? Interpretations of Risk and the Limits of Quarantine in NASA’s Apollo Program : figure 5).
NASA solved many of those problems for Apollo 12. Yet the leaks continued:
Engineers had implemented dozens of changes since Apollo 11 to help prevent, identify, and control containment faults and spills in the LRL. Yet human error was harder to avoid. “Inappropriate procedures” soon led a technician to tear open a glove in a biocabinet, exposing eleven technicians who were then quarantined in the Crew Reception Area. By coincidence, one fell ill and isolated in the MQF for seventy-two hours. On 11 December, another “improper procedure” in an autoclave exposed four technicians to “potentially contaminated fluid.” Although they were “followed closely” for three weeks, they were not quarantined
(Degroot, 2023. One Small Step for Man, One Giant Leap for Moon Microbes? Interpretations of Risk and the Limits of Quarantine in NASA’s Apollo Program).
As Richard Erb put it in his oral history transcript:
Erb: It'll be interesting to go through this again as we tackle Mars samples return, because in three or four years we'll be coming back with samples from Mars, and we'll have to think through all the same decisions, but now with, I think, a much greater likelihood of life forms from Mars. So the quarantine issue has already been discussed again.
(Butler, 1999, Edited Oral History Transcript).
I have not found these discussions of the quarantine period, except for historical discussions like this one. The modern planetary protection literature generally assumes we can contain martian organisms using similar protocols to terrestrial diseases without looking closely at this issue. It would surely have come up once experts got to the stage of detailed discussion of how to contain the samples in practice, working out protocols with the details needed to construct a Mars Receiving Facility, but these discussions haven't happened yet.
Issue of lab leaks already raised by an expert in infectious diseases under NEPA but NASA's team didn't recognize its significance
Though NASA didn't raise this issue in the PEIS, as we saw, William Agger, an infectious disease microbiologist did raise it. So we already have one public comment about lab leaks by an expert already on a not very well publicised PEIS. We can be certain that this question of how to handle lab leaks will come up again just as it did or Apollo.
William Agger: In addition, even a level 4 biosafety lab on Earth or even on the Moon, is of great concern to this Infectious Disease/Clinical Microbiologist. Even at the protection level of biosafety 4 labs have not been, nor will be, without accidents. What would society do, for instance, if researchers in a Utah lab or research astronauts in a biosafety 4 lab on the Moon, were in a lab accident?
What could be done for them and ethically with them? Bring them back to earth and/or let them out of the lab, potentially contaminating our biosphere?
In short, it's a scientific gamble with a small upside against a catastrophic downside for the living people on the Earth and to the only biosphere they will survive on. Finally, I am not opposed to looking for life on Mars. If found, it should be left on Mars and studied there.
(Agger, 2022, Comment Submitted by William Agger)
NASA reply to William Agger that the details are for discussions later between NASA and the CDC and NIH. They reassure him that everything will be fine because we have existing protocols for known infectious diseases:
NASA: High-containment laboratories around the world have existing protocols for maintaining staff safety and addressing possible exposures to known infectious substances. The MSR program would develop specific plans and procedures for safe handling of the Mars samples based on these protocols and related lessons learned—including what to do in the case of breach of containment—in close consultation with experts at organizations such as the Centers for Disease Control and the National Institutes of Health. These plans will prioritize staff health and safety, and open communications with local, state, and federal organizations, as well as with the general public.
(NASA, 2023. Mars Sample Return final PEIS : B-30)
However they are talking to an expert here, someone well aware of those protocols
As we mentioned before, the issue with NASA's reply here is the "known infectious substances". Potential alien pathogens are the epitome of unknown never encountered infectious substances. That is what leads to Carl Sagan's "vexing question".
Typhoid Mary had no endpoint to her isolation, no isolation period. She continued as a symptomless carrier capable of spreading typhoid throughout her life. Her isolation only ended with death. See above:
So what is the solution? Quarantine any technicians contaminated by lab leaks for the rest of their life, as for Typhoid Mary? Even that is not realistic even if they consented, because of human rights issues, and also because if they have other medical issues or if there is a fire or other emergency it must be permitted to remove them from isolation. See above:
There seems to be no solution to this conundrum.
Instead we may have to find a way to avoid any possibility of human technicians getting infected. But that is impossible in a lab run by human technicians.
It might be possible to solve this with a fully telerobotic laboratory. It wouldn't eliminate equipment failures and leaks but it could be designed so that the leaks only contaminate telerobots. However we have never designed or built such a laboratory before and it may have many issues and may be very expensive.
This is where the suggested alternative of a life detection lab above GEO comes in. It is likely to be a much lower cost solution that is resilient to any quarantine period and any level of assurance and size limit, and would likely to cost far less than a fully telerobotic laboratory. But let's look at proposals for a terrestrial facility first. I found only one telerobotic design so far in the literature on planetary protection for a Mars sample return.
A Mars Receiving Facility has unique challenges and a first of its kind fully telerobotic laboratory would be costly (over half a billion dollars) and a major challenge even for the National Institutes of Health (NIH)
All NASA says about the Mars Receiving Facility design is:
NASA’s concept for the SRF is to build a facility that can be characterized as a BSL-4 equivalent facility. The facility would nominally incorporate the designs and procedures of a BSL-4 facility (which has significant security requirements) and possibly, as yet undefined, additional cleanliness and protective measures
(NASA, 2023. Mars Sample Return final PEIS : 3-13)
In previous design studies those "possibly, as yet undefined, additional cleanliness and protective measures" have added considerably to the cost, making it more than four times more expensive than a conventional biosafety laboratory.
The complicating issue here is that unlike a normal biosafety laboratory, the laboratory must also function as a clean room, to keep out terrestrial contamination. The two types of facility, a clean room and a biosafety lab, have opposite requirements, and it is not easy to simultaneously keep out contamination and also to prevent the sample materials escaping containment.
Also since the requirements for the Mars Receiving Facility are novel, never built before, time is needed to plan it, build it and train the technicians to run it and make sure they have the experience needed to run the facility when the samples return.
Starting from the time when we already have the interagency panel set up and functioning and have worked out the requirements:
- One estimate for the timeline to build the facility is 11 years including an extra two years to train
technicians, divided up as: (Uhran et al., 2019, Updating Planetary Protection Considerations and Policies for Mars Sample Return : table 2)
- Establish facility requirements 2yrs;
- facility design 2yrs;
- construction 3yrs;
- commissioning 2yrs.
- train technicians 2yrs
Although there still is no facility in existence anywhere that combines the requisite biocontainment levels, cleanliness conditions, instrumentation, and other features needed for the characterization and testing of returned martian samples, there is an increasingly clear understanding of what will be required and how it can be accomplished
It has been estimated that the planning, design, site selection, environmental reviews, approvals, construction, commissioning, and pre-testing of a proposed SRF will occur 7 to 10 years before actual operations begin. ....
In addition, 5 to 6 years will likely be required for refinement and maturation of SRF-associated technologies for safely containing and handling samples to avoid contamination and to further develop and refine biohazard-test protocols. Many of the capabilities and technologies will either be entirely new or will be required to meet the unusual challenges of integration into an overall (end-to-end) Mars sample return program
(SSB, 2009, Assessment of planetary protection requirements for Mars sample return missions : 59) - The 2010 decadal review estimated the cost of the Mars sample receiving facility as $471
million in 2015 dollars (Mattingly, 2010, Mission Concept Study, Planetary Science Decadal Survey, MSR Orbiter Mission
(Including Mars Returned Sample Handling)) or $659 million dollars today.
Others estimate over half a billion dollars in 2015 dollars (Andrews, 2020, The Plan to Bring Mars Down to Earth). - The only designs looked at so far are based on the 1999 size limit, essentially a BSL-4 but with
the challenge that the samples need to be kept free of terrestrial contamination. The three main approaches for a lab staffed by human technicians are a clean
room inside a BSL-4 samples well protected), a BSL-4 inside a clean room (personnel well protected)
or a novel double wall construction (both samples and personnel well protected), all of which are new technology (Carrier et al., 2019,
Science-Driven Contamination Control Issues Associated with the Receiving and Initial
Processing of the MSR Samples : Figure 4) (Uhran et al., 2019, Updating Planetary Protection Considerations and Policies for Mars Sample Return : table 2):
- a clean room inside a biosafety level 4 facility
(personnel not well protected from samples) - a biosafety level 4 facility inside a clean room
(samples not well protected from personnel and terrestrial life), - a biosafety level 4 facility surrounded by a vacuum barrier inside a clean room in a novel
triple wall facility
(protects both sample and personnel)
(Figure 4 of (Carrier et al., 2019. Science-Driven Contamination Control Issues Associated with the Receiving and Initial Processing of the MSR Samples) adapted from (Rummel et al., 2002, A draft test protocol for detecting possible biohazards in martian samples returned to Earth : Figure 1)
The last of those three is the only one that protects both the personnel and the samples optimally and involves a novel triple wall structure.
the three ways of containing a returned sample.
This shows how the inner double wall of the triple wall system works: schematic illustration of double wall system to both isolate and contain the sample, Credit NASA / FLAD (Hsu, 2009, Keeping Mars Contained, NASA Astrobiology Magazine)
As examples of these types of design:
- EURO-CARES (Hutzler et al., 2017, EURO-CARES Extraterrestrial Sample Curation Facility: Architecture as an enabler of science) consists of a clean room inside a BSL4 so the staff need extra protection from the samples, which they provide with personal protection equipment suits.
- Calaway et al proposed a lower cost mobile laboratory that could be attached to existing
biosafety labs, and this is a BSL4 inside a clean room so there’s more risk of
contamination of the samples (Calaway et al., 2017. Mobile/Modular
BSL-4 Facilities for Meeting Restricted Earth Return Containment Requirements)
- The FLAD team concept is an example 3-wall design which also included a proposed concept for novel double walled glove boxes with the pressure reduced inside the double wall so this protects both samples and personnel (Beaty et al., 2009. Planning considerations for a Mars sample receiving facility: Summary and interpretation of three design studies
- a clean room inside a biosafety level 4 facility
However all three of those would use lab technicians and would need precautions for lab leaks.
I can find only one design in the literature that wouldn't need to use quarantine to handle lab leaks, at least within the facility. That is the LAS design for a fully telerobotic Mars Receiving Facility, one of three designs submitted to NASA in 2009. The LAS study relies on telerobots to do almost all the sample handling. (Beaty et al., 2009, Planning considerations for a Mars sample receiving facility: Summary and interpretation of three design studies:75). So we could do it already in 2009, and telerobotic capabilities have improved since then.
Sketch of telerobotic facility Credit NASA / LAS (Hsu, 2009, Keeping Mars Contained)
The 2010 decadal review estimated the cost of the Mars sample receiving facility for any of the three designs submitted as $471 million in 2015 dollars (Mattingly, 2010, Mission Concept Study, Planetary Science Decadal Survey, MSR Orbiter Mission (Including Mars Returned Sample Handling)) or $659 million dollars today. Others estimate over half a billion dollars in 2015 dollars (Andrews, 2020, The Plan to Bring Mars Down to Earth).
To put this in context the 2007 NIAID Integrated Research Facility with space for 30 researchers using BSL-2, BSL-3 and BSL-4 facilities cost $105 million (Bell laboratories, n.d., Integrated Research Facility, NIAID).
So the cost of a Mars Receiving Facility as envisioned in 2010 was substantially more than the cost of a normal BSL-4.
These designs were all based on the 1999 size limit, which is essentially a BSL-4 in containment requirements. However they had the additional challenge that samples need to be kept free of terrestrial contamination. The three solutions mentioned, a clean room inside a BSL-4, a BSL-4 inside a clean room or a novel double wall construction, all of which are new technology (Carrier et al, 2020, Mars Extant Life: What's Next? Conference Report (html) : Figure 4)
Adding the ESF requirement would likely increase the cost considerably since it wouldn't be possible to use HEPA filters, so it would be necessary to fund research to find a replacement that can achieve the desired containment levels.
This would also extend the timeline since we first need to develop the technology to contain ultramicrobacteria. It is also necessary to devise practical tests the operators can use to test the filters or air incinerator or other new technology to see if it needs replacing, and to repair it, and replace it. All this has to be done in a way that ensures no risk of a single particle of 0.05 microns or larger escaping the facility. See above:
Also as we saw NASA doesn't have the in house expertise to design, build and run such a laboratory. Given that NASA's current Mars Sample Return team don't yet have anyone familiar with the basics of how HEPA filters are used for biosafety, they will need to recruit new members before they can be competent to design, build and run such a facility, or get another agency to build it and run it for them.
See above:
It is impossible to know how easy or difficult it would be to make a fully telerobotic facility that can eliminate the risk of lab leaks with a reasonable degree of confidence.
There are several new issues to look at once we have to consider microbes that can persist indefinitely after a lab leak, such as a novel fungal genus or mirror life. This adds new issues such as how to sterilize the facility when decommissioned and how to repair the telerobots, filters, etc. When those are combined with the more challenging ESF size limit beyond the capabilities of HEPA filters, the result seems likely to be very expensive.
This book looks at the idea of a terrestrial telerobotic laboratory below. It appears to be technically possible if there is no limitation on the funds available to construct it and unlimited time to develop and test the technology, but it may be hard to do with a reasonable budget and to complete it by the 2030s:
We've seen in this section that even without additional requirements, the general picture is over a decade for the timeline. Adding the need for novel technology which we don't have yet and the need to make it affordable it may take longer than that.
See below: Timetable tight to complete a sample return to a ground based lab before the 2040s; while ESA and NASA can achieve considerable cost savings for their sample retrieval spacecraft launched this decade with an early decision to return them to a life detection lab above GEO
Miniature life detection lab above GEO: a teleoperated alternative that plays to NASA's strengths, modelled on its proposed in situ Europa life detection lander
This proposal is based on a mission design by NASA from 2017 to study life in situ on Jupiter's Moon Europa. My attachment 8 explained this, but sadly NASA prescreened that as non substantive and so didn't see it.
The proposal combines the in situ life detection above GEO with a Mars gravity simulation centrifuge so instruments can operate in Mars gravity conditions and any attempts to grow life returned from Mars can replicate surface conditions very exactly, better than any simulation chamber we can build on Earth. See next section:
The reason for choosing an orbit above GEO is because it is about as far as it is possible to get by way of delta V from Earth or the Moon. The main issue is whether any debris from the satellite could end up spreading into other orbits under the influence of the sun, Moon and solar radiation pressure. There are "frozen orbits" that may be safe enough, proposed for disposal orbits for Geostationary Earth Orbit satellites.
If not there are other potential safe orbits to consider including the point of balanced on the far side of the Earth from the sun called the Earth Sun L2 position. The advantage of above GEO, if it is possible is that it is far closer to Earth with a fraction of a second latency compared to 10 seconds round trip time for the Earth Sun L2 and a longer trip and more rocket fuel to access it. However that is still far more accessible than a life detection mission on Mars.
In 2017, scientists were already confident they could do life detection as ar away as Europa, if present, with a small life detection lab of less than 50 kilogram. it would have to be operated remotely from Earth with a round trip latency of over an hour between sending a signal and getting a response (Jupiter at its closest has a distance of 588 million km (365 million miles) or about 32 light minutes 42 seconds (Earth Science Teachers Association, n.d., Jupiter statistics)) [find better source]
The Europa Lander mission concept is designed to achieve ground-breaking science. The SDT (Science Definition Team) is confident that a payload matching or exceeding the requirements described herein could potentially reveal signs of life on Europa (Hand et al., 2017, Report of the Europa Lander Science Definition Team : xi).
.
Text on graphic: Europa Lander (NASA proposal from 2017)
Why not return Mars samples to a miniature lab like this .... but above GEO?
Keeps Earth 100% safe from Mars microbes
(adds centrifuge for artificial gravity)
Europa lander: total mass 42.5 kg.NASA's idea for a life detection lab on Jupiter's moon Europa
Graphic from (NASA, 2017, Europa Lander Study 2016 Report)
Given that we can do this as far as Europa with 2017 technology we can certainly do it above GEO with technology advanced by 7 years since then.
Unlike a terrestrial lab it is easy to build in end of mission sterilization. Add a small onboard heater and pass all the solar power through that heater. The vacuum of space is so insulating, like a vacuum flask, the main problem is usually heat rejection to get rid of waste heat. So the satellite would quickly heat up to 300 C and then would be sterilized. The engineering details seem likely to be easily surmounted.
So if anything happens to the satellite that is off nominal such as straying into the wrong orbit, it can be sterilized. It could also be set to auto-self sterilize in some circumstances if it loses connection with Earth for a long period of time.
We can send tons of equipment to above Geostationary Earth Orbit (GEO) with the same size of rocket as can send 50 kilograms to land on Europa [check details] and with a roundtrip latency of about half a second [ADDCITES].
It would be returned to above GEO in a safe inclined orbit in the Laplace plane so it can't contaminate satellites in GEO
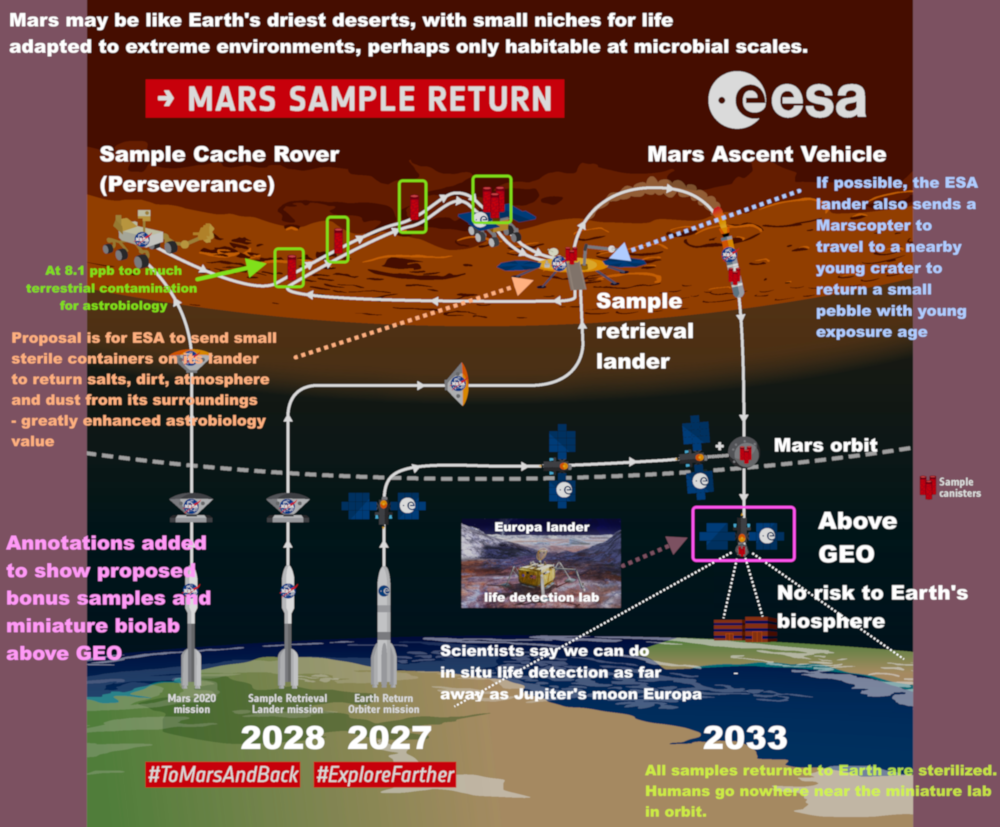
Text on graphic:Mars may be like Earth's driest deserts, with small niches for life adapted to extreme environments, perhaps only habitable at microbial scales
At 8.1 ppb too much terrestrial contamination for astrobiology
ESA sends small sterile containers on its lander to return salts, dirt, atmosphere, and dust from its surroundings - greatly enhances astrobiology value.
If possible, the ESA lander also sends a Marscopter to travel to a nearby young crater to return a small pebble with young exposure age.
Above GEO
No risk to Earth's biosphere.
Scientists say we can do in situ life detection as far away as Jupiter's moon Europa
All returned samples are sterilized
humans go nowhere near the satellite.
Graphic obtained by modifying the ESA graphic, (Oldenburg, 2019, Mars Sample Return overview infographic)
No humans ever go anywhere near it and anything returned to the Earth's biosphere is thoroughly sterilized.
Those bonus samples are needed because NASA has permitted a level of contamination with terrestrial life that makes the Perseverance samples almost certainly of no interest to astrobiology past or present as we'll see. We can transform this into a mission of significant interest as a first step in astrobiology with the bonus samples.
This proposed alternative submitted to NASA under NEPA uses a centrifuge for Mars gravity in an excellent Mars simulation chamber, which also means instruments can operate under Mars gravity
I made this proposal to NASA in a NEPA comment, which is relevant to the PEIS because NASA are required to consider any alternatives submitted in this way
12. Quarantine of humans can’t keep out a fungal disease of crops, mirror life, etc.
13. So any unsterilized samples will need to be studied remotely via telerobotics which also greatly reduces forwards contamination (issues with filtering ultramicrobacteria will go both ways).
14. astrobiologists now have tiny instruments that can go from sample preparation to life detection, even to a gene sequence, operated remotely on Mars. They could send hundreds of these in each 7 ton payload of the Ariane 5 to above GEO.
Let's make this an even better mission and SAFE for Earth.(Walker, 2022, Comment posted December 20th)
However we never got to see any assessment of the proposal by NASA. That's because NASA's Mars Sample Return team prescreened it as impossible based on out of date information about the capabilities of in situ instruments from over a decade ago as we will see in the next section:
In my proposal the satellite is of special interest for the bonus samples as these can be studied without the noise of forward contamination by terrestrial organics:
7. The high levels of forward contamination make astrobiology almost impossible. I recommend bonus samples of dirt, dust and atmosphere collected in a STERILE container with no terrestrial organics, brought to Mars especially on the ESA fetch rover.
8. I recommend returning these bonus astrobiology samples to a safe orbit above GEO where they can be tested for life(Walker, 2022, Comment posted December 20th)
For the forwards contamination issue see above:
Cassie Conley also raised the issue that instruments that do fine in low gravity often fail to work properly in zero gravity.
Cassie Conley: “Building instruments to operate in microgravity is extremely challenging, and the reliability, sensitivity and accuracy of microgravity instrumentation is much lower than the same instruments on Earth. The sorts of instrumentation failures that often happen on the ISS would make this impossible.”
(Marks, 2022.Calls grow for a safer Mars Sample Return)
In my proposal, the bonus samples would be returned to a very excellent Mars simulation chamber, rather like BIOMEX on the outside of the ISS but able to replicate martian gravity, and daily and seasonal cycles.
Text on graphic: Bonus samples in STERILE containers returned to satellite perhaps 50,000 or 100,000 km above GEO in what would be Earth’s ring plane if it had a ring system.
- NOT for safety testing
- Returned for astrobiological study - nexus of expanding off-planet astrobiology lab.
- Minimal forward contamination.
- Humans nowhere near this.
- Centrifuge to replicate martian gravity.
Many instruments placed in centrifuge along with the dust and operated remotely from Earth.
Graphic shows: (Clark, 2018. NOAA’s new GOES-17 weather satellite has degraded vision at night) just to have an image of a geostationary satellite, not that it would be a $2.5 billion dollar satellite. SETG from (Mojarro et al., 2016.SETG: nucleic acid extraction and sequencing for in situ life detection on Mars). Astrobionibbler from (Elleman, 2014. Path to Discovery) and a proposed ISS centrifugal motor for plant experiments, dialable to any level from microgravity to 2g which was going to be a component of the Japanese Centrifuge Accommodation Module (NASA, n.d., Centrifuge Rotor [biology experiment on the ISS]). This was designed and built but never flown to the ISS (Poplawski et al., 2014, Analysis of Space Station Centrifuge Rotor Bearing Systems: A Case Study : 15)
- Chiral labeled release.
- SETG from sample acquisition through to DNA sequence all automated in 2 units, each can be held in palm of hand.
- Astrobionibbler microfluidics can detect a single amino acid in a gram of sample
This uses a centrifuge for an excellent Mars simulation chamber. Though the larger Centrifuge Accommodations Module was never flown to the ISS, Japan has done a series of experiments with mice reared on the ISS in microgravity, full 1g artificial gravity and lunar gravity (Hagiwara et al., 2014, Development of Mouse Habitat Unit for Use in “KIBO” Japanese Experiment Module on International Space Station). (JAXA, 2019, World's first long-term habitation of mice on the International Space Station in a gravitational environment simulating the Moon! -- A first step toward expanding human activities into deep space)
The approach is similar to BIOMEX on the exterior of the ISS but much larger, with martian gravity. It could have day / night and seasonal cycles and be used to simulate conditions anywhere on Mars for both forward contamination simulation studies and attempts to get any life detected on Mars to grow.
It would use filtered light directly from the sun piped into the chamber perhaps using quartz based light tubes which would let it replicate the UV component as for BIOMEX in a way that we can't replicate on Earth. It would also have an ionizing radiation flux similar to the surface of Mars. It could be shielded to reduce the flux to levels identical to the martian surface. In this way it would have the advantages of BIOMEX over terrestrial Mars simulation chambers but with the addition of Martian gravity and far more flexibility to simulate the martian surface conditions in rather simple ways such as varying the light intensity to simulate day - night cycles and varying humidity.
Text on graphic: Mars simulation chamber based on ideas behind BIOMEX / EXPOSE R-2
Mars pressure atmosphere exposed to natural sunlight filtered to resemble Mars surface conditions, and natural ionizing radiation
- but done inside a small centrifuge which provides Mars level artificial gravity for instruments to use to study the samples and for extra fidelity Mars surface simulations.
Background graphic: in 2014, Russian cosmonauts Maxim Suraev (gloves visible at middle left, and top towards the right) and Aleksandr Samokutyayev removed the cover for BIOMEX to expose it to the natural sunlight.
(DLR, 2014. The EXPOSE-R2 experiment system with BIOMEX on the ISS).
It could have adjustable filters and blinds simulating day night cycles on Mars, and even seasonal cycles with UV levels as for Mars by simply filtering down the sunlight to half terrestrial intensity and then filtering out more of it to simulate the effect of the Martina atmosphere. Then more filters could simulate the shading and UV shading in dust storms.
The simulation chamber could be shielded from cosmic radiation and solar storms to a level needed to approximate the ionizing radiation levels on the surface of Mars. Water vapour could be added / removed to help approximate the seasonal cycles on Mars and the day night pressure and humidity cycles would happen to an extent automatically by the warming and cooling. Perhaps even the Martian frosts could be simulated and the pressure / temperature gradients over the near Martian surface?
In this way it could become a facility also for studies of terrestrial analogues similarly to BIOMEX but dedicated to Mars simulation chamber studies 24/7. The simulated Mars gravity, the natural cosmic and solar ionizing radiation and the natural sunlight would make it far more straightforward to simulate Mars surface conditions to far greater fidelity than we can ever do on Earth.
Fluid and gases can be transferred to / from the rotor from outside to inside the chamber using fluid slip rings (Hangzhou Grand Technology, 2023. Understanding Fluid Slip Rings: An In-depth Examination)
It doesn't need to be large or elaborate. This is an example design based on a cubesat
Text on graphic: Design for a cubesat based centrifuge intended for use in the ISS to explore plant growth in artificial gravity from asteroids up to Mars gravity.
This could be adapted to make a Mars simulation chamber for samples returned from Mars with instruments to examine the samples in the simulation chamber.
Illustration by A. Chandra and J. Thangavelautham
from: (Asphaug et al., 2017. A cubesat centrifuge for long duration milligravity research).
This centrifuge would likely be a collaboration between teams from the USA,Germany, and other places that have worked on Mars simulation chambers. This would likely have zero cost to NASA. They would be eager for the opportunity to build a Mars simulation chamber to function in space because of the excellence of the simulation possible in space conditions as with the enthusiasm there was for the BIOMEX experiment.
The Mars simulation chamber centrifuge would likely be designed and built as an international collaboration which would be likely to include the Michigan group lead by Nilton Rennó with one of the most sophisticated Mars simulation chambers in the world. DLR of Germany who also do advanced Mars simulation experiments, and JAXA because they designed and built an entire centrifuge module for the ISS which sadly never flew and remains in a parking lot in Japan(NASA, n.d., Centrifuge Rotor [biology experiment on the ISS]) (Poplawski et al., 2014, Analysis of Space Station Centrifuge Rotor Bearing Systems: A Case Study : 15)
In this way we can study the returned samples including any martian salts, dirt and dust in close to the natural conditions on Mars. We can also use those to construct more accurate regolith analogues and test terrestrial life in the regolith analogues in separate chambers again in very close to Mars surface conditions.
The main challenges are already solved. (ADDCITES)
- Satellite above GEO: Many designs for spacecraft in GEO with solar panels to supply solar power, resilient to the ionizing radiation and with a long lifetime. The delta v to get to a higher orbit above GEO is small compared to the delta v from low Earth orbit to GEO and would have almost no effect on the payload
- Autonomous docking: Russia has done this for decades with the Soyuz Progress supply ships to the ISS. DARPA achieved it for the first time for the USA in 2007 (Young, 2007. US achieves autonomous docking in space) and the SpaceX supply ships to the ISS do autonomous docking. ESA and others are working on spacecraft that can even dock with spacecraft without a docking port for de-orbiting to tackle the space debris problem. Then there's the work on servicing satellites in GEO and the Earth Return Orbiter will need to rendezvous autonomously with the M
- Robotic handling of the samples: to move them around within the satellite and to supply the samples to the experiments, a mature technology used with many robotic spacecraft to other planets but easier to do above GEO because of the low latency. Using robotic arms and handlers, can also use microfluidics.
- Sterilization: the challenge here is to sterilize samples and any other material returned to Earth while protecting the electronics in the space-craft as well as in the supply / servicing vessels. The satellite will need an airlock to contain a Mars simulation atmosphere inside it. So one way to do this is to line the airlock with lead or some other material to block ionizing radiation, another way is to focus the ionizing radiation so it radiates outwards only towards the airlock entrance.
The sterilization could be done with cobalt 60 or more flexibly with low power requirement nanoscale X-ray emitters, a mature technology which has the advantage it is tunable and can be switched on and off and can also be focused in a particular direction without need for large amounts of shielding to block it in other directions. Samples would be placed in the airlock and then the airlock sealed on the inside, then sterilized before the servicing spacecraft comes to pick them up. The servicing spacecraft would than add any new supplies into the airlock and remove the sterilized samples to return to Earth. It then leaves the airlock and is not affected by sterilization since it is switched off at that point. Then any supplies would be sterilized to remove terrestrial contamination.
The sterilization could be done using Cobalt 60 or possibly using the new low power carbon nanotube emitters for X-ray sterilization. See: - Mars simulation centrifuge: this uses a combination of several mature technologies with small centrifuges already used in space for artificial gravity experiments involving mice in microgravity, full 1g artificial gravity and lunar gravity (Hagiwara et al., 2014, Development of Mouse Habitat Unit for Use in “KIBO” Japanese Experiment Module on International Space Station). (JAXA, 2019, World's first long-term habitation of mice on the International Space Station in a gravitational environment simulating the Moon! -- A first step toward expanding human activities into deep space) .
We would have nearly a decade to work on the design and build the satellite as it only needs to reach orbit in time to receive the first samples from Mars in the early 2030s.
- A miniature lab above GEO can cost less than a Mars receiving facility on Earth - five times the payload for a mission to Mars for the same launch cost with far less complexity
- How a miniature life detection lab above GEO can avoid the technological uncertainties of a fully telerobotic biosafety lab on Earth, at significantly lower cost, with a significantly higher technological readiness level
This proposal also seems better for the time sensitive geological studies. The sample tubes could be opened in the satellite in a chamber pressurized to martian atmospheric pressure and preliminary studies done telerobotically on them, just as they would be done on Mars but with almost no latency. The headspace gases are likely to be of no or almost no scientific value and may even be lost altogether on the journey back depending on the quality of the seal and how much it's affected by any dust that gets into the seal as we'll see below:
Tosca et al. found many issues involved in opening the samples in chambers at terrestrial atmospheric pressure. The samples would have been altered already during the return journey by the temperature fluctuations and when they are exposed to gases at the pressure of Earth's atmosphere will start to change over a period of weeks. Many measurements would need to be done quickly before they are altered in this way, as outlined by Tosca et al. (Tosca et al, 2022, Time-Sensitive Aspects of Mars Sample Return (MSR) Science). If some of these measurements can be done in an orbiting satellite in a Mars pressure atmosphere that may lead to more accurate measurements and could be used to calibrate any measurements made on sterilized samples returned to Earth. So there are reasons in favour of opening the sample tubes in the satellite rather than after return to Earth.
This would make sterilization in space easier too as we can remove samples and study them in orbit first and then sterilize subsamples or the entire contents of the tube for return to Earth. The initial sample curation may be best done in orbit especially if sterilization is done prior to returning the samples to Earth, as this is the only way to do any sterilization sensitive studies
This solution of a satellite above GEO is one way NASA could resolve this, playing to their strengths, without the need for a NASA run biosafety program to protect Earth or biosafety lab and it has many potential benefits for the science value of the mission which would seem to deserve closer examination.
It's not playing to NASA's strengths to build and run a biosafety laboratory or to design a biosafety plan to protect Earth. We see this from this PEIS, from the treatment of the Mars meteorite argument and their response on HEPA filters and their argument from ten example diseases to near zero risk of pathogens.
However NASA can be expected to do an excellent job of providing a telerobotic life detection lab above GEO.
NASA's screening process for alternatives based on life detection in situ or in a robotic satellite lab was outdated and missed the in situ life detection instruments listed in its own 2016 Europa lander proposal
In my comments under NEPA I suggested this alternative miniature life detection lab above GEO based on NASA's own Europa lander mission plan from 2016. Their scientists assure us it is feasible to run such a lab on Europa.
Although not yet peer reviewed, I felt the idea was developed enough in my preprint to be submitted as a reasonable alternative under NEPA for NASA to consider.
However NASA never looked at this suggestion.This was because they relied on a survey of in situ life detection capabilities from 2008 to prescreen out any alternative that didn't do life detection in terrestrial laboratories, in "2.3.1 Programmatic Alternative Screening Criteria".
The International Mars Architecture for the Return of Samples Working Group, in 2008, evaluated the overall goals and objectives of Mars exploration and determined that, given the scope of what is realistically achievable via in situ exploration technology, a significant fraction of these investigations could not be meaningfully advanced without returned samples for the following reasons (iMARS Working Group 2008, Meyer et al. 2022):
(NASA, 2023. Mars Sample Return final PEIS : 3-3)
Their 2008 cite (MARS Working Group, 2008. Preliminary Planning for an International Mars Sample Return Mission : 9) predates all the more modern in situ instrument designs. Their 2022 cite (Meyer et al, 2022, Final Report of the Mars Sample Return Science Planning Group 2 (MSPG2)) doesn't seem to have any discussion of in situ instruments.
Based on this 2008 cite they prescreened all the suggestions to do life detection outside of Earth's biosphere as not a valid alternative. Here is their conclusion for my proposal:
Robert Walker: If there is a significant chance of viable present- day life in these samples, we suggest returning them to a satellite in a stable inclined orbit above GEO in Earth’s Laplace plane or “ring plane.
(NASA, 2023. Mars Sample Return final PEIS : B-58)
NASA: Utilization of the ISS, as well as analysis of samples in orbit and on the Moon, are alternatives considered but not carried forward for reasons as discussed in Section 2.3.1.1 of the PEIS.
(NASA, 2023. Mars Sample Return final PEIS : B-31)
And in more detail:
Robert Walker: Suggestion: the geological samples can be sterilized and returned directly to Earth and the suggested dust, atmosphere and dirt samples collected in 100% sterile containers can be returned to a satellite above GEO for the astrobiological studies.
(NASA, 2023. Mars Sample Return final PEIS : B-54)
NASA: As discussed in Section 1.3 of the PEIS, the complexity and cost of sending advanced instruments to study Mars in place (in situ) would restrict the scope and detail of the science that could be done; many important classes of scientific instruments are not amenable to the miniaturization and ruggedization that would be necessary to operate from a spacecraft. An important aspect of this is that many critical measurements can only be done on samples that have been through intricate sample preparation processes, and most of those processes are not able to be automated. Additionally, Section 2.3.1 of the PEIS discusses the limitations associated with a remote or in-orbit sample safety assessment, which was an alternative considered but not carried forward.(NASA, 2023. Mars Sample Return final PEIS : B-30)
The Mars sample return team were not aware of the survey from 2016 of in situ instrument capabilities for their own agency's Europa lander mission proposal (Hand et al., 2017, Report of the Europa Lander Science Definition Team).
NASA could have found out about their own Europa mission proposal from my attachment 8 which went into great detail about the instruments that can already be miniaturized and sent to above GEO based on their Europa lander proposal as the main source. This is in the section titled: "Modern miniaturized instruments designed to detect life in situ on Mars - could also be used to examine returned samples in an orbital telerobotic laboratory" (Walker, 2022, NASA and ESA are likely to be legally required to sterilize Mars samples to protect the environment until proven safe) as uploaded in the version submitted to the final round of public comments.
I continue to make minor fixes, and the latest version is at: (Walker, 2023, NASA and ESA are likely to be legally required to sterilize Mars samples to protect the environment until proven safe).
However, NASA's team prescreened this preprint as "nonsubstantive" including the chapter that details the modern instruments that are now miniaturized and able to be sent to above GEO.
NASA gives no explanation for this decision. It is just listed as non substantive in a list along with others they excluded, with no explanations.
Text on graphic: Instruments for lab above GEO, lab leak issues, quarantine issues for human technicians etc.
By dismissing my attachment 8 as "Nonsubstantive" NASA's team excluded the material needed to evaluate the suggested reasonable alternative of a miniature lab above GEO.
Graphic shows a screenshot from (NASA, 2023. Mars Sample Return final PEIS : B-28)
However, from the context of the rest of the prescreening criteria in the PEIS the most likely explanation was that the main focus of the preprint is on this proposal to return the samples to above GEO. Whatever the reason for excluding it, this means NASA never got to see its list of modern in situ instruments. NASA also never got to see its discussion of the issues with quarantine which was one of the main reasons for proposing return to a miniature lab above GEO. I don't know of any other reason why NASA would prescreen it out as nonsubstantive except for the proposed solution to the issues of planetary protection for a Mars sample return.
There is. a NEPA requirement to include a summary that identifies all alternatives submitted by public commenters
The final environmental impact statement shall include a summary that identifies all alternatives, information, and analyses submitted by State, Tribal, and local governments and other public commenters for consideration by the lead and cooperating agencies in developing the final environmental impact statement.
§ 1502.17
The Council on Environmental Quality also says an agency has to consider alternatives that don't fully meet all its goals.
An agency also has to look at alternatives that don't meet the applicants listed goals but better meet the policies and requirements set forth in NEPA and the agency's statutory authority and goals
(CEQ, 2022, National Environmental Policy Act Implementing Regulations Revisions - a rule by the CEQ on 4/20/2022)
See Supplementary information under :
- Fails NEPA requirements to consider reasonable alternatives that don't fully meet the agencies listed goals but better meet NASA and NEPA policies and requirements
- NASA also required all alternatives to test unsterilized samples for present day and past life in a terrestrial laboratory based on out of date information about in situ capabilities
In this case it seems to have been left out improperly due to relying on out of date science about the potential instruments we can send to above GEO. So now let's look at those instruments.
Modern miniature life detection instruments that could fly to above GEO, or to Mars
As mentioned in the previous sections, in my comments under NEPA I suggested an alternative miniature life detection lab above GEO based on NASA's own Europa lander mission plan from 2016. Scientists assure us it is feasible to run such a lab on Europa.
This orbiting astrobiology lab need not be expensive for a robotic mission. It could be less cost than the Europa lander and the equivalent of one geostationary satellite far above GEO.
As we saw, scientists assure us it is feasible to run such a lab on Europa, so it has to be possible on Mars or above GEO (using artificial gravity to avoid problems of using the instruments in microgravity).
In this way humans that stay on Earth can study the dust, dirt and atmosphere remotely, just as they would on Mars using exquisitely sensitive in situ instruments designed with end to end automation from sample preparation to analysis - these already exist such as the
- Life Marker Chip LDChip300 (antibodies) almost sent on Exomars but descoped which was able to
discover a previously undetected microhabitat in the Atacama desert
(Parro et al, 2011 A microbial oasis in the hypersaline Atacama subsurface discovered by a life detector chip: implications for the search for life on Mars)
target mass of less than 1 kg (ESA, n.d., Life Marker Chip) - the gene sequencer SETG designed to do end to end sequencing on Mars with obviously no preparation
on Earth. By 2016 it reached the capability to detect 10 ppb of DNA. It would be able to detect 400
highly conserved “universal genes” such as ribosomal DNA which would establish whether
martian life is related to terrestrial life, perhaps by meteorite transfer, or is independently
evolved
(Mojarro et al, 2016, SETG: nucleic acid extraction and sequencing for in situ life detection on Mars)
SETG needs more work but is being actively explored by NASA for in situ detection for icy worlds (Carr et al, 2020, Nanopore sequencing at Mars, Europa, and microgravity conditions). It uses biodegradable organic nanopores at present with a short shelf-life, and NASA are exploring non biodegradable nanopores for longer missions to Mars and icy moons of Jupiter and Saturn, which would also help with issues of resupply of the nanopores to above GEO. They are investigating “nanogaps”, solid state nanopores that can also detect and distinguish amino acids - so they may be able to sequence proteins too (Maggori et al, 2023, Life detection and taxonomic characterization with MinION sequencing in Mars and icy worlds analogue environments : 230) - astrobionibbler able to detect a single amino acid in a gram
(Schirber, 2013, Searching for Organics in a Nibble of Soil)
(Noel et al., 2016, In Situ Microfluidic Subcritical Water Extraction of Amino Acids)
(Elleman, 2014, Path to Discovery) - a chiral version of the Viking labeled release experiment which feeds the experiment with normal
organics and separately with mirror organics, since life would most likely preferentially use
organics of one symmetry over the other.
(Anbar et al., 2012, A Chiral Labeled Release Instrument for In Situ Detection of Extant Life)
[I'm not sure what happens in the situation here life already has the isomerases to flip organics of the opposite symmetry to make it digestible - life on Mars may have these isomerases in order to cope with the organic infall from space so it might still produce a signal for both symmetries of the organics but it may well still have a preference] - An off-axis holographic microscope to let the focus be adjusted after the image is taken making it
easier to image individual microbes in a liquid medium
(Lindensmith et al, 2016, A submersible, off-axis holographic microscope for detection of microbial motility and morphology in aqueous and icy environments)
- which doesn't need a lens so can be included on a chip in microfluidics systems
"Extremely small, robust, low-power microscopes have been constructed using light-emitting diodes in an in-line DIHM geometry. This type of design is lensless and may be coupled to microfluidics to create compact on-chip systems. The advantages of this approach are very low mass and low power, insensitivity to alignment, and lack of mechanically sensitive optics. Field of view is also decoupled from resolution because there is no objective lens.
The field of view is 24 mm2 without scanning and the entire instrument weighs < 100 g.
Super-resolution has been achieved in these systems using LEDs arrays where each is sequentially illuminated … and a corresponding frame of data captured. In this case, the image from each LED is a slightly shifted hologram; the Fourier representations may be summed to achieve ~800 nm spatial resolution."
(Nadeau et al., 2018. Imaging technologies and strategies for detection of extant extraterrestrial microorganisms : 235) - A method to check for redox reactions directly by measuring the electrons and protons they
liberate. This is sensitive to small numbers of microbes and has the advantage that it could detect
life even if not based on carbon or any form of conventional chemistry we know of
(Ximena et al, 2010, Microbial fuel cells applied to the metabolically based detection of extraterrestrial life) - A miniature variable pressure electron microscope that combines imaging with in situ chemical
analysis
(Gaskin et al, 2012, Miniature variable pressure scanning electron microscope for in-situ imaging & chemical analysis)
This would be especially useful if we have already identified life with the other instruments and can bring candidates into the field of view of the microscope, or can cultivate martian life
"Sample throughput is a major drawback of electron microscopy - the imaged volume is so small that it would not be useful for the ‘search’ part of a life detection instrument, though if candidate objects could be identified and brought into the field of the EM it could be valuable for evaluation of features indicative of life."
(Nadeau et al., 2018. Imaging technologies and strategies for detection of extant extraterrestrial microorganisms : 237-8)
Several instruments suggested for Europa:
- Tests for autofluoescence. Aromatic amino acids (incorporating a ring of six carbons) fluoresce when stimulated with deep UV at wavelengths less than 250 nm. Chlorophyll and some other biological organics also autofluoresce
- We could also use fluorescent dyes that bond to specific macromolecules such as lipids, proteins and nucleic acids
- We can also use this autofluorescence to directly search for the activity of swimming microbes
- Raman microspectroscopy synchronized with visible light can do a chemical analysis of the microbes directly
- Superresolution optical microscopy, which can go beyond the usual optical resolution limit of 200 nm to observe nanobacteria
(Hand et al., 2017, Report of the Europa Lander Science Definition Team)
Literature search suggests systemic issue in NASA decision making: A review of the need to search for life in situ on Mars first, backed up by all the studies by astrobiologists
Astrobiologists have often warned that we need to do in situ searches first before we can intelligently select samples of value for past or present day searches. This is also the conclusion from the only white paper submitted by astrobiologists that I've found for the decadal survey in 2010 which was the basis for NASA's decision to prioritize a sample return mission from Mars, based on search for life as their top priority. This suggests a systemic issue of some sort in NASA decision making. Bada et al wrote:
In this White Paper we argue that it is not yet time to start down the MSR path. We have by no means exhausted our quiver of tools, and we do not yet know enough to intelligently select samples for possible return. In the best possible scenario, advanced instrumentation would identify biomarkers and define for us the nature of potential sample to be returned.
In the worst scenario, we would mortgage the exploration program to return an arbitrary sample that proves to be as ambiguous with respect to the search for life as ALH84001.
(Bada et al., 2009. Seeking signs of life on Mars: In situ investigations as prerequisites to a sample return mission : 1)
I.e. we first need to understand what is on Mars
In more detail Bada et al write:
1. MARS SAMPLE RETURN (MSR) SHOULD ONLY BE SUPPORTED AFTER THE PRESENCE OF BIOMARKERS HAS BEEN CONFIRMED.
Mars sample return missions would eventually allow for high precision measurements to be conducted with higher sensitivity, accuracy, and greater scope than is possible with in situ instrumentation. The major scientific drawbacks of such mission architectures would be the low achievable sample return masses (~350 grams), the fact that any returned sample(s) would only probe a minute geographical area on Mars, and the fact that no unequivocal evidence of biosignatures has yet been obtained to ensure that the returned sample would address the potential detection of extraterrestrial life.
There are serious community reservations about a rush to commit valuable scientific resources and funding to MSR until a valid scientific discovery has been made to justify investment … the in situ detection of localized biosignatures and an attempt at characterization of spatial variability as a function of depth or mineralogy would make a strong case as a valid scientific rationale on which to pursue expensive sample return ambitions. We feel that organic detection efforts over the next two decades via investment into advanced in situ robotic instrumentation are fundamental in support of a future intelligent MSR mission. Currently, MSR is regarded by much of the scientific community as largely weighted towards a technology demonstration as the rationale for good astrobiology will not be apparent until we discover more about our neighboring planet.
(Bada et al., 2009. Seeking signs of life on Mars: In situ investigations as prerequisites to a sample return mission : 2)
Bada et al. specifically recommend "follow the nitrogen". This is an essential atom for terrestrial life as it permits bonds that can be broken easily for life processes. But Mars doesn't have widespread inorganic nitrate deposits.
So - they suggest looking for organics associated with nitrogen.
It has been argued that while there are numerous abiotic pathways to carbon chemistry, the presence of nitrogenous compounds is specifically diagnostic for biogenicity on Mars, a planet that lacks widespread inorganic nitrate deposits [Capone 2006]. We endorse here a variation of the Follow the Nitrogen approach in which chemical characterization of highly specific nitrogenous compound classes would provide a method for unequivocal biosignature detection.
(Bada et al., 2009. Seeking signs of life on Mars: In situ investigations as prerequisites to a sample return mission : 2)
That by itself wouldn't be enough to detect samples suitable to return to Earth. They go on to suggest we look specifically for amino acids, amino sugars and other small molecules likely to be diagnostic of life. Amino acids occur naturally in meteorites (for instance) but then we'd notice if they all are in the same chiral form - either all the same as the terrestrial amino acid or all its mirror image. Amino acids on the surface or near the surface would soon be degraded by cosmic radiation but if buried sufficiently deep enough may remain to be diagnostic of likely life.
They say that we need to be able to detect low parts per billion or parts per trillion of amino acids.
Diagenetic pathways may have acted to degrade organic compounds over time due to the oxidizing surface conditions on Mars [Benner 2000]. For this reason, it is paramount that technologies exist for highly specific quantification of the target biomolecular compounds at trace levels, equivalent to low parts-per-billion (ppb) or parts-per-trillion (pptr) sensitivity.
Bada et al were involved in developing the Urey in situ life detector instrument package for ExoMars which sadly was descoped. So this next paragraph is based on their experience with field trials of Urey. They find that there are "incredibly pronounced" variations in biodensity at both the macroscale and the microscale.
Field studies carried out in 2005 as part of Urey instrument development efforts have shown that in extremely arid locations like the Atacama Desert, variations in biodensity are incredibly pronounced on both the macroscale and microscale [Skelley 2007]. If similar levels of biological heterogeneity were expected at one time on Mars, then it is probable that biosignatures could remain elusive during in situ investigation if instruments with inadequate sensitivity were utilized. Similarly, selection of a limited sample size could result in a null result for life detection during MSR missions and poses a high risk of ultimate failure
(Bada et al., 2009. Seeking signs of life on Mars: In situ investigations as prerequisites to a sample return mission : 7)
So even if there was life there in the past with similar abundance to the Atacama desert we would likely not find it unless the in situ instruments are sufficiently sensitive.
In my literature search almost all the other studies I found by astrobiologists came to the same conclusion including (Paige, 2000. Mars exploration strategies: Forget about sample return) and (Davila et al., 2010. New priorities in the robotic exploration of Mars: the case for in situ search for extant life). Even the one apparent exception, "Safe on Mars" from 2002 (SSB, 2002, Safe on Mars: Precursor measurements necessary to support human operations on the Martian surface : chapter 5 : 38), actually recommends in situ studies if our capabilities improve, as has happened since then.
NASA in its summing up for the decadal survey said the Mars community was emphatic in their view that we need to prioritize a Mars sample return:
The Mars community, in their inputs to the decadal survey, was emphatic in their view that a sample return mission is the logical next step in Mars exploration. Mars science has reached a level of sophistication such that fundamental advances in addressing the important questions above will come only from analysis of returned samples.
(Space Studies Board and National Research Council, 2012. Vision and voyages for planetary science in the decade 2013-2022 : 17)
NASA's decadal review cited the 2002 "Safe on Mars" paper in support of its conclusions. However this came from a time with a much less complex understanding of potential habitats for Mars, it seemed sterile on the surface, and a time with far less capable instruments for in situ studies (SSB, 2002, Safe on Mars: Precursor measurements necessary to support human operations on the Martian surface : chapter 5 : 38). Then on closer study, even their 2002 source said that in situ studies were best if the capabilities to search in situ improved:
"If such capabilities were to become available, one advantage is that the experiment would not be limited by the small amount of material that a Mars sample return mission would provide. What is more, with the use of rovers, an in situ experiment could be conducted over a wide range of locations."
This view wasn't a consensus even with the limited in situ instrument capabilities we had in 2002. Paige et al., writing two years earlier than “Safe on Mars”, in 2000 argued that we don’t know where to go on Mars to get the samples we need to answer central questions in astrobiology.
We don’t know where to go on Mars to get the samples we need to answer the life on Mars question ...
They argued for a search in situ first for both past and present day life
Phase 3. Deployment of Exobiologically-Focused Experiments, to provide detailed characterizations of the population of organic compounds, and to search for biomarkers of formerly living organisms, and extant life.>
Phase 4. Robotic Return of Martian Samples to Earth, to improve the characterization of organic compounds, and to verify any evidence for biomarkers and extant life discovered in Phase 3.
By 2009, at the time of Bada et al's paper, just 7 years later, instruments were already far smaller and more capable. Since then astrobiological instruments continued to get smaller and more capable, while our understanding of past and present day habitability of Mars gets more complex. The by then overwhelming case for in situ study for astrobiology continues to get stronger.
So this issue can already be seen in the decadal survey which originated this mission. NASA's summing up presented the opposite of the consensus statement from the eight astrobiologists who submitted that white paper.
Davilla et al. came to the same conclusion as Bada et al., in 2010:
… the immediate strategy for Mars exploration cannot focus only on past life based on the result of the Viking missions, particularly given that recent analyses call for a re-evaluation of some of these results. It also cannot be based on the assumption that the surface of Mars is uniformly prohibitive for extant life, since research contributed in the past 30 years in extreme environments on Earth has shown that life is possible under extremes of cold and dryness.
...
Sample return would be most efficient and logical once we have information from a variety of environments, particularly if evidence of extant or extinct life is found at any of these sites"
(Davila et al., 2010. New priorities in the robotic exploration of Mars: the case for in situ search for extant life)
Astrobiologists continue to make the same recommendations through to the present. Most recently in 2020:
Future missions would therefore benefit from the development of instruments capable of direct and unambiguous detection of extant life in situ, and improvements are needed in capabilities for sample preparation to optimize biosignature detection. Spacecraft resources should support a sufficient number of sample analyses to support replicate analyses, positive and negative controls.
Contamination control should be coupled with contamination knowledge so that Earth-sourced material can be eliminated as a possible source of any biological material discovered in Martian samples.
(Carrier et al, 2020, Mars Extant Life: What's Next? Conference Report (html) : 802)
This seems to be a systemic issue that needs to be addressed. How did NASA come to a conclusion that was the opposite of what the astrobiologists recommended in the only submission to the decadal review?
There seems to be a need to find a way to ensure the NASA management listen to experts who give advice that may sometimes make the engineering more complex or require major changes of plans, just as NASA need to be able to listen to the members of the public who pointed out NASA's many mistakes in the draft PEIS and need members on NASA's team with the necessary background and disciplines to respond to what they say.
This suggests something more systemic is needed than restoring the panels NASA closed down.
A new PEIS also needs to consider reasonable alternatives such as sterilizing the samples before they are returned to Earth
Indeed, the simplest solution is to sterilize all samples before they are returned to Earth.
In this case all that’s needed is
- A review of methods for sterilization adequate to sterilize even unfamiliar life that may be hardier even than radiodurans while preserving astrobiological interest, including using ionizing radiation or x-rays
The rock samples can simply be sterilized before they reach Earth during the return journey, or they can be returned to a satellite for sterilization in a safe orbit above GEO.
Then even with the Earth kept 100% safe (no realistic risk) through sterilization, it’s important to engage with the public and get widespread agreement that the chosen method is effective and would keep Earth safe. The ESF study in 2012 recommended fora open to representatives from all countries globally because negative impacts could affect countries beyond the ones involved directly in the mission (Ammann et al., 2012, Mars Sample Return backward contamination – Strategic advice and requirements : 59)
It is still important for a sterilized sample return that the public and other countries need to be in agreement that the sterilization method selected is effective. So it would still fall under the recommendations to keep the public involved in risk governance. See above:
The 2002 study “Safe on Mars” proposed a simple test for astronaut safety with a single sample return mission, but with our current more complex understanding of Mars this is impossible
It's understandable that prospective Mars astronauts and colonists want a mission with a single "Yes / no" test to discover if it is safe to send humans to Mars. This may not be as easy as it seems at first. As we’ve seen it would need to answer “Yes / no” to questions such as
- “Are there Martian mirror life nanobacteria on Mars or in Jezero crater?” or
- "Are there any hazardous fungi in Jezero crater" or
- "Is Jezero crater safe for astronauts and is it safe for astronauts to return from Jezero crater to Earth?"..
At first sight Perseverance’s sample return might seem to be the best way to do this. Indeed, the study“Safe on Mars” by the National Research Council in 2002 proposed that NASA establishes zones of minimal biological risk on Mars.
The suggestion was to send a precursor mission to determine if organic carbon is present. If organics were found, at or above the life-detection threshold, the suggestion was to use a sample return to find out if there is life present (SSB, 2002, Safe on Mars: Precursor measurements necessary to support human operations on the Martian surface : chapter 5 : 38). If no life was found in the sample returned from a region, it would be declared a safe place for humans to land.
However, though Curiosity found organics in 2014 (JPL, 2014, How NASA Curiosity Instrument Made First Detection of Organic Matter on Mars), the organics discovered so far are believed to come from meteorite impacts, and the search for life is far more complex than just investigating the first organics found on Mars.
As we saw in the last section, the reason “Safe on Mars” recommended a sample return as the best way to determine safety was because in 2002 they lacked instruments that could do in situ biosignature searches in 2002, a reason that no longer applies today.
"Safe on Mars"was also written at a time when the possibility of present day life on the Mars surface was considered to be remote. At the time the surface was thought to have no possibilities for liquid water.
Based on what was known at the time, the authors of “Safe on Mars” were so certain that nothing of significance would be found, as the most likely outcome, that they suggested planning for a manned mission should go ahead, even before a sample can be returned. They expected the result of the first sample return to be favorable for a manned mission immediately after it
There has been some concern that if a sample return is required, the planning for the first human mission to Mars may be delayed until a sample can be obtained. The committee believes that, even should a sample be required because organic carbon has been found, a baseline plan for a mission to Mars and even hardware development may still proceed under the assumption that a sample return will not find anything significant enough with regard to Martian biology to invalidate the baseline mission plan.
(SSB, 2002, Safe on Mars: Precursor measurements necessary to support human operations on the Martian surface : chapter 5 : 41)
“Safe on Mars” is one of the main Mars related cites in the Decadal survey which in turn was the original motivation for the Mars sample return mission (Space Studies Board and National Research Council, 2012. Vision and voyages for planetary science in the decade 2013-2022 : 157)
It is the only cite in the Decadal survey summary for the sentence:
The elements of the Mars Sample Return campaign, beginning with the Mars Science Laboratory, will provide crucial data for landing significant mass, executing surface ascent and return to Earth, and identifying potential hazards and resources."
(Space Studies Board and National Research Council, 2012. Vision and voyages for planetary science in the decade 2013-2022 : 17)
One of the white papers for the next Decadal survey, submitted in 2020 made the same point as “Safe on Mars” that returned samples are critical for planetary protection protocols
Returned samples are also critical for developing appropriate planetary protection protocols for both Mars and Earth.
“Breaking the chain of contact” when leaving Mars is technically achievable for robotic missions, but it is not possible for a crewed mission and potential biological hazards must be determined before humans go to Mars.
(McSween et al., 2020. Why Mars Sample Return is a Mission Campaign of Compelling Importance to Planetary Science and Exploration.) White Paper for the Survey.)
Sample returns will indeed be needed at a later stage for planetary protection protocols, if we discover life, to learn more about its capabilities. However, sadly, Perseverance mission is not going to settle questions about the safety for Earth’s biosphere or astronauts of any present day life on Mars, even in Jezero crater.
Perseverance is:
- targeting a region of interest for past life rather than present day life
- is not equipped to search for biosignatures in situ, past or present.
- is not searching for extant life in Jezero crater, for instance, won’t sample the expected brine layers in the Jezero crater sand dunes (which could be habitats for more capable Martian life) or much of the dirt, or salts.
- won’t return much by way of dust, which might potentially carry viable dust from distant parts of Mars – it may have perhaps one sample of regolith, that may contain dust and whatever dust adheres to the outside of the sample tube walls
So, the current sample return strategy doesn’t have a strong focus on extant life, and is not going to return samples from the most likely places to search for present day life even in Jezero crater such as the dirt, salty brine microhabitats or the Martian dust.
Even if Perseverance finds a region with potential microhabitats for terrestrial life, such as one of the Recurring Slope Lineae, in Jezero crater, it is not sufficiently sterilized to approach any of them to sample them.
In short, the Perseverance mission with its criteria for selection of samples and its level of sterilization is not designed to give even a first idea of whether there might be extant life in Jezero crater. Even with the suggested bonus samples, Perseverance would only return extant life if it is surprisingly abundant in Jezero crater or in the dust.
With our understanding of Mars as we have it today, there is simply no way to use a sample return mission from Jezero crater to prove that it is safe to send astronauts there. We need a far more comprehensive investigation to establish that,
See also sections:
through to
NASA world leading on planetary protection until it closed down its office at early stages of Mars sample return planning against repeated advice of the Space Studies Board
In my comments under NEPA I suggested an alternative miniature life detection lab above GEO based on NASA's own Europa lander mission plan from 2016. Scientists assure us it is feasible to run such a lab on Europa.
NASA has been world leading in planetary protection in the forwards direction. In the photo in the Introduction, Carl Sagan is standing in front of a model of NASA's Viking lander, the cleanest space-craft ever sent to Mars. They made the spacecraft as clean as they could make them then heated them for 23 to 30 hours at 111.7°C in a nitrogen atmosphere with an oxygen content less than 2.5% and a moisture content less than 0.097% by weight (Puleo et al., 1976, Microbiological Profiles of the Viking Spacecraft). After this procedure the spacecraft had an estimated 30 cultivable spores per spacecraft (Barengoltz, 2005. A review of the approach of NASA projects to planetary protection compliance : 3), There would be between hundreds and thousands of viable organisms per spore. But these were reduced further by the journey to Mars and the harsh conditions on the martian surface and it likely had few microbes left. This remains the "gold standard" for planetary protection in the forwards direction. At the time of Viking we didn't have the technology to achieve 100% planetary protection in the forwards direction and the aim was to reduce the risk of forwards contamination per mission to less than 1 in 10,000 (Barengoltz, 2005, A Review of the Approach of NASA Projects to Planetary protection compliance : table 1 / page 3)
NASA's planetary protection officers wanted NASA to keep going with Viking level sterilization. It was feasible and the main issue was cost. It would have added $100 million per mission from this interview with NASA's second planetary protection officer Cassie Conley. As Cassie Conley, second planetary protection officer, put it:
But most of the data from Viking pointed to Mars as a lifeless place. If we’re going to look for life on Mars, it would be really kind of lame to bring Earth life and find that instead
…
Since then, NASA has still cleaned its Mars spacecraft to the same standards — “better than a surgical suite,” Dr. Conley said — but skipped the baking step. Sterilization would add perhaps $100 million to the price of a mission.(Chang, 2015, Mars Is Pretty Clean. Her Job at NASA Is to Keep It That Way)
COSPAR defines a Special Region on Mars as a region where terrestrial organisms are likely to propagate or with high potential for extant martian life (SSB, 2015. Review of the MEPAG report on Mars special regions : 6). An uncertain region is one that might be special, but we are not able to tell if it is nor not.
That saving of $100 million per mission had a downside as it meant we had to target sites for searches for past life and geology as the spacecraft weren't sterilized sufficiently to visit regions where present day life might be present (uncertain regions).
Thus NASA has avoided the special regions, which include anywhere with water ice within a meter of the surface. (An exception was the Phoenix Mars lander, which dug into ice in the polar region. The arm — but only the arm — was sterilized to Viking standards.)
(Chang, 2015, Mars Is Pretty Clean. Her Job at NASA Is to Keep It That Way)
We most likely haven't contaminated Mars yet with terrestrial life as our spacecraft are sent to regions on Mars unlikely to be suitable for terrestrial life to spread easily and though some spores have surely fallen off into the dirt they would be unlikely to find conditions that are suitable for them in the very harsh conditions on Mars and most would be quickly sterilized especially if exposed to sunlight and UV
She said that at launch, there were probably 20,000 to 40,000 heat-resistant bacterial spores on Curiosity, and perhaps 100 or 1,000 times more microbes not counted. Many of them would have since perished in the vacuum of space. Intense ultraviolet radiation on the martian surface would have killed many more — but not all, and some drop in the soil as Curiosity trundles by and performs its science work.
(Chang, 2015, Mars Is Pretty Clean. Her Job at NASA Is to Keep It That Way)
As we'll see at the end of this article we now have the technology to build spacecraft that can be heated to 300°C instead of the 111.7°C of Viking and this would let us achieve 100% sterile landers, marscopters, eventually rovers on Mars.
Much of the current planetary protection literature for a Mars sample return mission and for protection of Mars from terrestrial life in the forwards direction is written by either John Rummel or Cassie Conley who were employed full time by NASA for two decades from 1997 to 2017 (Voosen, 2017, With planetary protection office up for grabs, scientists rail against limits to Mars exploration).. to do research and advise NASA on planetary protection.
- Cassie Conley's research papers
- John Rummel's research papers
- John Rummel's CV with selected publications through to 2019 (Rummel, 2019, Curriculum Vitae)
They would not have made the mistakes I found in this PEIS.
At the last meeting of the planetary protection subcommittee in 2016, before it was closed down, the planetary protection experts on that committee recommended that NASA add an extra full time equivalent civil servant to the Planetary Protection Office because the workload was getting to be too much for a single planetary protection officer.
PPS … also heard a programmatics view of investment planning at STMD, noting again that there's a worrisome lack of investment in planetary protection technology. PPS fears it has been unable to get STMD to embrace planetary protection technologies that will enable NASA to go to water worlds or Mars, or to send humans to Mars.
PPS issued a recommendation that NASA assign an additional full-time equivalent (FTE) civil servant to the PPO, reasoning that a one person office at Headquarters is inadequate given the growing number of missions that have planetary protection consequences. PPS notes that there is increasing load on PPO that is not accompanied by concomitant funding
(Peterson, 2016, National Advisory Council Science Committee Meeting Report, July 25-27, 2016 : 9)
Instead of adding an extra civil servant or increasing funding for planetary protection, NASA closed down the planetary protection office.
This is against the repeated advice of the Space Studies Board that NASA needs such a panel. This was the conclusion of the 2009 Sample Return study written three years after NASA closed the interagency panel:
It is clear to the committee that NASA will need to obtain continuing interagency advice (e.g. from the Centers for Disease Control and Prevention and relevant biosecurity agencies and organizations) on planetary protection policies and compliance, similar to the functional role played by the Interagency Committee on Back Contamination (ICBC) during the Apollo program. At present, important advice is provided via the interagency representation on NASA's internal Planetary Protection Subcommittee. However PPS currently reports via the Science Committee of the NASA Advisory Council, an arrangement that arguably leads to conflicts of interest with science and mission efforts.
(SSB, 2009, Assessment of planetary protection requirements for Mars sample return missions : 67 - 68)
NASA instead went on to closed down the planetary protection subcommittee too.
The Space Studies Board repeated this advice most recently in 2018 saying that it is needed for peer review (amongst other reasons):
Finding: The development and implementation of planetary protection policy at NASA has benefited in the past from a formally constituted independent advisory process and body. As this report is written, both the advisory body and process are in a state of suspension.
Recommendation 3.6: NASA should reestablish an independent and appropriate advisory body and process to help guide formulation and implementation of planetary protection adequate to serve the best interests of the public, the NASA program, and the variety of new entrants that may become active
The roles of the advisory body include the following:
- Serve as a sounding board and source of input to assist in development of planetary protection requirements for new missions and U.S. input to the deliberations of COSPAR’s Panel on Planetary Protection;
- Provide advice on opportunities, needs, and priorities for investments in planetary protection research and technology development; and
- Act as a peer review forum to facilitate the effectiveness of NASA’s planetary protection activities.
(SSB, 2018, Review and Assessment of Planetary Protection … : 61 - 62)
This wasn’t done.
But we can change this.
Need to avoid a trade-off between planetary protection and NASA's obligations to minimize costs
NASA sadly has a perverse incentive to skimp on planetary protection for funding reasons. They always have too many mission proposals they want to do and too little funding for it, especially for the robotic programs. That $100 million for every $1 billion million mission for planetary protection could be used for something else. They are also required to minimize costs of the missions. It is understandable NASA on a tight budget trims costs.
As an example a fully featured Mars Receiving Facility as envisioned in 2009 (Mattingly, 2010, Mission Concept Study, Planetary Science Decadal Survey, MSR Orbiter Mission (Including Mars Returned Sample Handling)), Mars Receiving would cost over $471 million in 2015 dollars.
By saying that environmental effects would not be significant and health impacts negligible they can replace it with a much lower cost " BSL-4 equivalent facility".
NASA’s concept for the SRF is to build a facility that can be characterized as a BSL-4 equivalent facility. The facility would nominally incorporate the designs and procedures of a BSL-4 facility (which has significant security requirements) and possibly, as yet undefined, additional cleanliness and protective measures
(NASA, 2023. Mars Sample Return final PEIS : 3-13)
Of course this wouldn't be their reason for making that decision. But it is an influence on planners that they know that if they can show that minimal planetary protection is needed it is far easier to get the mission within budget and on time.
If we decide we need a fully telerobotic facility to contain lab leaks and adding the capability to keep out 100% of ultramicrobacteria, this would likely add to the roughly half billion dollar cost tag from 2009.
The proposed alternative of a miniature satellite similar to the Europa lander but only launched to above GEO could cost significantly less than that half billion dollars and at a high technological readiness level.
Though in this case the planetary protection costs are reasonably low, the cost of one extra satellite which will cost less to put into orbit by the 2030s, we are likely to have occasions where we need to pay substantially more for a more thorough planetary protection of Earth. So we need a way to overcome the incentive to reduce costs for planetary protection to find funds for other things.
An independent body to safeguard Earth and Mars: avoiding perverse incentives to reduce safety by ensuring planetary protection costs don't come from NASA's budget
This may need to be addressed at a higher level such as in Congress if it is agreed that it is important to preserve the biosafety of Earth and to protect Mars with adequate staff to liaise with commercial space as they move towards their first missions to Mars.
NASA currently runs at planetary protection costs ranging from 0.4% to 1.1% (Bearden et al., 2017, Cost of Planetary Protection Implementation : 3)
The Viking mission cost an extra 10% in order to protect Mars from terrestrial contamination in the forwards direction (SSB, 2018, Review and Assessment of Planetary Protection … : 35)
Rummel et al reviewing the literature find a cost equivalent to the cost of one large science instrument. They cite a 2006 review which found the recurring cost for Viking-level-sterilization of the rovers at about 14% above non-sterilized-rover cost.
Claims that planetary protection implementations are “cost-prohibitive” don't usually address the question of planetary protection costs in detail but simply state that costs “can substantially increase” if one goes to a Special Region (cf. Fairén and Schulze-Makuch, 2013).
The oft-repeated estimate of “10% of total mission cost” for the planetary protection project for Viking included the cost of ground-based infrastructure, while the two most expensive science instruments on Viking were 20% of the total mission cost (Bionetics Corporation, 1988); thus, planetary protection total costs were equivalent to that of one large science instrument. A thorough Jet Propulsion Laboratory study (Gavit et al.,2006) put the recurring costs of sending Viking-level-sterilized Mars Exploration Rovers to Mars at about 14% above non-sterilized-rover costs, including reserves. When one considers that each Mars Exploration Rover cost less than a new Boeing 747, this is not a huge amount of money—certainly not enough to prohibit doing a proper job of searching for life on Mars by ensuring we don't instead find the Earth life we brought with us.
(Rummel et al., 2017, Four Fallacies and an Oversight: Searching for Martian Life)
The 14% figure is based on the science of 17 years now and may well be reduced since then. This book's proposal for 100% sterile rovers based on modern technology would need a new assessment of costs.
Rummel et al say the reason NASA never made funding available for Viking level sterilization for modern rovers is that the decadal surveys have never concluded that it is a high priority to access the special regions where we might find present day martian life and need Viking level sterilization. As a result, there isn't the incentive needed to develop the technology.
Issues that are of most concern to mission planners include cost and technical capability—yet the Viking Program was successful in sending nearly sterile life-detection missions to Mars when that had never been done before. The development of technical capability certainly incurs costs— and estimates of the costs associated with performing full-system dry heat microbial reduction of a Mars lander have remained essentially constant at the equivalent of one large science instrument, as we cited previously (Rummel and Conley, 2017).
On the one hand, it is not possible to say that this is inexpensive, given that recent large instruments (e.g., mass spectrometers) sent to Mars have cost over 100 million dollars. On the other hand, the total cost of recent Mars missions has run into several billions of dollars, so the fractional cost of planetary protection measures could be under 10%.
Again, it becomes a question of priorities—if one really wanted to study a Mars Special Region, this would be an acceptable cost. However, because access to Special Regions was not identified as a high priority in Planetary Science Decadal Surveys, the investments necessary to reestablish those capabilities (linking back to the Viking experience) have not been made.
(Rummel et al., 2018. Inadvertently Finding Earth Contamination on Mars Should Not Be a Priority for Anyone)
As we see, it is of exceptionally high priority for astrobiology to access these regions, though this was not picked up in the decadal surveys for some reason.
This book suggests we shift to more rather than less ambition in both directions and suggests we can achieve this by integrating more closely with human missions by establishing the aim to find out whether it is safe or not for humans to land on Mars as a top priority.
If we do this, funding still remains an important issue and we need to look in detail at how to resolve these issues. The issue for NASA if they are required to make planetary protection a high budget priority internally is that given fixed funding caps, it reduces the number of instruments they can send to Mars even though the ability to access the special regions makes the missions more interesting for astrobiology.
So can we do this in a way that increases planetary protection without forcing NASA into a constant balancing act trading off science instruments with planetary protection? That is where this idea of independent funding for planetary protection can help.
It would have a ring fenced discretionary budget it could use to fund planetary protection for NASA missions that need it. The extra funding would be decided based on what is needed to protect the biospheres of Earth and Mars, rather than based on science priorities. By separating this into a different body then we avoid the trade-off. Of course it would be required to minimize cost but only if it can be done consistent with maintaining planetary protection and not as a trade off with the costs of robotic instruments or science capabilities.
This would let NASA design a mission based on the science return, and then the planetary protection for that mission added based on what is needed to protect the biospheres of Earth and Mars. There would likely be a cost cap for planetary protection, perhaps 15%? But if so, it is not permitted to compromise on planetary protection to achieve that cost cap. Instead, if adequate planetary protection is not possible within that cost cap the mission doesn't fly or it requires an extra procedure, perhaps a request to Congress for high profile missions. There would of course be a lot of coordination, but it would remove most issues to do with trade-offs between planetary protection and science return such as the ones NASA's first two planetary protection officers identified.
Funding planetary protection: a public good for private space companies
Private space also faces issues with the costs of planetary protection. Profitiliotis looked at that in his paper. He suggests we can make planetary protection more accessible with:
- Pre-competitive collaboration, private space and space agencies collaborate at an early stage to
produce data and tools for the benefit of the entire industrial sector. They can use their financial
and knowledge resources to overcome common problems
(Profitiliotis, 2021, Planetary Protection Issues of Private Endeavours in Research, Exploration, and Human Access to Space: an Environmental Economics Approach to Forward Contamination : 5) - It often costs more to fix problems than to prevent them, so preventing contamination may reduce overall costs compared to permitting it and meeting the costs for any effects
In that paper he looks at forwards contamination. The costs for backwards contamination could be billions of dollars even for a new mold from Mars that spoils cheese or bread as we will see below in:
The example scenarios of very large-scale harm we introduced here such as a global fungal pandemic or mirror life mass extinction, though they may be very unlikely, could cost far more even than the COVID pandemic. These are worst case costs for the global economy which can be prevented by taking adequate precautions in advance. The economic case is similar to the economic case for smoke alarms and fire extinguishers.
More serious impacts could have higher economic costs. The costs of the COVID pandemic on the US alone is estimated in trillions of dollars (Hlavka et al., 2023, Opinion: ‘Unprecedented by most measures’: Calculating the astonishing economic costs of COVID) (Walmsley et al., 2022. Macroeconomic consequences of the COVID-19 pandemic).
The worst case scenarios we introduced here such as a global fungal pandemic or mirror life mass extinction could cost far more even than the COVID pandemic. These are worst case costs for the global economy which can be prevented by taking adequate precautions in advance. The economic case is similar to the economic case for smoke alarms and fire extinguishers.
For forwards contamination he lists four costs (Profitiliotis, 2021, Planetary Protection Issues of Private Endeavours in Research, Exploration, and Human Access to Space: an Environmental Economics Approach to Forward Contamination : 3)
- Impact on astrobiological research value of Mars
- Endangering or disrupting possible pristine niches of martian ecology
- Inserting hardy terrestrial organisms into in situ resources on Mars such as underground aquifers
that may lead to biofouling
[Some terrestrial microbes may also turn them into calcite, cement making them inaccessible altogether] - Introduced microbes could interfere with attempts at terraforming or more generally ecopoesis (e.g. trying to restore the ecology of ancient Mars) by ecological competition with deliberately introduced beneficial microbes.
He suggests a private / public investment expenditures scheme to finance forwards planetary protection.
As mentioned in a previous section, the prevention of environmental degradation is usually more cost-efficient than its remediation, though this remains to be quantitatively assessed for the case of forward contamination. Following the paradigm of funding environmental pollution abatement and control, this flagship project could be financed via a public/private expenditures scheme as well.
To gauge the contribution of public funding to this mix, a valuation of the total economic value of the aforementioned extraterrestrial non-excludable goods may be utilized.
…
However, although private space actors already handle some of the planetary protection costs as internal to their core business, an internalization of the negative externalities through a pre-competitive collaborative funding of a shared forward contamination mitigation analogue program may assist in a reduction of the internalized costs, by splitting them across a global industry consortium. Setting aside functional business strategies, such as corporate social responsibility and sustainability, that may use such a flagship project for positive branding purposes, it seems that NewSpace companies could be significantly benefitted from such an initiative even from a strictly financial point of view.
(Profitiliotis, 2021, Planetary Protection Issues of Private Endeavours in Research, Exploration, and Human Access to Space: an Environmental Economics Approach to Forward Contamination : 6)
The same approach could work in the backwards direction too. It would be far more effective to use public and private finance together to achieve a common goal.
How an independent body can enable fast bandwidth and sterile landers for a rapid astrobiological survey
The same independent body could also have extra ring-fenced discretionary funding for:
- telerobotics above GEO for the proposal to return samples to a miniature telerobotic lab above GEO, and pre-sterilization of samples returned to Earth,
- adapting the Venus HOTTECH to achieve 100% forward planetary protection
- high bandwidth optical communications with Mars to enable a rapid astrobiological survey of the
planet
- this is a priority for NASA but one they find hard to fund and vital for a rapid astrobiological survey of Mars
- with high bandwidth their HiRISE mission would return images equivalent to 18 such satellites orbiting Mars.
HiRISE is the remarkable high resolution optical telescope orbiting Mars which achieves sub-meter resolution photographs of the surface. However, it is limited in the number of photographs it can take of Mars mainly because of the bandwidth. It can only send back 6 Megabytes per second.
At MRO's maximum data rate of 6 megabits per second (Mbps) (the highest of any Mars mission), it takes nearly 7.5 hours to empty its on-board recorder and 1.5 hours to transfer a single image back to Earth that the onboard High Resolution Imaging Science Experiment (HiRISE) camera has taken. In contrast, with an optical communications solution at 100 Mbps, the recorder could be emptied in 26 minutes, and an image could be transferred to the Earth in less than 5 minutes.
(NASA, 2014, Benefits of Optical Communications)
So at 100 Mbps with optical communications, it will be able to return a maximum of nearly 17 images (100/6) in the time it currently takes to return one image.
If this can be coupled with a 12 meter ground telescope at night, it could transmit far more, never less than double the current maximum rate, even when Mars is at is furthest from Earth, reading off those numbers from this figure:
(NASA, n.d., Deep Space Optical Communications (DSOC))
NASA plan to send a technology demo on the Psyche mission when it launches to visit the asteroid of the same name - a huge asteroid made entirely of metal. The Deep Space Optical Communicator will be tested as far as its flyby of Mars and possibly for longer in an extended mission (NASA, n.d., Deep Space Optical Communications (DSOC))Psyche will launch in 2023.
This is a closely related and connected issue to planetary protection. It's far easier for NASA to fund a new flagship mission with new instruments and with the possibility of new results from Mars than an optical communications satellite that will benefit all its missions, lead to huge science return per mission for rovers and satellites, but not introduce any new capabilities itself.
NASA needs to evaluate missions according to their scientific merit which makes it hard to approve missions that have low scientific interest themselves but hugely boost the scientific value of all other missions.
Given the importance of bandwidth for a rapid astrobiological survey of Mars it might be appropriate to combine together the costs of telerobotics / bandwidth and planetary protection in a separate ring fenced budget to make sure this gets appropriate attention.
At present the bandwidth is so low that even with a round trip latency which at its best is less than 7 minutes, Perseverance and Curiosity’s teams can only download data from the previous day which they then use to plan operations for the next day which they then send up in commands to Mars (Buckner, 2022, NASA Mars Perseverance: A Sol in the Life of a Rover). It would be as easy to control a rover on Pluto with round trip latency of 11 hours as a rover on Mars! Though it's not as easy to quantify this for rovers as with HiRISE, it's clear that our rovers on Mars could do far more science per sol with high bandwidth.
This would also help with NASA relations with the new agency, as they would see it as boosting the capabilities of all their missions on Mars.
It could be funded separately in much the same way the ISS is funded separately but with a much lower budget than the ISS as telerobotic missions are far lower cost than missions with human astronauts. Similar also to the Center for Near Earth Object Studies which is funded for another type of planetary defence, defence against asteroids.
As an example in the 2021 budget request, NASA has:
- $2,660 million for Planetary Science including Commercial Lunar Payload Services, the VIPER lunar rover, Discovery and New Frontiers missions (Lucy, Psyche, Dragonfly), and Planetary Defense (DART).
- $12,949 million for human exploration and operations
- $1,578 million for exploration technology - to support human exploration.
- $3,549 million for Safety, Security and Mission Services and Construction and Environmental Remediation
- Funds critical Agency-wide capabilities, workforce, and facilities across the country that are
essential to NASA meeting agency priorities, including the
Moon-to-Mars campaign. - Ensures NASA infrastructure and assets are safe, secure, environmentally sound, appropriately sized, and efficiently operated.
(NASA, 2021, NASA FY 2021 BUDGET REQUEST)
There isn't any separate budget for planetary protection.
It is especially important to make sure NASA's planetary protection is adequately funded now that we have:
- Technology to develop 100% sterile rovers and landers in the forward direction (as a result of NASA’s HOTTECH program for a Venus surface lander).
- Technology to send dozens, even hundreds of micro-rovers to numerous locations on Mars with a single mission
- which has the potential to rapidly expand our understanding of Mars
- but if done without adequate protection could also lead to rapid and widespread contamination in the forwards direction of the most interesting locations for native life on Mars
To involve private space in the rapid astrobiological survey of Mars, one approach might be using prizes like the Ansari X-prize that lead to the first privately funded human space-flight in history, with a prize of $10 million and $30 million invested in the winning entry vehicle designed by Rutan and the basis for Virgin Galactica. (NASA, 2004, Ansari X-Prize : A Brief History and Background). There could be similar prizes for the first lander, or later rover, designed for Mars to resist heating to 300C for an hour (or whatever is needed) with particular requirements for what it needs to be able to do on Mars, and then later to demonstrate it on Mars, to make miniaturized landers to spread over Mars and so on.
Detailed scenarios of mirror life and a novel fungal genus to motivate the need for great care in biosafety planning to protect Earth
I developed two illustrative scenarios with the aim to motivate space agencies to take their planetary protection responsibilities seriously. These are not intended as high risk examples, but rather to show that just as for the house fire example, we have to look at worst case scenarios even if they appear to be very low risk.
They are intended as examples to illustrate the scientific literature on the potential for large scale effects.
The need for the PEIS to cover such examples is included under NEPA in section § 1502.21 (c) and (d)
(c) If the information relevant to reasonably foreseeable significant adverse impacts cannot be obtained because the overall costs of obtaining it are unreasonable or the means to obtain it are not known, the agency shall include within the environmental impact statement:
(1) A statement that such information is incomplete or unavailable;
(2) A statement of the relevance of the incomplete or unavailable information to evaluating reasonably foreseeable significant adverse impacts on the human environment;
(3) A summary of existing credible scientific evidence that is relevant to evaluating the reasonably foreseeable significant adverse impacts on the human environment; and
(4) The agency's evaluation of such impacts based upon theoretical approaches or research methods generally accepted in the scientific community.
(d) For the purposes of this section, “reasonably foreseeable” includes impacts that have catastrophic consequences, even if their probability of occurrence is low, provided that the analysis of the impacts is supported by credible scientific evidence, is not based on pure conjecture, and is within the rule of reason.
My two scenarios are, for environmental effects: See below:
And for human health and wildlife:
Mirror life as a potential astrobiological surprise like the unexpected carbon dioxide geysers for geology
NASA has made many extraordinary geological discoveries on Mars, such as the CO2 geysers.
Text on graphic: Artist’s impression of CO2 geysers on Mars, one of many geological surprises.
Mars could have astrobiological surprises too.
What if Mars has independently evolved mirror life?
Artist’s impression by Ron Miller of the martial CO2 geysers that form in spring in the polar regions (Miller, 2006, PIA08660: Sand-Laden Jets (Artist's Concept), JPL).
We have the potential to make similar discoveries in astrobiology, as surprising as life based on mirror chemistry or even more exotic.
When a molecule can occur in two mirror forms, like your hands, it’s called chiral - the word chiral is derived from the Greek word χειρ (kheir) for hand. Terrestrial life is homochiral, which means that nearly all of its asymmetrical (chiral) molecules occur in only one of its two mirror forms. Also terrestrial life for the most part can’t use any mirror organics it finds and just ignores them.
So how did terrestrial life get its preference for one symmetry? Depending on when and how this happened,it might be possible for independently originated life on Mars to have the opposite symmetry.
Life could have originated before the first prebiotic chemical evolution towards life, either on Earth or in space or at various stages during the evolution of life (Sallembien et al., 2022. Possible chemical and physical scenarios towards biological homochirality : fig 19).
One of the more popular ideas is that the Earth was seeded by organics from space which already had a slight excess of one symmetry because of the effect of circularly polarized light on the organics that form in a molecular cloud that collapsed to form our solar system. We see this type of light in some parts of the Orion nebula for instance (Fukue et al., 2010. Extended High Circular Polarization in the Orion Massive Star Forming Region: Implications for the Origin of Homochirality in the Solar System) (Sallembien et al., 2022. Possible chemical and physical scenarios towards biological homochirality : 3.2 (a))
If that is how it happened, or if life on Earth was seeded from Mars and vice versa or if both had a common origin we would expect life on Mars to have the same symmetry as terrestrial life.
However there are numerous other proposed explanations. Some physical, prebiotic. Some due to life processes themselves.
In many of the theories the selection of ordinary over mirror organics is random. See (Sallembien et al., 2022. Possible chemical and physical scenarios towards biological homochirality : figure 1) which covers these topics in detail. This book covers the topic in more detail below:
For the purposes of this illustrative example, we'll take one popular theory, the theory of punctuated chirality. According to this theory, there’s a 50 - 50 chance that independently evolved life on Mars uses mirror organics. The suggestion is that early on as life was just starting to evolve, there were patches of chemicals that worked together with each other in chiral networks which expand converting a non chiral substrate into chiral organics,.Then, where two chiral networks of opposite chirality meet there are ways for them to slowly convert each other to the opposite chirality.
There would be many such patches, some with the same chirality as terrestrial life and some with mirror organics. According to this theory, these patches would expand and flip each other back and forth in chirality on an environmental scale, with the chirality reset multiple times in Early Earth even if it didn’t go extinct (Gleiser et all, 2008, Punctuated chirality : 6) until one of them got established as the basis for the evolution of life.
If so, depending on how the flips went on Mars, life could easily have evolved from the mirror chemicals of the ones Earth life evolved from.
“Our analysis predicts that other planetary platforms in this solar system and elsewhere could have developed an opposite chiral bias.”
(Gleiser et all, 2008, Punctuated chirality : 7)
Text on graphic: By the theory of punctuated chirality, in a large sample of glucose from many planets containing life or prebiotic chemistry, roughly half would be D-glucose and half L-glucose
D-Glucose which terrestrial life can use
L-Glucose used as an artificial sweetener
Similarly for all organics that can occur in two mirror forms
Graphic for L-glucose and D-glucose by reflecting the graphic horizontally. Grey: Carbon, Red: oxygen, white: Hydrogen.
Scenario of a mirror life algae from Mars pre-adapted to normal organics brought to Mars by infall from space
So, what if we find mirror life on Mars? It needn't be mirror life, it could be something even more exotic including possibilities we haven’t considered yet, as much of a surprise for astrobiologists as the CO2 geysers were for planetary geologists. It might be as remarkable as the CO2 geysers, but not safe to mix with our biosphere. For our illustrative scenario we use mirror life.
So that leads to this new scenario:
- Scenario of independently evolved mirror life, evolved from the mirror chemicals on Mars. If Mars has independently evolved life, this could be mirror life (Gleiser et all, 2008, Punctuated chirality : 7) and if we return mirror life from Mars the worst case could be quite dire, mirror organics spreading through our soils, of no nutritional value to our microbes, grass, etc. Meanwhile in this scenario, mirror life can probably use terrestrial organics, accustomed to infall of both types of organics from space on Mars. This leads to a worst case scenario already considered by synthetic biologists as a motivation to be ultra cautious to contain synthetic life (Bohannon, 2010, Mirror-image cells could transform science-or kill us all)
- In this scenario, the mirror life is likely to be adapted to use normal organics because of
infall from space, but few terrestrial microbes have the enzymes to convert mirror to normal
organics to metabolize it
Most terrestrial life depends on L-amino acids and can't use D-amino acids. However, the strain Raineyella antarctica LZ-22T is able to use both L and D forms of the amino acids Met (methionine) and Leu (Leucine, and is only able to use the D forms and not the L forms of His (Histidine), and Val (Valine) (Zipse, 2009, Amino acids and their abbreviations). See: (Pikuta et al.., 2016. Raineyella antarctica gen. nov., sp. nov., a psychrotolerant, d-amino-acid-utilizing anaerobe isolated from two geographic locations of the Southern Hemisphere) summarized in (Aliashkevich et al.,., 2018. New insights into the mechanisms and biological roles of D-amino acids in complex eco-systems : D-Amino Acids as a Sole Carbon and Nitrogen Source) - We won’t want to find out what happens if mirror life spreads through our planet converting normal to mirror organics that only mirror life can use.
- Terrestrial life might well still eat mirror organics, like artificial sweeteners, but starve
in the midst of plenty because it couldn’t do anything with the flipped starches or
proteins, and many fats would also be inaccessible.
For some of the differences see: (Dinan et al., 2007, An adventure in stereochemistry: Alice in mirror image land) answers in: (Yee, 2002, Through the Looking Glass and What Alice Ate There). It’s quite tricky telling the difference between a molecule that’s chiral (different from its mirror image) and achiral (same as its mirror image) molecule - if you can just turn it over to get the other pattern it’s achiral, but if you have to reflect it in 3D as in a mirror it’s chiral. A carbon atom with four distinct groups attached to it is normally going to be chiral and life can usually only use one of the two forms - see (Kennepohl et al., n.d., Chiral Molecules)
Text on graphic: Normal life, Mirror life, DNA, amino acids, sugars, fats, everything flipped. Most normal life can’t eat mirror organics. Martian mirror life might be able to eat normal organics.
Background image from (NOAA, n.d. Can we clean up, stop, or end harmful algal blooms?). DNA spiral from (Pusey, 2012. DNA groove animation based on PDB 1DNH
To be more specific, in this scenario we might have a mirror life analogue of chroococcidiopsis on Mars.
- Chroococcidiopsis, a highly resilient blue-green algae does well in Mars simulation chambers (Li et al., 2022, Protection and Damage Repair Mechanisms Contributed To the Survival of Chroococcidiopsis sp. Exposed To a Mars-Like Near Space Environment)
- and is able to repair extensive DNA damage including double strand breaks, taking about 12 hours to repair the damage, with no significant loss of viability even after revival after the equivalent of 20 million years of surface ionizing radiation on Mars (Mosca et al., 2021, Role of DNA repair pathways in the recovery of a dried, radioresistant cyanobacterium exposed to high-LET radiation: implications for the habitability of Mars : 9 - 10)
- It can find all its nutritional requirements in seawater, or rocks such as basalt, so long as
it also has access to water and a source of energy such as sunlight or hydrogen. It's able to flourish in complete darkness, even 10 to 750 meters below the Atlantic sea bed using an alternative metabolic pathway to
metabolize hydrogen and with nothing else except gabbro to live on (Li et al., 2019, Recycling and metabolic
flexibility dictate life in the lower oceanic crust : 3). It is able to live there in small
populations by using carbon and nitrogen found in small quantities in the basalt - though it can
also use photosynthesis to add to the carbon when it has access to sunlight and can do nitrogen
fixation to add to the nitrogen when it has access to nitrogen.
- A single monolayer of grains 0.15 mm thick reduces the UV nearly 100-fold (Godin et al., 2023, Absorption and Scattering of UV and Visible Light Through Simulated martian Regoliths and Rock Samples : 5) and (from abstract) iron oxides in the Mars dirt preferentially block out damaging UV and lets through frequencies of light used by photosynthesis - a microbe protected by even a single grain of JSC Mars 1 simulant has orders of magnitude less UV damage. In that 2023 paper, Golin et al. concluded: "From the perspective of the radiation environment, a radioresistant microorganism that uses photosynthesis as an energy source could survive in the subsurface of Mars." (Godin et al., 2023, Absorption and Scattering of UV and Visible Light Through Simulated martian Regoliths and Rock Samples : 10).
- Many minerals found on Mars similarly let light through and block damaging UV, like calcite, and kieserite a magnesium sulphate mineral abundant on Mars (Mosca et al, 2022, The spectral variability of kieserite (MgSO4·H2O) with temperature and grain size and its application to the martian surface). The best of all in this respect is the clay mineral cheto bentonite. These all block out damaging UV much more than they block the light needed for photosynthesis (Godin et al., 2023, Absorption and Scattering of UV and Visible Light Through Simulated martian Regoliths and Rock Samples : 9))
- This blue-green algae could live anywhere on Mars with basalt and access to water.
- Chroococcidiopsis is an ancient polyextremophile with numerous alternative metabolic pathways that strains of this genus can use, including nitrogen fixation, methanotrophy, sulfate reduction, nitrate reduction, etc. (KEGG, n.d., Metabolic pathways - Chroococcidiopsis thermalis). An alien microbe from Mars based on mirror life might be just as versatile, accumulating many pathways over billions of years of evolution on Mars. That would help it to radiate through our biosphere even if we return only one species from Mars.
The suggestion is not an exact copy of Chroococcidiopsis flipped in a mirror. It's an independently evolved mirror life analogue of our blue-green algae on Mars with similar nutritional requirements and environmental preferences, but made up of mirror organics.
Some synthetic biologists have embarked on a likely decades-long research project to make mirror life in the laboratory by slowly flipping components of a terrestrial microbe bit by bit.
Synthetic biologists will ensure mirror life is not able to survive in the wild at any point in this slow step by step transformation from normal to mirror life. In Kasting and Church’s worst-case scenario for release of mirror life from a terrestrial lab, mirror life retains the edge over normal life in this evolutionary race and eventually there is little left except mirror organics and life that can use it. They suggest humans could go extinct in this worst case scenario that they need to avoid, at least in natural ecosystems
“—both Kasting and Church think mirror predators would evolve, but whatever life existed on Earth by that point wouldn’t include us.”
(Bohannon, 2010, Mirror-image cells could transform science-or kill us all)
It would be a similar scenario if we return naturally evolved mirror life from another planet.
I don’t think we’d go extinct in that scenario. We might be able to engineer terrestrial microbes with the ability to eat mirror organics and limit the mirror life, and in the worst case we could “paraterraform Earth” - we could cover jungles, grassland, mountains, islands, coral reefs, eventually continents, ice sheets, and large areas of the oceans with larger and larger shelters like Biosphere 2 (Biosphere 2, n.d., Under the Glass Systems) and with the technology of the future, manage them internally to keep out most of the mirror life. But if we do have mirror life on Mars, we as a civilization would surely decide not to do the experiment of returning it to Earth.
Potential for mirror life and ordinary life on Mars to co-exist, either as both independently evolved on Mars or the ordinary life imported from Earth
The mirror life could also co-exist with normal life on Mars which could have evolved originally in regions separated by physical barriers, for instance after the volcanic eruption on the flanks of Arsia Mons 210 million years ago, which likely lead to 100 cubic kilometers of subsurface melt in three lakes, which would have stayed melted for centuries to millennia insulated by surface ice (Scanlon, 2014, A habitable environment on Martin volcano?)
With the punctuated chirality model, Gleiser et al. see it as an unlikely possibility that the very early stage of prebiotic chemistry they are looking at could start in hydrothermal vents, but if it could, different vents would vary in chirality. However, there is too much mixing from the circulation of the oceans for them to stay as separate networks indefinitely, as they gradually evolve to life (Gleiser et all, 2008, Punctuated chirality : 6).
Mars would have many more opportunities for separate networks early on with less water in its ocean, and separated impact basins. Perhaps that might suggest it could have co-existing normal and mirror life even early on.
This is a combination of two scenarios in Cockell's paper on trajectories of habitability on Mars, the idea of a new biologically isolated habitat that forms by impact into permafrost::
A scenario for the formation of an uninhabited habitat on Mars by an impact into permafrost, which remains hydrologically isolated at macroscopic and microscopic scales even on a planet that has a hypothetically colonized subsurface
(Cockell, 2012, Trajectories of martian habitability : fig. 6)
Which is combined with the idea of life becoming extinct and reoriginating on Mars - in this case it only becomes locally extinct and is still present elsewhere on Mars.
There are other trajectories of greater complexity that can be envisaged. Examples include an inhabited Mars on which life becomes extinct and then reoriginates (or is transferred from Earth) at some later time.
(Cockell, 2012, Trajectories of martian habitability : fig. 6)
If the habitat forms as a result of a volcanic eruption then it could remain habitable, but isolated beneath a surface layer of ice, perhaps for some considerable period of time. Also if life never developed photosynthesis or this happened before photosynthesis, uninhabited habitats on the surface could become biologically isolated more easily. Similarly if it never developed resilient spores or other propagules able to spread in the dust storms any life would likely be very localized.
Whether this is possible would depend on how long it takes for a new form of life to evolve, and how much time the habitats persisted for at various times in martian history and the capabilities of any life that does evolve.
Potential for mirror life nanobes on Mars that might be able to use nutrients more efficiently and avoid grazing by being ultra small
It might be possible for very early life to co-exist with terrestrial life if its cells are very small, based on mirror life or some other biology. That was the inspiration behind the hypothesis of a shadow biosphere of nanobes (Cleland, 2019, The Quest for a Universal Theory of Life: Searching for Life as we don't know it : 213 - 214) which could co-exist with modern life. Earth doesn’t seem to have a shadow biosphere (yet) but small cells have an advantage in an environment with low nutrient concentrations (Ghuneim et al., 2018, Nano-sized and filterable bacteria and archaea: biodiversity and function)
- can escape grazing by larger grazing amoebas which don’t notice them
- have a larger surface area to volume ratio, so can use nutrients better in nutrient poor conditions, and so take up nutrients more efficiently.
If ribocells are possible they could be very tiny (SSB, 1999, Size limits of very small microorganisms: proceedings of a workshop : 117). These are cells that have only RNA, no DNA, no proteins, and the enzymes are made up of fragments of RNA. It is a field of very active research at present (Kun, 2021. Maintenance of Genetic Information in the First Ribocell). If at some point in our exploration of Mars, we find very small early life cells, even of ordinary life, it doesn't seem that we can assume in advance that they couldn't survive alongside more evolved terrestrial life. We would need to evaluate them carefully to see if they can be safely returned to Earth unsterilized.
In the case of mirror life, these co-existing ribocells could transform ordinary to mirror organics in the same way as larger microbes, but with the additional advantage that they avoid protozoan grazing and can colonize nutrient poor conditions more efficiently.
Martian life could also have more efficient photosynthesis. See below:
So in the worst case scenario here, which we do need to look at for planetary protection, martian mirror life could have multiple advantages permitting it to spread faster on Earth.
We do have a potential analogue of a transformation of Earth's biosphere by novel microbes in the past. We don't actually know that photosynthetic life originated on Earth. If it got to Earth from Mars, a minority view, it could have been the largest ever transformation of Earth's biosphere the result of potentially just one viable microbe transferred from Mars to Earth. Life could have originated on Mars and it might by chance have evolved photosynthesis first there, perhaps because conditions on Mars for some reason favoured the evolutionary pathways leading to photosynthesis.
Great Oxygenation Event used to show a single species could in principle cause large scale biosphere changes and mass extinction as for the worst case mirror life scenarios
To make this mirror life scenario more concrete, let's use the microbe Chroococcidiopsis. It may be partially responsible for the oxygenation of our atmosphere. One minority view explains the unusual ionizing radiation resistance of Chroococcidiopsis as a natural adaptation of martian organisms (Pavlov et al., 2006. Was Earth ever infected by martian biota? Clues from radioresistant bacteria).
This is weak evidence since the ionizing radiation resistance of chroococcidiopsis could be a byproduct of the repair mechanisms that chroococcidiopsis uses for UV resistance and desiccation resistance. Cyanobacteria originated in the Precambrian era. It could have developed these mechanisms back then, when, with no oxygen in the atmosphere, there was no ozone layer to shield out UV radiation (Casero et al., 2020. Response of endolithic Chroococcidiopsis strains from the poly extreme Atacama Desert to light radiation) (Raman et al., 2006, Analysis of proteins involved in the production of BMAs in two Cyanobacteria Synechocystis PCC 6803 and Anabaena cylindrica)
However, the early martian atmosphere was rich in oxygen (Lanza et al, 2016, Oxidation of manganese in an ancient aquifer, Kimberley formation, Gale crater, Mars) before Earth and though much of that may well be due to ionizing radiation from solar storms splitting the water it’s not impossible that it had photosynthetic life.as well.
Benner and others have argued that Mars is a likely origin for life (Shilling, 2015, Are We martians After All?) (Benner, 2015. The case for a martian origin for Earth life) (Ranjan, 2017, The UV Environment for Prebiotic Chemistry: Connecting Origin-of-Life Scenarios to Planetary Environments : 193)))
Their reasoning is that:
- it had a larger area of dry land for the wet / dry cycles that may have been important for concentrating organics for early life
- dry conditions are needed for RNA which can’t form in water (water is corrosive for RNA).
- it had boron, and phosphates important for likely early life processes,
- boron combines with the oxygen in carbohydrates to prevent formation of tars
It had molybdates which catalyses reactions that can convert the boron stabilized carbohydrates into ribose, needed for the formation of RNA and early life precursors
The curious thing about the Great Oxygenation Event is how long it took. Maybe it takes 2 billion years to evolve the capability. But there is evidence even the earliest bacteria had started on the path to evolution of Photosystemr II. So it could have evolved far faster on other planets. If so, could it have evolved first on Mars, by chance, and then got here after that? (Oliver et al., 2021, Time-resolved comparative molecular evolution of oxygenic photosynthesis), press release (Dunning, 2021, Photosynthesis could be as old as life itself)
Whatever the cause of the GOE (most would say late evolution of photosynthesis on Earth), it's an example to show the potential for a single species to transform the biosphere of a planet. See:
It would be a major challenge for photosynthetic life to get from Mars to Earth as Charles Cockell showed. The biggest challenge is that the preferred habitat of photosynthetic life is just below the surface or on the surface of rocks where it can access light. In an experiment he did with chroococcidiopsis attached to the aeroshell of a rocket during re-entry of Earth's atmosphere, he found that none of it survived. Not even the organics survived.
However, he didn't rule out the possibility that it could happen on very rare occasions. He concluded
… Thus, the planetary exchange of photosynthesis might not be impossible, but quite specific physical situations and/or evolutionary innovations are required to create conditions where a photosynthetic organism happens to be buried deep within a rock during ejection to survive atmospheric transit.
(Cockell, 2008, The Interplanetary Exchange of Photosynthesis : 13 section: Survival of the Filters)
So was this an extinction event? The Great Oxygenation Event might have forced rapid evolution rather than extinction. Early anaerobes may have retreated to anaerobic habitats as obligate anaerobes, which we still have today (Lane, 2015. The vital question: energy, evolution, and the origins of complex life : 49)
However, there is some evidence suggesting extinctions. There is evidence of exceptionally large sulfur reducing bacteria from this time, 20 to 265 µm in size, which also occasionally occur in short chains of cells. This may be part of a diverse ecosystem that predated the GOE (Czaja et al., 2016. Sulfur-oxidizing bacteria prior to the Great Oxidation Event from the 2.52 Ga Gamohaan Formation of South Africa),. See also Czaja interviewed for University of Cincinnati by Melanie Schefft,
“And this discovery is helping us reveal a diversity of life and ecosystems that existed just prior to the Great Oxidation Event, a time of major atmospheric evolution.”
(Schefft, 2016, Life before oxygen)
For more about these potential microfossils, see (Lepot, 2020, Signatures of early microbial life from the Archean (4 to 2.5 Ga) eon : 6.1.3. Large spherical microfossils). This paper also looks at other evidence suggesting a diverse microbial ecosystem before the Great Oxygenation event. It could have been based on anoxgenic photosynthesis, photosynthesis that doesn't produce oxygen. In our current oxygen rich atmosphere, any iron II ( Fe2+) is rapidly oxidised to insoluble iron III ( Fe3+). But before the Great Oxygenation Event most of the iron would be dissolved in the oceans as iron II. Life could metabolize that to Iron II using sunlight, and that may be the explanation of the distinctive banded iron formations from early Earth. The same process happens today in iron-rich meromitic lakes (lakes with layers of water that do not mix).
The equation is (Camacho et al., 2017, Photoferrotrophy: Remains of an Ancient Photosynthesis in Modern Environments.):
4 Fe2+ + HCO3- + 10 H2O + light → 4 Fe (OH)3 + CH2O + 7 H+
The earlier banded iron formations probably formed through this process, while later on life resembling the modern blue-green algae would have produced oxygen using sunlight and the oxygen then lead to the formation of the iron deposits.
The earliest banded iron formations were found in Greenland as a result of ice melting from global warming, the Isua greenstone formations from 3.8 billion years ago, which may have formed in this way through anoxygenic photosynthesis. Later banded iron formation likely formed due to oxygen produced by oxygenic photosynthesis by blue-green algae (Wang et al., 2023, Archean to early Paleoproterozoic iron formations document a transition in iron oxidation mechanism). This paper also looks at other evidence suggesting a diverse microbial ecosystem before the Great Oxygenation event.
Text on graphic: These banded iron formations from Isua in Greenland are 3.8 billion years old and may have been deposited before modern photosynthesis
Dark layers rich in iron oxides likely deposited by ancient lifeThe ecosystem that formed these rocks could have experienced a slow mass extinction during the Great Oxygenation event, used to an atmosphere without oxygen.
Whether the earlier ecosystem went extinct or survived, the Great Oxygenation Event is an example of a large-scale change that could in principle be caused by a single species from Mars.
Though the likelihood is surely low, mirror life could in principle cause large-scale changes and mass extinction according to calculations by synthetic biologists.
Background image from: (St John, 2010, Graphitic BIF (meta-BIF) (Eoarchean, 3.8 Ga; Isua Supracrustal Belt, southwestern Greenland)
[See also copyright policy for the Field Museum)
If such an ecosystem existed, most traces of it are gone now. Traces from such an early diverse ecosystem might have survived in smaller numbers in anaerobic habitats, but it seems not impossible that the GOE had major impacts on a prior diverse ecosystem.
There are many other confirmed mass extinctions in the fossil record. In many cases the cause is not fully known or debated leaving it at least hypothetically possible that microbial transfer from Mars could be part of the explanation.
Whether or not this ever happened in the past, this worked example of the Great Oxygenation Event shows how in the worst case scenario, independently evolved life from another planet could lead to large scale transformations of the chemistry of Earth’s atmosphere or oceans, climate and ecosystems. Humans with modern technology would surely survive a gradual transformation of our atmosphere and oceans by a microbe with novel capabilities no terrestrial life has yet explored - but it could make the planet significantly less habitable in the short term for humans and other species.
I shared a concrete example of this possibility for a single species of microbe based on alien biology to transform our biosphere with the mirror life scenario above, in this case gradually changing our normal organics to mirror organics, leading either to a situation with nearly all organics in their mirror form or an equal quantity of mirror and normal organics depending on whether and how the terrestrial life is able to evolve to metabolize mirror life - or possibly the other way round that terrestrial life wins.
It seems a biologically possible scenario that a mirror microbe from a planet resembling Mars somewhere in our universe could transform a biosphere resembling Earth's to a mirror biosphere depending on its capabilities. It's hard to estimate the timescale as it depends on its capabilities and how rapidly it radiates to new ecosystems.
The European Science Foundation report briefly touches on the possibility that life transferred from Mars to Earth in the past could have had evolutionary consequences for Earth. It concludes that we can't rule out the possibility of this happening again.:
This is not to say that these exercises in logic [the Mars meteorite argument] can provide any guarantee of safety. Indeed, the implications of Mileikowsky et al. (2000) are that it is possible that the natural interchange of materials between Mars and Earth, perpetuated as a result of large impact events across the history of the solar system, could have also involved the infrequent exchange of live microorganisms from time to time.
This could have resulted in either colonization of one planet by life from the other, and the potential for biospheric exchange that may have had evolutionary consequences. Joshua Lederberg, himself a pioneer in the consideration of the consequences of an interplanetary exchange of organisms, noted the limitations of mankind's ability to deal with the problem of a sample returned from Mars and its possible consequences for Earth life (Lederberg, 1999):
… On the one hand, how could microbes from Mars be pathogenic for hosts on Earth when so many subtle adaptations are needed for any new organ- isms to come into a host and cause disease? On the other hand, microorganisms make little besides proteins and carbohydrates, and the human or other mammalian immune systems typically respond to peptides or carbohydrates produced by invading pathogens. Thus, although the hypothetical parasite from Mars is not adapted to live in a host from Earth, our immune systems are not equipped to cope with totally alien parasites: a conceptual impasse” (Lederberg, 1999).
With those thoughts in mind, it may seem that the risk posed by returning a dangerous biological entity (e.g. a virus-type, microorganism, etc.) is quite low. Nevertheless, it still cannot be guaranteed to be impossible.
(Ammann et al., 2012, Mars Sample Return backward contamination–Strategic advice and requirements : 12)
For more about what Lederberg said in his two papers on the topic of the potential effects of returning a completely alien biology see the Supplementary information section below under:
The NRC study makes a similar point in Chapter 5, that we can't know if any life from Mars could have caused large-scale effects in the past.
Section title: Potential for Large Scale Effects
Despite suggestions to the contrary, it is simply not possible, on the basis of current knowledge, to determine whether viable Martian life forms have already been delivered to Earth. Certainly in the modern era, there is no evidence for large-scale or other negative effects that are attributable to the frequent deliveries to Earth of essentially unaltered Martian rocks. However the possibility that such effects occurred in the distant past cannot be discounted.
…
Thus it is not appropriate to argue that the existence of martian meteorites on Earth negate the need to treat as potentially hazardous any samples returned from Mars by robotic spacecraft.
A prudent planetary protection policy must assume a biological hazard exists from Mars sample return and that every precaution should be taken to ensure the complete isolation of any deliberately returned samples, until it can be determined that no hazard exists.
The committee concludes that the risk [of large scale effects] appears to be low but not demonstrably zero.
(SSB, 2009, Assessment of planetary protection requirements for Mars sample return missions : 48)
The 2002 "Safe on Mars" NRC study makes a similar argument from past mass extinctions. It uses it to argue that we need to make sure any location we send astronauts to on Mars is lifeless, as if there is Martian life there we can't prove that it is safe to return it to Earth:
Finally, it must be acknowledged that there have been biological upheavals through the course of paleontological history here on Earth, including millions of species extinctions, with few explanations of cause. It is impossible to determine the cause of these upheavals with absolute certainty, be they physical (meteorite impact) or biological (life introduced onto Earth). While the possibility that life was introduced on Earth from elsewhere is unlikely, the uncertainty makes it impossible to prove that meteorites from another planet have never had an effect on Earth 's biosphere (NRC, 1997).
These considerations, and the consideration that a goal of future human missions will be to search for life-forms in Martian oases, if those oases exist, negate the argument that there is no biohazard threat from Mars based on the existence of sterile Martian meteorites.
(SSB, 2002, Safe on Mars: Precursor Measurements Necessary to Support Human Operations on the Martian Surface : 37)
This issue is likely to be raised many times by the lay and scientific public, it already has been raised in the NEPA comments. I raised it of course based on the National Academy of Sciences study, but it was also raised independently the Infectious Disease/Clinical Microbiologist William Agger (USNews, n.d., Dr. William A. Agger MD) who raises this issue based on his own reasoning:
William Agger: I am opposed to bringing rocks from Mars to Earth. While some believe the risk can be kept to a minimum, the potential effects of accidentally introducing invasive Martian bacteria to the Earth’s biosphere is too great. For instance, it has been scientifically shown that the Earth has been periodically showered through geologic time with Martian rocks, and yet “We are still here”. This is “anthropomorphic” reasoning. Yes, we are still here, but the Earth’s geologic periods are replete with massive extinctions which changed the course of biologic history. Is it not possible that some, if not all, of the massive extinction events may have been related to panspermic meteoric showers?
Even if only one was the result of a Martian microbe finally surviving through interplanetary space to cause a massive change in the web of life on Earth, that alone should disqualify NASA from adding this risk to the ongoing human-caused mass extinction.
... In short, it’s a scientific gamble with a small upside against a catastrophic downside for the living people on the Earth and to the only biosphere they will survive on. Finally, I am not opposed to looking for life on Mars. If found, it should be left on Mars and studied there.
(Agger, 2022, Comment Submitted by William Agger)
I hope the mirror life illustrative scenario that we discussed above helps decision makers and mission planners to appreciate that these concerns are indeed reasonable and grounded in science.:
This scenario of mirror life from Mars isn't needed to motivate planetary protection from an academic point of view. However it is a way to make this issue more compelling, of a low likelihood of potential mass extinction from a sample returned from Mar, for space agencies tasked with ensuring they have a comprehensive biosafety plan to protect Earth's biosphere. It can also help clarify ideas and is a useful tool to let us look closely at some of the issues that might arise. A worked out scenario like this can make potential issues easier to study than with the abstract idea of large-scale harm without a particular concrete scenario.
Scenario of a novel fungal genus, analogue of Aspergillus fumigatus, able to infect humans with a high fatality rate
I also developed a plausible scenario for a serious human pathogen to motivate taking great care to protect human health from large-scale impacts. It is also a good example to motivate taking great care with the Mars samples to protect birds (wild or domestic).
This particular example of a species from a novel fungal genus similar to Aspergillus is new, but I cover fungal diseases from Mars in the preprint that I was working on before the first round of public comments. The version I attached to the second round of public comments is here:
I'd like to emphasize, especially for any members of the public reading this that get easily scared, this is a worst case scenario like a house fire. Not intending to suggest this is a likely scenario, that we return a fungus like this from Mars.
So here is the scenario:
- a fungal disease, similar to Aspergillus, which is not adapted to humans and has severe effects on 200,000 immunocompromised people a year, fatality rate 30% to 95% (Brown et al., 2015, Hidden killers: human fungal infections : table 1), and in immunocompetent people, it causes allergic reactions in 2.5% of all asthma cases. That’s typically 48 million cases of ABPA (allergic bronchopulmonary aspergillosis), which in 400,000 cases have progressed to the serious chronic disease of chronic pulmonary aspergillosis (Denning et al., 2013, Global burden of allergic bronchopulmonary aspergillosis with asthma and its complication chronic pulmonary aspergillosis in adults) for details of the disease: (AAAAI, n.d., Allergic Bronchopulmonary Aspergillosis (ABPA) Symptoms, Diagnosis, Treatment & Management)
- Aspergillus fumigatus (the main Aspergillus species to harm humans) has no known specific adaptations to infect any other organism, human or otherwise (McCormick et al., 2010, Aspergillus fumigatus: contours of an opportunistic human pathogen). Instead it infects us because of many adaptations to extreme environments (Paulussen et al., 2017, Ecology of aspergillosis: insights into the pathogenic potency of Aspergillus fumigatus and some other Aspergillus species)
- with characteristics that may well be shared by martian fungi (Paulussen et al., 2017, Ecology of aspergillosis: insights into the pathogenic potency of Aspergillus fumigatus and some other Aspergillus species : table 1, table 2 and section: Biophysical capabilities and ecophysiology of pathogenic Aspergillus species).
Paulussen et al put it like this:
Collectively, the aspergilli are remarkable fungi. … there are numerous aspects of Aspergillus cell biology and ecology (including their metabolic dexterity when adapting to nutritional and biophysical challenges) which contribute to their status as, arguably, the most potent opportunistic fungal pathogens of mammalian hosts.
Aspergillus species are able to utilize a wide range of substrates, highly efficient at acquiring such resources, and can store considerable quantities of nutrients within the cell; all traits which contribute to their energy‐generating capacity and competitive ability
…
Species of Aspergillus are also among the most stress‐tolerant microbes thus far characterized in relation to, for example, low water activity, osmotic stress, resistance to extreme temperatures, longevity, chaotropicity, hydrophobicity and oxidative stress
(Paulussen et al., 2017, Ecology of aspergillosis: insights into the pathogenic potency of Aspergillus fumigatus and some other Aspergillus species : section: Biophysical capabilities and ecophysiology of pathogenic Aspergillus species).
Those are all capabilities that would be very useful on Mars in the high stress, highly oxidising, low nutrient conditions there, as well as other adaptations of Aspergillus mentioned in that paper such as ability to respond to rapid hydration and ability to cope with low oxygen conditions in the lungs.
Microbes returned from Mars could be even more stress tolerant than Aspergillus species, to all those conditions.
It may help to give a summary of some of the key points.
From the section: “Biophysical capabilities and ecophysiology of pathogenic Aspergillus species” (Paulussen et al., 2017, Ecology of aspergillosis: insights into the pathogenic potency of Aspergillus fumigatus and some other Aspergillus species). aspergillus species are able to be pathogenic in humans because of many adaptations in extreme environments, as a result of which they:
- Can recover and rehydrate rapidly from desiccation – this lets inhaled desiccated spores recover rapidly to colonize the respiratory tract
- Have large amounts of melanin which can protect the cell membranes from breakdown (lysis) by the immune system
- Produce enzymes that break down proteins (proteases)
- Produce branching filaments (hyphae) which mechanically penetrate tissues and can also break up into fragments that can spread rapidly through immunocompromised patients
- All these activities require a lot of energy and Aspergillus species are able to colonize a wide range of media, highly efficient at taking up nutrients and converting it to energy
- Amongst the most stress tolerant of species, able to resist extreme high temperatures, highly tolerant of low temperatures, also oxidative stress (including hydrogen peroxide), UV, carbon and nitrogen starvation, and ionizing radiation (Paulussen et al., 2017, Ecology of aspergillosis: insights into the pathogenic potency of Aspergillus fumigatus and some other Aspergillus species : table 2)
- Able to protect themselves from chaotropic agents like urea and ethanol, by stabilizing their proteins, producing more proteins, more energy, and modifying their membrane composition and increase production of enzymes that remove reactive oxygen species. [there are many chaotropic agents on Mars such as the perchlorates]
- Able to tolerate low oxygen levels in the lungs (as low as 1% partial pressure in inflamed tissue) and some strains can function without oxygen (Paulussen et al., 2017, Ecology of aspergillosis: insights into the pathogenic potency of Aspergillus fumigatus and some other Aspergillus species : table 2)
- Many specific adaptations to stress including ability to produce EPS for biofilms, accumulate large amounts of melanin in the cell walls, and protein stabilization mechanisms, and they can synthesize solutes like glycerol for protection from the environment around them. The glycerol can protect against freeze thawing (Paulussen et al., 2017, Ecology of aspergillosis: insights into the pathogenic potency of Aspergillus fumigatus and some other Aspergillus species : table 2)
- Naturally resistant to antifungals generally - some species of aspergillus can pump antifungals out of the cell (using efflux pump transport proteins) and also secrete plastic like substances (polymeric substances) to reduce contact with the antifungals, the melanin in the cell walls can bind to antifungals and they can use heat shock responses to reduce the entry of antifungals
(Paulussen et al., 2017, Ecology of aspergillosis: insights into the pathogenic potency of Aspergillus fumigatus and some other Aspergillus species : : table 1)
These are all capabilities that could be shared by a new genus of fungi from Mars. Indeed, aspergillus niger, one of the occasional opportunistic pathogens of humans, also happens to have high resistance to UV for a terrestrial microbe, higher than some microbes from Mars analogue environments. It was tested in a Mars analogue experiment in a high altitude stratospheric balloon because of potential for forward contamination of a human mission to Mars (it’s found on the outside of spacecraft and is one of the species of microbe in the ISS) and was still viable after 5 hours of UV at levels similar to full daylight on the Martian surface (Cortesão et al., 2021. MARSBOx: fungal and bacterial endurance from a balloon-flown analog mission in the stratosphere : table 3) and ionizing radiation between a third and a quarter of Mars surface conditions (Cortesão et al., 2021. MARSBOx: fungal and bacterial endurance from a balloon-flown analog mission in the stratosphere)
Aspergillus is capable of micro-evolution as it spreads through the body after it infects a host (Ballard et al., 2018. In-host microevolution of Aspergillus fumigatus: A phenotypic and genotypic analysis). The same could be true of any putative Martian fungus after it infects a human host.
This suggests a scenario where, just like Aspergillus fumigatus, a martian fungus could be pre-adapted for spreading rapidly through a human host because of adaptations to extreme stress conditions on Mars including low oxygen, UV, ionizing radiation, rapid changes in temperature and humidity, and likely evolutionary adaptations for rapid recovery and rehydration after desiccation.
Some of these adaptations to extreme stress would be directly useful, and others would assist in infecting humans because of adaptations such as biopolymers to protect the fungus from stress would also protect the fungus from stressors in the human host such as immune system responses.
Also Aspergillus can form aerosols very easily - spores floating in the wind - and a fungus on Mars might do the same, to propagate in the very thin atmosphere on Mars.
Any martian organism that propagates in the dust storms needs to form spores or propagules that can be picked up by gentle winds. Either low density, with a high surface area to volume ratio or very small. Typical wind speeds are from 3 meters per second at night in dust storms to 10 meters per second or higher in daytime during dust storms (Viúdez-Moreira et al., 2020, Effects of a large dust storm in the near-surface atmosphere as measured by InSight in Elysium Planitia, Mars : figure 5). That's up to 36 kilometers per hour to 22 miles per hour.
Dust devils on Mars often travel horizontally as fast as 20 to 30 m/sec and can reach over 50 m / sec (Stanzel et al., 2010, Dust devil speeds, directions of motion and general characteristics observed by the Mars Express High Resolution Stereo Camera : table 1, page 28). That's over 180 km / hour, or over 111 miles per hour)
However with the atmosphere at typically less than a hundredth of the density of Earth's atmosphere, since kinetic energy is mass * velocity squared, winds need to be ten times faster on Mars to have the same effect. Seasonal pressures in Perseverance crater vary from about 0.6% to around 0.8% of terrestrial pressures (Sánchez-Lavega et al., 2023, Mars 2020 Perseverance Rover Studies of the Martian Atmosphere Over Jezero From Pressure Measurements : fig 4) which means that a wind on Mars is roughly equivalent to a wind on Earth of an eleventh of the speed at the highest pressure measured so far of 0.8%.
So, even 180 km per hour is equivalent to 16 km / hour of terrestrial winds, or about Beaufort scale 3, which is not quite enough to lift dust and loose paper (Lewis, n.d., Beaufort scale). The 36 kilometers per hour daytime dust storm wind speed is equivalent to 3.3 kim / hour terrestrial winds, or Beaufort scale 2, fast enough for leaves to rustle (Lewis, n.d., Beaufort scale). Andy Weir's dust storm at the start of his book "The Martian" was poetic license (Weir, 2014, The Martian | Andy Weir | Talks at Google)
Even with the low gravity the only reason that Mars has dust storms is because the particles of dust are very small, up to a few microns in diameter. So we could expect any fungus that does spread in martian winds to produce light easily spread spores like Aspergillus fumigatus.
This shows how aspergillus fumigatus infects the lungs. First the wild fungus produces spores. The spores settle in the lungs. They then penetrate the lungs with hyphae (tendrils) which extract organics. It protects itself with chemical barriers and produces branching networks of these tendrils which then break up and spread through the body.
Text on graphic. The way a fungus infects us is very straightforward - insert tendrils (hyphae) to extract organics. Even an alien fungus with no adaptations to terrestrial life could do this. Uses chemicals to attack tissues. Also protects itself with chemical barriers.
Graphic from: (Creative Biolabs, n.d., Aspergillosis)
We already have a Mars analogue black yeast Exophilia Jeanselmei which can grow in Mars simulation chambers and is classified as a risk group 2 organism in humans.
The main thing that may be different from a Mars organism is that Aspergillus fumigatus needs oxygen, but it can manage with levels of oxygen as low as 1% at sites of infection where the tissues take up oxygen to fight back, while the related Aspergillus terreus can manage in lungs without any oxygen using nitrogen reduction (Paulussen et al, 2017, Ecology of aspergillosis: insights into the pathogenic potency of Aspergillus fumigatus and some other Aspergillus species : table 2). Many fungi don't need oxygen, also Mars has some possibility of oxygen in colder martian brines (Stamenković et al., 2018, O2 solubility in martian near-surface environments and implications for aerobic life). Also the fungal component of the alpine lichen Pleopsidium chlorophanum found on rocks from California to Antarctica can be grown without oxygen in Mars simulation chambers, where it seems to get all the oxygen it needs from the algal component of the lichen (de Vera et al., 2014, Adaptation of an Antarctic lichen to martian niche conditions can occur within 34 days). Perhaps a martian fungus could also grow in a biofilm that includes photosynthetic life. So we might well return fungi from Mars that are able to use oxygen as well as to grow without oxygen.
This is a close up of the details of how a spore (conida) lands on a surface and starts to grow into it.
Though the details are complex the basic way a fungus like aspergillus infect us is rather straightforward - it uses tendrils to extract organics and it protects itself with chemical barriers. It also uses chemicals such as acids or alkalis to damage the host tissue to help with breaking up the organics and to protect itself. These are things that even a fungus analogue from an alien unrelated biology might do.
Graphics from (Creative Biolabs, n.d., Aspergillosis)
The novel fungal genus scenario also doubles as an illustrative worst case for poultry and wild bird
This also doubles as a worst case for wildlife as Aspergillus is a serious pathogen of some wild birds (Tell, 2005, Aspergillosis in mammals and birds: impact on veterinary medicine) especially wildfowl, raptors and gulls (Cornell Wildlife Health Lab, n.d., Aspergillosis).
It also doubles as a worst case scenario for domesticated birds because Aspergillus often causes serious problems with turkeys and other poultry in farms. There is no treatment available for commercial flocks though pet birds can be treated (Lorenzoni, 2023, Aspergillosis in Poultry)
- Aspergillus is especially serious in birds, because they don’t have an epiglottis to prevent particles getting into the lower respiratory tract, or a diaphragm for a cough reflex, and have fewer cilia to move foreign bodies out of the lungs : (Tell, 2005, Aspergillosis in mammals and birds: impact on veterinary medicine)
Our body doesn't have broad spectrum antifungals like it has for bacteria and archaea because they have neutral cell walls like our own cells - so it uses patterns specific to each genus and might either ignore a novel genus from Mars or else overreact with an allergic reaction - and there may be no antifungals to treat it
The details here are technical and this book goes into them in more depth in the Supplementary information under:
Many ways samples from Mars can be harmless
We need to look at worst cases, as for a house fire, for a biosafety plan. But that doesn't mean they are likely. Let's look at what else can happen
- We most likely don’t return life at all
- if we return life, it most likely wouldn’t be able to spread outside the laboratory,
- we don't know that Mars has ultramicrobacteria and if it does the ultramicrobacteria might be harmless to Earth
- a BSL-4 might well be able to contain harmful life,
- if Mars has harmful microbes still, most species may well be harmless or beneficial.
Looking particularly at the Perseverance mission, the first samples returned from Mars:
- even if Mars has a few harmful microbial species, the first microbes from Mars might well be harmless or even beneficial
- we may well return no life given that this is a mission that isn’t able to detect present day life on Mars, is sent to a region of Mars thought to be less habitable than other regions, and given the harsh conditions on Mars.
In all the sample return reports to date, the experts have been of the view that the chance of returning a microbe capable of large-scale harm to human health or the environment like these examples appears to be low.
However, as for house fires,we need to prepare for worst case scenarios, and not just best case scenarios. We also need to set a good precedent for future samples returned from Mars.
There's another possibility that is rarely considered.
Illustrative "minor" nuisance: Rapidly moldy bread or cheese from cold competent Martian life with potential billions of dollars global liability and impact on public support for space exploration
Most of the discussion rightly is on the worst case scenarios. But we should touch briefly on relatively "minor" scenarios as though minor compared to the worst cases, they could still have major impacts, for instance economic impacts.
As an example of a minor effect, Martian life would encounter brines at temperatures down to -70°C and below. Even if it can retain water through to warmer conditions in biofilms, there'd be a strong evolutionary pressure to use the water at the coldest possible temperatures to have water available for more of the year or day. So, one rather plausible possibility is that we find martian life that is adapted to grow at well below the usual lower limit of growth for life of around -20°C, and to grow relatively quickly at low temperatures too, perhaps with a novel type of biology.
Cheese is especially vulnerable to spoilage by molds at low temperatures because of its high moisture content. Some molds can spoil cheese below 0°C especially in the Penicillium genus.
Many Penicillium species have the ability to grow at low temperatures, even below 0 °C, and so are a prime cause of spoilage of refrigerated foodstuffs. A few species also have the ability to grow under reduced oxygen tensions, and so are the major cause of spoilage of packaged, refrigerated goods. Penicillia can be seen on goods in nearly any refrigerator. Perhaps the most vulnerable food of all is cheese, with a generally high water activity, and generally dependent on refrigeration and packaging for microbial stability.
(Pitt, 2006, Food Spoilage Microorganisms [snippet shared in "cheese spoilage"] )
Bread is another example of a food especially vulnerable to spoilage by mold. One of the common causes of bread spoilage is aspergillus niger (Rahman et all, 2022, A Comprehensive Review on Bio-Preservation of Bread: An Approach to Adopt Wholesome Strategies : table 2). We saw above that it is a reasonable analogue of a martian organism.
See above:
Species of Penicillium, Fusarium and Cladosporium are capable of growing at temperatures below 5°C, and some even just below freezing (Bullerman et al., 1984, Formation and Control of Mycotoxins in Food)
The global cheese economy is over $100 billion and many cheeses rely on molds for flavor, but molds are also a major nuisance (Backes, 2021, The science behind moldy cheeses: An interview with Dr. Maike Montanhini). The global bread industry is over $200 billion annually (MMR, 2023, Bread Market: Global Industry Analysis and Forecast (2023-2029) ). A significant percentage of bread produced is sometimes lost to molds, for instance 34.7% in Germany in 2015 and 10% in Brazil in 2021 (Rahman et al., 2022. A Comprehensive Review on Bio-Preservation of Bread: An Approach to Adopt Wholesome Strategies)
Crops are also directly vulnerable to molds and these also have economic effects on milk and meat due to animals fed with moldy cereals. There are many molds that have economic impacts. This graphic summarizes the economic and health impacts of aflatoxins produced by species of Aspergillus, especially Aspergillus fumigatus.
From: (Sayed et al., 2022. An overview on the major mycotoxins in food products: characteristics, toxicity, and analysis : figure 1)
Aspergillus flavus is a common opportunistic pathogen of drought-stressed oilseed crops including maize, peanut, cottonseed and tree nuts, and of insects and animals including humans (Klich, 2007, Aspergillus flavus: the major producer of aflatoxin). As with Aspergillus fumigatus the mode of attack is straightforward, it just finds entry into the host and extracts organics that it finds useful for metabolism, protecting itself from hazards in the host much as it does from hazards in the soil (Paullussen et al., 2016, Ecology of aspergillosis: insights into the pathogenic potency of Aspergillus fumigatus and some other Aspergillus species), It produces the highly carcinogenic aflatoxins (Klich, 2007, Aspergillus flavus: the major producer of aflatoxin)
One estimate is that in the warmer conditions due to climate change, aflatoxins produced by the fungi Aspergillus flavus and A. parasiticus will cost the US corn industry alone US$52.1 million to US$1.68 billion a year (Mitchell et al., 2016, Potential economic losses to the USA corn industry from aflatoxin contamination)
Martian molds might cause more economic damage than this, We've seen that alien fungi don't need to be especially adapted to the conditions they face. They just need to be adapted to extreme conditions that coincidentally enable them to form protective barriers and to extend fibers into the food. Martian fungi might be able to grow in cold conditions well below zero by manufacturing perchlorates or hydrogen peroxide or other chaotropic agents and ice binding agents and extruding them to make the low temperatures habitable to them.
We covered details of how they might work above in the discussion of whether the brines Curiosity found could be habitable for native martian life. See:
So, in this scenario we return alien molds from Mars that can spoil food not only at just below 0°C but at very low temperatures. They may grow rapidly in fridges and even be able to grow and spoil food in freezers. Clark put it like this.
Clark looked briefly at the potential for litigation of space agencies if the returned samples led to issues with safety of frozen food. He draws attention to how much greater the global economy is than the budget of the space program. Based on examples like the economic effects of mad cow disease, he estimates that economic penalties could be 1 to 10 billion dollars, comparable to the total annual budgets of the space agencies that return them. This might lead to litigation of space agencies for the costs.
In our modern global and highly interdependent economy, relatively small perturbations can have large- scale effects. Although the overall economy is resilient to many such perturbations, their effects can be tallied in terms of economic loss. For example, one previously hypothesized scenario is that martian microorganisms might be super-competent in low-temperature environments.
Suppose there were to arise a concern for the safety of certain frozen foods, resulting in the destruction of foodstocks. Recent examples include the slaughtering of vast numbers of chickens in Hong Kong and beef cattle in Great Britain. Suppose the economic losses amounted to order of magnitude [1 to 10 billion] U.S. dollars (USD). Who would reimburse those whose livelihood had been affected -- the space agencies who participated in the project? Litigation would surely be considered since public reaction and allegations might be well beyond what can be readily proved. In this case, the economic penalties could be of the same order as the total annual budgets of the space agencies involved
(Clark, 2002, Martian meteorites do not eliminate the need for back contamination precautions on sample return missions : 1597)
He suggests that the effect on public support for space activities might be an even greater factor.
“Much worse, the effect on future programmatic support could result in a far greater negative impact”
(Clark, 2002, Martian meteorites do not eliminate the need for back contamination precautions on sample return missions : 1598)
It doesn't need to involve frozen food. It could spoil food in the refrigerator, or food that is transported at room temperature and normally thought to be safe to keep at room temperature. There are many accidental toxins that don't make food completely inedible but make it unpalatable so the food has to be discarded and can't be sold. We cover some of these in the Supplementary information under:
- An alien biochemistry could have many beneficial bioactive compounds but also many accidental toxins
in the section
In the worst cases here, the resulting economic damage globally from food spoilage, or even localized to the USA, could be comparable to or exceed the budget of space programs. There might be demands for immediate compensation from food producers for the spoilt food. If one effect is that lower temperatures are needed to preserve food it might also lead to longer term expenses for redesign and replacement of cool rooms, fridges, freezers, refrigerated trucks (lorries) and boxcars (trains), and food storage facilities to protect our food against spoilage by martian organisms. The power consumption of freezers, fridges, etc., would also be higher if they have to keep food at lower temperatures than before.
It could also be a similar economic harm if the molds grow at more normal temperatures but with novel toxins that make food unpalatable and unsaleable even though still edible.
This could also be a major economic issue for the space program and the near term future of humans in space if NASA and ESA became liable under litigation. It may be a similar situation too for private space companies if they accidentally return life from Mars that spoils food globally leading to large scale litigation against them for damages.
Issues like this may seem "minor" if they don't lead to extinctions or to human or animal diseases, or even plant diseases. All that happens is preventable rotting of frozen plant products after they are harvested and of frozen meat from livestock, with use of colder temperatures in freezers fixing the issue. However these issues are far from minor if they are permanent changes to our biosphere globally.
This is another example that may help motivate space colonization enthusiasts to take such possibilities seriously.
We are less likely to have no effects from any microbes if we return most of the species from a complete martian biosphere
The experts I cite all seem of that view that large-scale effects are unlikely. Especially if Mars has related life, not exotic mirror life, we also get many invasive species that have no noticeable effects, just take part in the ecosystem alongside similar species. Many are also beneficial to the ecosystems they colonize. It is easily possible that if there is any life on a first mission that it has no effects or is beneficial.
However, if we continue and return all the life from a complete biosphere - if Mars does have a biosphere - that could be a different story. If Mars has dozens or thousands of species, even if all the species are microbial, it might be more surprising if none of them have any noticeable effects on Earth's biosphere or agriculture or significantly affect the millions of species that share our planet, either beneficial or harmful.
If Mars has a biosphere it evolved in conditions very different from Earth:
- The superoxidising surface conditions including the perchlorates, rare on Earth
- Ionizing radiation at levels life can only encounter on Earth in the upper atmosphere
- UV at higher levels than ever encountered on Earth, again except in the upper atmosphere
- Ultracold brines down to below -70°C with daily variations of temperature sometimes from below -70°C to above 15°C and back again in a single day (Earth gets temperatures that low but without the perchlorate brines, the record at 89.2°C is close to -90°C (Arizona State University, n.d., World's lowest temperature).
- Near vacuum atmospheric pressure
- Almost all the organics on the surface come from organic infall from comets, interplanetary dust and meteorites and so likely have roughly equal amounts of normal and mirror organics.
[ADDCITES to list]
Although some terrestrial extremophile microbes can likely cope with all those conditions in Mars simulation chambers, it's a different matter to evolve in such conditions for billions of years.
Any life on Mars may well have evolved to be significantly more resilient to those conditions than terrestrial life. Also, even if it is ordinary not mirror life, it is likely to have the isomerases to convert mirror organics to normal organics to metabolize the infall from space, a rare capability on Earth.
This suggests that any martian life is likely to have metabolic processes, enzymes, and capabilities that are unknown to terrestrial life even if it is related life.
So it seems unlikely that all, or even most life from Mars which is able to live on Earth is a "drop in" replacement for terrestrial life.
Martian life could easily still be largely beneficial or harmless. The entire domain of the archaea is largely beneficial or harmless to higher life. This is covered in the Supplementary information section under:
But If we do find life on Mars, related or unrelated, we simply don't have the science yet to say what the effect of mixing it with terrestrial life would be. So we will have difficult decisions as a species if we do find an indigenous martian biosphere. That became clear during the deliberations of the COSPAR sample safety assessment framework for samples from Mars. The method used for Apollo of exposing a wide variety of terrestrial species to lunar dust in experimental conditions is not sufficient to predict the effects of introduced life on our biosphere and can't be used any more.
During the Working Group’s deliberations, it became clear that a comprehensive assessment to predict the effects of introducing life in new environments or ecologies is difficult and practically impossible, even for terrestrial life and certainly more so for unknown extraterrestrial life.
(Kminek et al, 2022, COSPAR Sample Safety Assessment Framework (SSAF))
I cover this under:
It will be challenging even to rule out the potential for large-scale harm, especially from as yet undiscovered species in the biosphere.
The potential for large-scale benefit is also extraordinary if we find novel life on Mars, economically, in terms of knowledge of life, likely benefits to medicine and so on. It would be possible to get these benefits however by setting up an industry on Mars and never returning life to Earth. This is covered in the Supplementary information section under:
It is also possible that mixing the two biospheres has large-scale benefit too. It's possible that Martian life could enhance Earth, make it more habitable, help to fertilize deserts and so on. This is covered in the Supplementary information section under:
At present we have no idea what the effects would be of introducing martian life to Earth. If Mars does have a biosphere then it may be hard to predict what those effects would be even once we know what is there.
It would be hard to prove even that a single novel strain of chroococcidiopsis will cause no harm when returned to Earth. We cover this below in:
Any final decision might involve balancing benefits and risks, presumably with some way of compensating those that would be adversely affected in the case where we find returning martian life would be of great benefit to Earth's biosphere. There might be difficult decisions to make in that future if Mars does have a biosphere. But we don't have the information we need to make such decisions for all of humanity until we know what is there.
This book suggests that we need to go into this with our eyes open, find out what is there, and decide what to do based on knowledge rather than knowledge gaps.
This book will look into the knowledge gaps closer starting in the next section.
- Exploring the knowledge gaps in the Space Study Board's thought-provoking review of SR-SAG2
[and following sections]
After that this book will turn to how we can do a sufficiently thorough search for life on Mars to get the knowledge we need for future decisions about whether life on Mars could have
- major effects on public health or the environment
- or be a minor nuisance (potentially though with major economic effects),
- or indeed, be beneficial.
See below:
- We may only find past or present day life after a long search of many geologically identical rocks
[and following sections]
Exploring the knowledge gaps in the Space Study Board's thought-provoking review of SR-SAG2
Before we turn to the question of how to search for life on Mars, we need to look more closely at the knowledge gaps that we identified above in :
- Why NASA still looks for present day martian life: our only previous attempt to look got confused, the main focus has been on past habitability, and Mars could have barely habitable microbial oases in an almost lifeless planet
- Life in the uninhabitable - the Space Study Board's thought-provoking review of SR-SAG2 commissioned by NASA and ESA a year later
It is important to realize that SR-SAG2 specifically did NOT look at the potential for habitats for native evolved martian life that might have different capabilities from terrestrial life, reasoning that at present there are no regions on Mars characterized by the existence of native Martian life, and so focused only on regions where terrestrial life can spread.
COSPAR defines Special Regions as ‘‘a region within which terrestrial organisms are likely to replicate’’ and states that ‘‘any region which is interpreted to have a high potential for the existence of extant martian life forms is also defined as a Special Region’’ (COSPAR, 2011). At present there are no Special Regions defined by the existence of extant martian life, and this study concentrates only on the first aspect of the definition.
(Rummel et al., 2014. A new analysis of Mars “special regions”: findings of the second MEPAG Special Regions Science Analysis Group (SR-SAG2) : 888)
The Space Studies Board review was also focused on terrestrial contamination and didn’t look at the potential for more martian life with additional capabilities through evolution or novel biochemistry.
However though we don't know the capabilities of any native martian life, we do know that anything terrestrial life can do on Mars is something that potentially some form of martian life could do too. It is possible martian life evolved extra capabilities that terrestrial life never explored in very different martian conditions, or that are impossible for terrestrial life because they require a novel biochemistry (e.g. to be active at lower temperatures on Mars).
NASA and ESA's unusual step of commissioning and independent review of SR-SAG2 with the Space Studies Board, out of concerns its team was too closely aligned with NASA's Mars Program Office
First, why did NASA and ESA even do a review of SR-SAG2? It is very unusual for a major review study published in a peer reviewed journal to be questioned in this way and immediately in effect given a second review.
This was partly in response to concerns that the MEPAG team that wrote it were too closely aligned with NASA's Mars Program office. So NASA and ESA took the unusual step of commissioning an independent review of the SR-SAG2 study through the Space Studies Board and National Academy of Sciences.
NASA and ESA commissioned this review of SR-SAG2 partly out of concerns that MEPAG is not independent from NASA. When they found out that they had similar concerns they commissioned a combined review.
There were two reasons why both agencies took the seemingly unusual step of independently commissioning reviews of a review paper that was to be published in a peer-reviewed journal.
First, there is the perception in some circles that MEPAG is not independent and that its views are too closely aligned with NASA’s Mars Program Office. …
(SSB, 2015. Review of the MEPAG report on Mars special regions : xi – xii)
Here are the two studies:
- SR-SAG2 (Rummel et al., 2014. A new analysis of Mars “special regions”: findings of the second MEPAG Special Regions Science Analysis Group (SR-SAG2))
- The Space Studies Board review of it (SSB, 2015. Review of the MEPAG report on Mars special regions).
As already mentioned, the SSB review paints a picture of a Mars with significantly more potential for life than the inhospitable picture presented in SR-SAG2 which would indeed suggest almost no possibility of life in Jezero crater.
The SSB review identified several major knowledge gaps in SR-SAG2 relevant to Jezero crater, which couldn't be listed as findings, revisions or updates in their Appendix B
The main meat of the SSB review is in the recommendations for further research which identified many knowledge gaps about the potential for microhabitats, biofilms, caves that can't be seen from orbit and transport of viable life in the atmosphere and dust.
I commented on the draft PEIS saying it was a serious omission to cite SR-SAG2 and not cite the SSB review of it. NASA replied that the changes proposed were minor and only made a difference to fine differentiation of the special regions, if they exist, where terrestrial life might be likely to spread on Mars.
Robert Walker: It is a serious omission to cite Rummel et al 2014 and not cite the 2015 study commissioned by ESA and NASA that overturned or modified many of its findings.
This serious omission is especially important as it lead to an omission of any discussion of microhabitats in Jezero crater or of spores or propagules that could be brought there in the dust. (Board, 2015) found microhabitats and transport of microbes in dust storms were knowledge gaps that need to be addressed and weren't adequately covered by (Rummel et al, 2014)
(NASA, 2023. Mars Sample Return final PEIS : B-73 - B74)
NASA: the changes proposed to the 2014 paper by Rummel et al. (no change to 29 findings, modification of 13, combination of 2 findings, removal of 1 finding and introduction of a new finding regarding Humans to Mars missions) as a result of a joint review by the National Academies of Science, Engineering, and Medicine and the European Science Foundation are relevant for fine differentiation of special regions from other areas on Mars for forward planetary protection.
Even to the extent that habitability and special regions are considered together, Jezero Crater’s shallow subsurface has parameters for neither.
(NASA, 2023. Mars Sample Return final PEIS : B-73)
So NASA's team were aware of the 2015 review but thought the changes were too small for there to be a need to cite it. This is an understandable conclusion if one just goes to the list of "Findings, Revisions and Updates" in its appendix B.
Even Appendix B does have several relevance to habitability of Jezero crater if one examines the list more carefully. Here I am summarizing the four most relevant findings in (SSB, 2015. Review of the MEPAG report on Mars special regions : Appendix B)
- 2-4: possibility of liquid water associated with the sources of the methane plumes
- 3-1: possibility of slow growth of terrestrial life below below –18°C (255 K)
- 4-6/7: possibility of groundwater near the surface using finer resolution than we have for our orbital radar surveys MARSIS and SHARAD
- 5-3: Evidence of ice within 1 meter of the surface in poleward facing slopes in tropical areas.
However for the most important changes relevant to the habitability of Mars in Jezero crater we need to look at the informal recommendations. These were included as a result of the encouragement of the planetary protection officers of both NASA and ESA.
The review committee’s statement of task (see Preface) did not call for recommendations for future research to reduce uncertainties surrounding the various issues and questions discussed in its report. Nevertheless, the committee was verbally encouraged to do so by the planetary protection officers from NASA and ESA.
In the absence of a formal request for specific research recommendations, the review committee has limited itself to providing suggestions for future research. These suggestions are open ended and are not comprehensive. They are not directed at any particular organization or group and are unlikely to provide new insights or information on a timescale that will impact the Mars missions currently in development. Moreover, since they were not formally requested, they are not developed in any detail and contain few, if any, specifics. Nevertheless, the review committee believes that they represent a good starting point for any future group chartered to study Special Regions and issue formal recommendations.
(SSB, 2015. Review of the MEPAG report on Mars special regions : 45)
These informal recommendations are found as
- extensive comments in the main body of the report in their discussion of the SR-SAG2 findings
- chapter 5 on the utility of maps (SSB, 2015. Review of the MEPAG report on Mars special regions : 30), and
- appendix A, suggestions for future research (SSB, 2015. Review of the MEPAG report on Mars special regions : Appendix A)
From their reply to my comment, it seems likely NASA's team only paid attention to Appendix B and missed the significance of these informal recommendations which outlined many knowledge gaps not covered by SR-SAG2, and so got an incomplete picture of the relevance of this review for Jezero crater.
We can pick out five of these knowledge gaps as of special relevance to Jezero Crater:
- though SR-SAG2 considered microenvironments on Mars, it only briefly considered the implications of our lack of knowledge of them
- microbes are likely to need to develop biofilms, like organic plastic "houses" or cloches, before they can grow in otherwise uninhabitable ecological niches on Mars
- SR-SAG2 didn’t adequately discuss the potential for life to be transported in the dust in the atmosphere (e.g. dust storms)
- there may be more potential for life in caves and subsurface cavities than suggested in SR-SAG2
- Any maps made from orbit such as the ones used in SR-SAG2 can only represent incomplete knowledge about habitability that will certainly be changed
So now let's look at these main knowledge gaps from the SSB review in detail.
SSB Review: though SR-SAG2 considered microenvironments on Mars, it only briefly considered the implications of our lack of knowledge of them
Our best Mars analogue deserts such as the hyperarid core of the Atacama desert may look uninhabited to start with. But then we may spot small patches which are marginally more habitable for microbes, on rocks, under rocks, pores in salt pillars, etc. If we split the rocks open we may detect a touch of green or other colours from the microbes in there. This is a photograph of an endolithic community, living inside a rock in Antarctica.
Original caption: This cryptoendolith (hidden-in-rock in Greek) is one of the strangest life form of all. It is a whole ecosystem growing inside clear rocks, just a few millimeters under the surface and includes an association of bacteria and algae. It is visible as the green stripe on this split rock. Enough light and water gets through the rock for photosynthesis, and that's all you need to live, right ? Picture size about 5cm.
(Dargaud., 1996, Antarctic Animals)
License: GDPL
In very dry regions on Earth, such as the hyperarid core of the Atacama desert, some gypsum salts are habitable and others are almost devoid of life.
The colonization of gypsum crusts within the hyperarid core appears to be controlled by the moisture regime. Our data shows that the threshold for colonization is crossed within the dry core, with abundant colonization in gypsum crusts at one study site, while crusts at a drier site are virtually devoid of life. We show that the cumulative time in 1 year of relative humidity (RH) above 60% is the best parameter to explain the difference in colonization between both sites.
(Wierzchos et al., 2011, Microbial colonization of Ca-sulfate crusts in the hyperarid core of the Atacama Desert: Implications for the search for life on Mars : abstract)
Wierchos et al. see these as a potential analogue for microhabitats for martian life:
The work presented here and work conducted in deserts around the world in the past 40 years, indicate that any putative microbial life on Mars would have likely colonized the insides of rocks and crusts, as they provide natural shelter against harmful radiation and also enhance the moisture conditions on a micro scale.
Sulfate and chloride evaporitic deposits have been suggested as a possible oasis for an extant biota, or the last refuge for an extinct biota on the surface of Mars (Rothschild, 1990; Mancinelli et al., 2004; Barbieri et al., 2006; Dong et al., 2007; Stivaletta et al., 2009; Davila et al., 2010). In this context, terrestrial evaporite rocks, including the Ca-sulfate crusts of the dry core of the Atacama Desert as key terrestrial analogs of Martian aqueous deposits, might prove useful for assessing the habit- ability of the Martian surface, and develop or improve current strategies for the search for life on Mars.
(Wierzchos et al., 2011, Microbial colonization of Ca-sulfate crusts in the hyperarid core of the Atacama Desert: Implications for the search for life on Mars : 14)
ASA's second planetary protection officer, Cassie Conley also mentioned this as another possible habitat in places like Gale crater and Jezero crater:
From the perspective of planetary protection, Conley is also concerned about terrestrial organisms that can absorb water from the air. She recalls fieldwork she did in the Atacama Desert in Chile, which is one of the driest places on Earth, with less than 0.04 inch of rain a year.
Even in this desiccated place, she found life: photosynthetic bacteria that had made a home in tiny chambers within halite salt crystals. There’s a small amount of water retained inside the halite and, at night, it cools down and condenses both on the walls of the chambers and on the surface of the organisms that are sitting there.
(Strauss, 2016, Going to Mars Could Mess Up the Hunt for Alien Life)
So now imagine we found a planet where the situation is the reverse of what it is for Earth. Instead of almost all gypsum habitable except for rare deposits in hyperarid deserts, on this planet, nearly all the gypsum is uninhabitable but there are a few places where there are habitable oases of gypsum due to minute local variations of humidity and micropores in the gypsum. How will an orbiter spot these? How will even a rover spot them if it is not dedicated to life detection? The answer is it is impossible to find such microhabitats without dedicated capabilities to search for such microhabitats in situ.
Mars may be such a planet, though we can't know yet if it is. We haven't yet sent instruments that could search for such microhabitats on Mars or look for life in them.
ILLUSTRATION NEEDS PERMISSION
(f) and (g) show microbial communities entirely contained within the gypsum crust.
(Wierzchos et al., 2011, Microbial colonization of Ca-sulfate crusts in the hyperarid core of the Atacama Desert: Implications for the search for life on Mars : figure 3)
SR-SAG2 looked at this briefly and did say such cryptic habitats are a major challenge to the task of interpreting where to find the Special Regions on Mars (regions where terrestrial life might be likely to grow).
Finding 3-10: Determining the continuity/heterogeneity of microscale conditions over time and space is a major challenge to interpreting when and where Special Regions occur on Mars.
(Rummel et al., 2014. A new analysis of Mars “special regions”: findings of the second MEPAG Special Regions Science Analysis Group (SR-SAG2) : 904)
The SSB didn't modify this finding. However, it commented stressing its significance. It said that the implications of this lack of knowledge were only briefly considered in SR-SAG2
Physical and chemical conditions in microenvironments can be substantially different from those of larger scales. Although the SR-SAG2 report considered the microenvironment (Finding 3-10), the implications of the lack of knowledge about microscale conditions was only briefly considered.
…
Craters, and even microenvironments underneath and on the underside of rocks, could potentially provide favorable conditions for the establishment of life on Mars, potentially leading to the recognition of Special Regions where landscape-scale temperature and humidity conditions would not enable it.
…
The review committee agrees with Finding 3-10 of the SR-SAG2 report but stresses the significance of the microenvironment and the role it might play on the definition of a Special Region in areas that (macroscopically speaking) would not be considered as such.
(SSB, 2015. Review of the MEPAG report on Mars special regions : 11 - 12 )
SR-SAG2 gives a list of seven naturally occurring microenvironments on Mars:
- Vapor-phase water available Vapor or aerosols in planet’s atmosphere; within soil cavities, porous rocks, etc.; within or beneath spacecraft or spacecraft debris
- Ice-related Liquid or vapor-phase water coming off frost, solid ice, regolith or subsurface ice crystals, glaciers
- Brine-related Liquid water in deliquescing salts, in channels within ice, on the surface of ice, within salt crystals within halite or other types of ‘‘rock salt’’
- Aqueous films on rock or soil grains Liquid water on regolith particles of their components such as clay minerals, on surface of ice, on and within rocks, on surfaces of spacecraft
- Groundwater and thermal springs (macroenvironments) Liquid water
- Places receiving periodic condensation or dew Liquid water on regolith particles of their components such as clay minerals, on surface of ice, on and within rocks, on surfaces of spacecraft
- Water in minerals Liquid water bound to minerals
(Rummel et al., 2014. A new analysis of Mars “special regions”: findings of the second MEPAG Special Regions Science Analysis Group (SR-SAG2) : 904)
These continue to be of great interest for potential microhabitats on or near the surface of Mars. As we'll see some are relevant to Jezero crater. See below:
SSB Review of SR-SAG2: microbes are likely to need to develop biofilms, like organic plastic "houses" or cloches, before they can grow in otherwise uninhabitable ecological niches on Mars
Just as we are able to use shelter, clothes, fire, to live in places too cold or inhospitable for humans to live comfortably, microbes also can modify their surroundings to make them more habitable. They do this using biofilms, communities of species working together to their mutual benefit. They extrude bioplastics to cover themselves and they modify the conditions around them to live in places they might not be able to live in otherwise. Even if Mars does have microbial oases, they might be just on the verge of habitability and it may be hard for a single microbe to colonize a microhabitat. But once it starts to grow it can then form a community, a biofilm and other species with other capabilities can join in and complement it to make it more and more habitable.
Interestingly, biofilms can also be propagated as fragments. This is like Dorothy's house in the Wizard of Oz, an entire fragment of biofilm can be lifted up and fall in a different place where it already forms a habitat for the microbes to live in.
Text on Graphic: A fragment of biofilm can land in a desert as a readymade "home" for microbes much like Dorothy's house in "Wizard of Oz". In this way native biofilms might colonize places where single microbes can never grow on their own.
(Radin, 2007, Dorothy's House after the storm)
Native biofilms could use this to live in a place where they couldn't live at all if they got their one by one as individual microbes. This might let biofilms inhabit present day Mars even if there is nowhere left that they can colonize one by one as individual microbes. This is a proposal from Mosca et al. (Mosca et all., 2019, Over-expression of UV-damage DNA repair genes and ribonucleic acid persistence contribute to the resilience of dried biofilms of the desert cyanobacetrium Chroococcidiopsis exposed to Mars-like UV flux and long-term desiccation)
For details see below:
SR-SAG2 has only one mention of biofilms. They are mentioned in passing in the first of its list of eight knowledge gaps.
The synergy of multiple factors that enable enhanced microbial survival and growth (i.e., storage mechanisms, biofilms, and the structure of microbial communities), and mechanisms that may allow for temporal separation in microbial resource use.
(Rummel et al., 2014. A new analysis of Mars “special regions”: findings of the second MEPAG Special Regions Science Analysis Group (SR-SAG2) : 7.5 Knowledge gaps)
The SSB review has an extensive discussion of this topic, saying it is likely that any terrestrial stowaways to Mars would need to develop biofilms to grow and replicate on Mars.
Given the wide distribution and advantages that communities of organisms have when they live as biofilms enmeshed in copious amounts of EPS [organic plastics exuded by microbes], it is likely that any microbial stowaways that could survive the trip to Mars would need to develop biofilms to be able to establish themselves in clement microenvironments in Special Regions so that they could grow and replicate.
(SSB, 2015. Review of the MEPAG report on Mars special regions : 11)
The 2015 Space Studies Board review starts the biofilm section expanding on that knowledge gap, saying that multi-species biofilms are highly organized structures, with the cells immersed in a film of many organic chemicals which they exude to protect themselves and make the film more habitable for themselves. The general term for these protective organics they exude is "Extrapolymeric substances", or EPS for short, where "polymeric" means they form a sort of natural organic plastic (i.e. made up of smaller chemical units attached together in larger macro-molecules).
The SR-SAG2 report identified the ability of microorganisms to withstand multiple stressors as an important area of research. Extreme ecosystems on Earth are often subjected to a multitude of conditions considered to push the limits of microbial life.
In nature, microorganisms typically live and proliferate as members of communities rather than as single cells or populations. A widespread growth form of life in natural habitats occurs as multispecies biofilms where the cells are embedded in a self-produced extracellular matrix consisting of polysaccharides and proteins, which includes other macromolecules such as lipids and DNA. These so-called extrapolymeric substances (EPS) provide protection against different environmental stressors (e.g., desiccation, radiation, harmful chemical agents, and predators). Biofilms are highly organized structures that enable microbial communication via signaling molecules, disperse cells and EPS, distribute nutrients and release metabolites, and facilitate horizontal gene transfer.
(SSB, 2015, Review of the MEPAG report on Mars special regions :11).
Here is a graphic to show the idea (not from the SSB board report - they don't have any graphics on biofilms)
Text on graphic: How EPS (extrapolymeric substances) can make a “home” of the hostile martian surface.
Some of the environment stressors
100% humidity varies to 0%
Heat, cold, UV, dust storms
Oxidants, nutrients
Algae may add oxygen
Retains moisture from night to daytime when temperature soars from -70°C to above 0°C.
Cryoprotectants - protects from cold shock
Extrapolymeric substances (EPS): proteins, DNA, lipids, polysaccharides, other large organic molecules.
A biofilm is like a microbe's "house" which can keep it warm, wet, protected from UV, and which it shares with other microbes
Graphic adapted from (Sabater et al., 2016, Stream biofilm responses to flow intermittency: from cells to ecosystems : fig. 2)
These biofilms are like microbial "homes" or one can think of the organic plastic coverings as a kind of miniature polytunnel or garden cloche to protect them from the elements.
Text on graphic: Microbes on Mars might use organic plastics to construct biofilms, structures they use to protect themselves like miniature versions of garden cloches
(McCain, 2010, Cloche, bell jar, in Colonel Williamsberg garden)
Mars might have microbial "almost oases" just on the verge of habitability. Microbes in deserts often use biofilms, thin layers of microbes, often multi-species, working together to modify the environment locally to their liking at a microscopic scale. These can form in two ways. One is that a few solitary microbes find the oasis, are able to grow slowly and as they grow they make the conditions there more habitable for themselves, and then other microbes join in that can now grow there which couldn't before. The other way it can happen is that a fragment of a biofilm can be broken off in the wind and blow to a new place where it can set up home a bit like a
SSB Review: SR-SAG2 didn’t adequately discuss the potential for life to be transported in the dust in the atmosphere (e.g. dust storms)
"The SR-SAG2 report does not adequately discuss the transport of material in the martian atmosphere. The issue is especially worthy of consideration because if survival is possible during atmospheric transport, the designation of Special Regions becomes more difficult, or even irrelevant."
(SSB, 2015. Review of the MEPAG report on Mars special regions :12)
Here, as we already mentioned, “special regions” are regions where terrestrial organisms are likely to propagate or with high potential for extant martian life (SSB, 2015. Review of the MEPAG report on Mars special regions : 6). The second half of the definition isn’t used much given that we don’t yet know capabilities of any putative martian life.
The 2015 SSB review says this is an important knowledge gap to look at because if terrestrial life can be spread from anywhere to anywhere on Mars it becomes much harder or impossible to map out safe regions for forward contamination.
We still don't have anything like enough information to answer the question. Can a fragment of a terrestrial biofilm can be transported in the dust storms? Can terrestrial life even get transported as single microbes protected, perhaps in a crack in a dust grain, on rare occasions. We need a far better understanding of the martian dust and martian chemistry and conditions to fill this knowledge gap.
However, so far experiments suggest it may be possible. One experiment by Billi et al. found that a fragment of biofilm only three hundredths of a millimeter thick could be transported for 8 hours in daylight, and still be viable which is enough time to travel 100 kilometers (further if one takes account of transport at night and in dust storms). martian life adapted to the conditions over billions of years may be abe to travel further before it is sterilized (Billi et al., 2019. A desert cyanobacterium under simulated Mars-like conditions in low Earth orbit: implications for the habitability of Mars). This experiment only considered effects of UV not the effects of perchlorates activated by ionizing radiation to chlorates and chlorites. However, a biofilm might well be resilient to those too.
These experiments also use terrestrial life with no adaptations specific to the conditions on Mars. Any native martian life is likely to have many specific adaptations to be more resilient in the extreme martian conditions. Martian biofilms would likely use EPS (biopolymers) as for terrestrial biofilms but adapted to martian conditions, and other strategies to protect themselves
For a more detailed look at whether native martian life could spread in the dust storms see the section below:
- How low temperatures, dust, shadows, and the protective layers such as biopolymers in biofilms could protect native microbes against harm from UV activated perchlorates and help them survive travel for long distances in dust storms
SSB Review: there may be more potential for life in caves and subsurface cavities than suggested in SR-SAG2
This is another finding that is listed as "no change" but the SSB Review adds substantially to the potential for knowledge gaps in its comments on the finding. The SR-SAG2 finding is:
SR-SAG2 Finding 4-11: On Earth, special geomorphic regions such as caves can provide radically different environments from the immediately overlying surface environments providing enhanced levels of environmental protection for potential contaminating organisms. The extent of such geomorphic regions on Mars and their enhancement (if any) of habitability are currently unknown.”
No change.
(SSB, 2015. Review of the MEPAG report on Mars special regions : 24)
SR-SAG2 lists the possibilities as:
- Drained lava tubes
- Caves formed by tension fractures
- Caves in the salts left after evaporation of salty water – e.g. in gypsum
None of those are of special interest for present day habitability though caves in salts would be of great interest for past habitability. However the Space Studies Board review adds:
- Caves formed by water running underground (like many terrestrial caves)
- Caves formed by mud volcanoes or material expelled by hydrothermal processes
The SSB review adds that the consideration of caves is paramount, because
- They provide a protected environment (e.g. from extreme cold and radiation)
- They provide a way that terrestrial contamination can access environments in the deep subsurface of Mars:
It also gives two types of potentially more habitable subsurface cavities not listed in SR-SAG2. This is how they put it:
Consideration of caves and subsurface cavities is paramount for two reasons. First, they provide a protected environment (e.g., from extremely low temperatures and radiation). Second, they can provide a means by which terrestrial contamination can access martian subsurface environments. In addition to drained lava tubes, voids resulting from tension fracturing, and possible caves in evaporites (e.g., gypsum karst), there are types of subsurface cavities on Mars not mentioned in the SR-SAG2 report that may have been produced by subsurface erosion by water (analogous to piping; e.g., Higgins and Coates 1990) or by expulsion of material through hydrothermalism (Rodríguez et al. 2005) or mud volcanism (Rodríguez et al. 2012). However, to the best of the review committee’s knowledge, there is no data on the availability of water in martian caves.
(SSB, 2015. Review of the MEPAG report on Mars special regions : 24)
The SSB review looks at potential sources of chemical energy in caves, and finds several possibilities. It concludes the discussion of caves saying:
In conclusion, there could be a number of possible primary sources of the necessary ingredients for life inside caves and subsurface cavities on Mars, and therefore, they are best classified as Uncertain Regions and treated as Special Regions until proven otherwise.
(SSB, 2015. Review of the MEPAG report on Mars special regions : 25)
Penelope Boston added several other potential caves not mentioned by either SR-SAG2 or the SSB review: glacier caves, wind scoured caves, caves formed from water by moving fine particles instead of dissolving the rock, and caves formed by ice and dry ice subliming directly into the atmosphere. . Location, location, location! Lava caves on Mars for habitat, resources, and the search for life):
This continues to be an ongoing area of research and caves are seen as one of the highest priority targets to search for life on Mars. These might be able to vent life to the surface. See below:
SSB Review: Any maps made from orbit such as the ones used in SR-SAG2 can only represent incomplete knowledge about habitability that will certainly be changed
The SSB Review of SR-SAG2 saw this issue as so important, the whole of chapter 5 is devoted to issues with using maps to mark out special regions from orbit. Chapter 5: Generalization of special regions and the utility of maps (SSB, 2015. Review of the MEPAG report on Mars special regions : 30). This chapter is not presented as a new finding or a revision of a finding but it warned that the use of maps in SR-SAG2 to mark out regions from orbit is not appropriate.
Maps that illustrate the distribution of specific relevant landforms or other surface features can only represent the current (and incomplete) state of knowledge for a specific time—knowledge that will certainly be subject to change or be updated as new information is obtained
(SSB, 2015. Review of the MEPAG report on Mars special regions : 28)
SR-SAG2 didn't present their recommendation to use maps as a specific finding, it is more of an underlying assumption behind the approach of SR-SAG", so the SSB review had no finding to modify here. So though the SSB review has an entire chapter on the topic, this isn't mentioned in the list of findings and revisions in Appendix B.
This is what it says.
Another potential source of misinterpretation related to the use of maps in Special Region studies is the issue of scale. Identification of a Special Region needs a multiscale approach (see also the discussion in Chapter 2, “Detectability of Potential Small Scale Microbial Habitats,” and thus, as far as missions to Mars are concerned, conservatism demands that each landing ellipse be scrutinized on a case-by-case basis. Maps, which come necessarily at a fixed scale, can only provide information at that scale and are, therefore, generalizations.
…
In general, the review committee contends that the use of maps to delineate regions with a lower or higher probability to host Special Regions is most useful if the maps are accompanied by cautionary remarks on their limitations. Maps that illustrate the distribution of specific relevant landforms or other surface features can only represent the current (and incomplete) state of knowledge for a specific time—knowledge that will certainly be subject to change or be updated as new information is obtained(SSB, 2015. Review of the MEPAG report on Mars special regions : 28)
This finding on the inadequacy of maps is one of the major new findings of the 2015 review if they'd been able to present new findings. It is of course directly relevant to Jezero crater which was classified as not a special region based on maps which could only be done using orbital images.
Perseverance has very limited abilities to detect local microenvironments in Jezero crater. It is less capable than Curiosity without the DAN instrument for detecting subsurface water molecules. Perseverance can't detect the cold salty brines Curiosity found. It may be able to detect micron thick layers of frosts however.
SSB Review's suggestions for future research to expand knowledge about habitability of Mars
The Appendix A suggestions for future research includes to expand knowledge about microbial processes and habitability at micron scales and develop the methods needed to search for such microhabitats on other planets like Mars:
Detectability of Potential Small-Scale Microbial Habitats
Perform in situ investigations in extreme environments on Earth to deepen our knowledge about microbial processes and habitability at micron scales. Adapt and optimize existing technologies and develop new ones to undertake the kind of investigations which may be used in the future exploratory missions to other planets and moons of astrobiological relevance.
(SSB, 2015. Review of the MEPAG report on Mars special regions : 46)
Also research into microbial communities (such as biofilms), the ability of terrestrial microbial communities to adapt to martian conditions over time if not initially able to colonize them.
Life in Extreme Environments and in Multispecies Communities
Investigate the effects of successively and simultaneously applied multiple Mars-relevant environmental stress factors on microbial isolates and microbial communities to improve understanding of the mechanisms of adaptation, the evolutionary process whereby an organism becomes better able to live in habitats subject to extreme environmental conditions.
(SSB, 2015. Review of the MEPAG report on Mars special regions : 46)
It also suggests future research into transport of microbes and spores in the atmosphere, for instance in dust storms
Translocation of Terrestrial Contamination
Undertake investigations of transport mechanisms and microbial viability in Mars simulation chambers—e.g., the Mars Surface Wind Tunnel facility at NASA’s Ames Research Center or the low-pressure recirculating wind tunnels in the Mars Simulation Laboratory at Aarhus University—wherein microbes and spores are exposed to Mars-relevant levels of ultraviolet radiation, desiccation, nutrient deficit, and air movement, to assess the likelihood of survival during transport by, for example, dust storms.
(SSB, 2015. Review of the MEPAG report on Mars special regions : 46)
For some of the research done since then on transport of terrestrial contamination in dust storms see below:
- How low temperatures, dust, shadows, and the protective layers such as biopolymers in biofilms could protect native microbes against harm from UV activated perchlorates and help them survive travel for long distances in dust storms
It also suggests future research into the Polar dark dune streaks, and snow and ice deposits
Shallow Subsurface Conditions and Ice Deposits
Studies to address the formation of liquid brines on past and future landing sites, the kinetic factors involved, and their implication for microbial growth.
Snow, Ice Deposits, and Subsurface Ice
Research addressing the range of conditions in which liquid brines could form and be temporarily stable on the surface and shallow subsurface of Mars.
(SSB, 2015. Review of the MEPAG report on Mars special regions : 47)
This serious omission is likely to be an issue with team selection, with no members with the necessary background knowledge of the planetary protection literature to catch the mistake
With this background that the knowledge gaps that the SSB 2015 review identified were directly relevant to Jezero crater, we see that it was indeed a serious omission to not consider this study, especially chapter 5 and appendix A and the detailed discussions of each finding.
However from the other omissions, such as not being aware of the rebuttal of the Mars meteorite argument in their own cite, or the discrepancy between their statements in the PEIS and the plans to test the samples for present day life, it seems credible that NASA's current team just didn't have the background knowledge of the planetary protection literature to recognize that it was a serious omission to leave out this study.
There is nothing here to suggest any intent to withhold information. It seems more an issue with team selection, and suggests NASA's team didn't have any members with the relevant knowledge of the planetary protection literature to catch these mistakes.
These comments and knowledge gaps in the SSB review change the picture for Jezero crater in a major way.
If there is life in Jezero crater, it is likely to be present in ultra-low concentrations, lower than even in hyperarid terrestrial deserts, but we do not know that it is completely uninhabited
Most would probably still say there isn't a very high potential for martian life in Jezero crater, but we can't say Jezero crater is uninhabited, especially by martian life adapted to local conditions. As we saw above, the iMost team already has plans for experimenters to see if they can get life to grow from the samples:
Earlier this century, Mars seemed uninhabitable but on closer inspection we see many potential ways for life to survive there. This barely habitable Mars might seem to make surface life unlikely. Surely at times, it would be less habitable than today and any life would go extinct?
Lovelock reasoned with his Gaia hypothesis in the 1970s that if there is surface life on Mars it would have modified the environment to take it well out of equilibrium and more habitable for itself (Lovelock, 1975. Thermodynamics and the recognition of alien biospheres). We certainly don't see that. (His reasoning doesn't apply to deep subsurface life, for instance in caves with no access to the surface).
Others since then have found ways that life can form an "anti-Gaia" that could rapidly undermine the conditions for its own existence (Gaspirini, 2022, Life on Mars May Have Been Its Own Worst Enemy). That would seem to make the situation even worse. Even if Mars had life in the past it likely made itself extinct and if it got habitable enough for life to re-evolve at a later date it would once more make itself extinct, or retreated to deep below the surface.
However, neither hypothesis explains why would we see an atmosphere so close to uninhabitable but not quite totally uninhabitable. Indeed the combination of atmospheric pressure and temperature is close to the triple point of water, where water can change from water to solid to gas, at around 0°C and 6.1 millibars of pressure.
Some authors have suggested this may be more than a coincidence, and that there may be abiotic processes such as carbonate formation and abiotic photosynthesis that take carbon dioxide out of the atmosphere whenever it gets warm enough for widespread water to form. This would keep Mars indefinitely at just barely habitable except from time to time when a pulse of carbon dioxide would make it more habitable briefly until the abiotic processes removed it again (Nolan, 2008. Mars: A cosmic stepping stone. In MARS A Cosmic Stepping Stone : 137) (Kahn, 1985. The evolution of CO2 on Mars) (Haberle et al., 2001. On the possibility of liquid water on present ‐ day Mars). Unlike Earth, Mars has no continental drift to return large deposits of carbonates or organics to the atmosphere after they form. Here a point in favour is the large quantities of carbonates on the surface of Mars, enough in the dust alone for double Earth's atmospheric pressure of carbon dioxide (Bandfield et al., 2003. Spectroscopic identification of carbonate minerals in the Martian dust) (Niles et al., 2013. Geochemistry of carbonates on Mars: implications for climate history and nature of aqueous environments : section 3.1).
This book presents a new suggestion, that life itself could amplify this effect, for instance that biotic photosynthesis could have a similar feedback effect to abiotic photosynthesis but over a much wider range of CO2 emission scenarios for the Martian volcanoes. In short that without life Mars might still have running water and lakes much of the time but life removes any CO2 rapidly as soon as such lakes form through photosynthesis.
With this hypothesis what we see on Mars, a barely habitable Mars, rather than a completely uninhabitable Mars, might actually be a weak biosignature of life.
O'Malley-James coined the term "Swansong biosphere" for a biosphere that is gradually becoming less habitable but is not yet uninhabitable (O'Malley-James et al., 2013. Swansong biospheres: refuges for life and novel microbial biospheres on terrestrial planets near the end of their habitable lifetimes) (O'Malley-James et al., 2014. Swansong biospheres II: The final signs of life on terrestrial planets near the end of their habitable lifetimes). That suggests the term Swansong Gaia for this hypothesis of biological pumps removing warming gases in a feedback process to maintain a planet indefinitely in a barely habitable Swansong state. Barely habitable but never uninhabitable enough to extinguish the life. On this hypothesis, without life it would be far more habitable, possibly even with a carbon dioxide atmosphere today of up to several bars given the amounts of carbonates sequestered in dust and rocks on Mars.
There are many other cycles to consider here involving carbon dioxide, methane, hydrogen and nitrogen. We look at this in:
At present there is a physical process that keeps the atmospheric pressure at 6 millibars which rapidly restores the atmosphere to the current 6 millibars by depositing dry ice at the poles, whenever it goes above that pressure. However at times of higher axial tilt the dry ice sublimes completely and raises the atmospheric pressure to around 12 millibars with a possible range of 7.6 to 14.5 millibars depending on how much gets absorbed in or released from the regolith as the dry ice evaporates(Buhler et al., 2021, Obliquity-Driven CO2 Exchange Between Mars' Atmosphere, Regolith, and Polar Cap : table 1). If this is maximum atmospheric pressure was reduced to this level by biological pump presumably the biological pump weakens to the point where it has minimal effect below around 12 millibars due to the small amounts of liquid water available. For details see:
So far, we have focused so far on water and temperature. There are various chemical energy sources that life on Mars can use for its metabolism, and if it is able to use photosynthesis that's widely available on the surface. Life on Mars would be able to get all the trace elements it needs from basalt.
However one element is in short supply, nitrogen. Nitrates dissolve readily in water and there seems to be little by way of nitrates left on the surface of Mars. So, can present day life use nitrogen fixation in the very thin Martian atmosphere? If not, present day life would be restricted to regions with readily available sources of fixed nitrogen. Or is nitrogen fixation possibly but only barely so? If so we might find ultra low levels of life in areas without abiotic fixed nitrogen. Then if life is involved in using and producing nitrogen that might be another feedback to take account of in Swansong Gaia. We will find that if Martian nitrogen fixers and microbes that return nitrogen to the atmosphere work similarly to the microbes in our terrestrial Mars analogue deserts, this could be a feedback to keep the nitrogen levels low enough to be just barely able to sustain nitrogen fixing life over the surface of Mars, another cycle that may reinforce a Swansong Gaia.
We look at this issue below in:
How these knowledge gaps affect planetary protection: robotic spacecraft unchanged, many astrobiologists say human missions might start a martian "anthropocene" and increased though still low chance for native martian life for sample returns
Let's look again at our list of some of the major knowledge gaps of special relevance to the potential for terrestrial life to grow in Jezero crater. The 2015 SSB Review of SR-SAG2 said that
- though SR-SAG2 considered microenvironments on Mars, it only briefly considered the implications of our lack of knowledge of them
- microbes are likely to need to develop biofilms, like organic plastic "houses" or cloches, before they can grow in otherwise uninhabitable ecological niches on Mars
- SR-SAG2 didn’t adequately discuss the potential for life to be transported in the dust in the atmosphere (e.g. dust storms)
- any maps from orbit can only represent incomplete knowledge about habitability that will certainly be changed
- there may be more potential for life in caves than suggested in SR-SAG2
Since these knowledge gaps were identified in the 2015 SSB review of SR-SAG2, they have been explored further, and found to be significant as we'll see in the rest of this book, with much more research needed on Mars before we can resolve them.
The SSB review didn't lead to any immediate changes in recommendations for sterilization to prevent forward contamination protection for category IVa robotic missions. We may not be as sure of the uninhabitability of Jezero crater as previously, but given how cold and dry it is, Perseverance is still considered adequately sterilized to ensure a very low risk of forward contamination of Jezero crater. See below:
However it is clear these knowledge gaps are relevant to human landings missions to Jezero crater because of the higher bioloads and the potential for contaminating Mars not only with single microbial spores and dormant microbes, but with biofilm fragments too, which can establish life in places single microbes may not be able to colonize.
We might think that Perseverance could help us investigate to see if there are microhabitats in Jezero crater, but it is not designed to look for them. Perseverance doesn't even have the instrument that Curiosity used to detect subsurface temporary ultracold salty water in the top few cms of the so. We cover this below in:
Also as with all the missions since Viking, Perseverance has no in situ life detection capabilities.
The SSB Review didn't explicitly apply the knowledge gaps to human missions. Instead, it acknowledged that this was not the place for a detailed examination of the issues for human missions, but commented that the SR-SAG2 discussion of human landings wasn't as thorough as the rest of it.
The review committee noted that the impact of human spaceflight on planetary protection, in general, and Special Regions, in particular, had not been considered with the same rigor and thoroughness that had been applied to other parts of the SR-SAG2 report
(SSB, 2015. Review of the MEPAG report on Mars special regions : 26) .
There were no findings in SR-SAG2 for human landings and the SSB Review felt that a minimal amount should be said. It proposed a new finding, which of course isn't listed in Appendix B as that is only about revisions of existing findings:
New Finding 6-1: Human missions to Mars are required to fully follow the planetary protection requirements specified by COSPAR, including the limitations specified for Special Regions. This may prevent humans from landing in or entering areas that may be Special Regions or may become Special Regions through modifications of the environment by space systems and/or human explorers.
(SSB, 2015. Review of the MEPAG report on Mars special regions : 27)
This is also covered by the SSB Review in Appendix A Suggestions for future research (for the knowledge gaps they identified)
Conduct research and related activities to understand the implications of landing and operating human systems on Mars in a manner consistent with COSPAR planetary protection policy.
(SSB, 2015. Review of the MEPAG report on Mars special regions : 46)
Scientists studying our closest Mars analogue deserts, the cold dry McMurdo dry valleys in Antarctica, use a corral type system to reduce contamination of the deserts by the microbes they bring with them on their own bodies to a small region around the research camp (Cowan et al., 2011. Non-indigenous microorganisms in the Antarctic: Assessing the risks : boxes 1 and 2). Could the hyperarid Martian deserts also be vulnerable to contamination after a human landing, that would confuse our science experiments?.
Few outside of the astrobiology literature seem to be aware that one of the most important debates in the planetary protection literature today is about how much research we can do on Mars before humans introduce terrestrial life to Mars globally and irreversibly. The debaters call this the martian "anthropocene". As late as the first decade of this century, most astrobiologists thought human missions to Mars could only introduce terrestrial contamination locally, as for the Moon. This in principle could be reversed by sterilizing the landing site for the human mission. Now though, most astrobiologists say this local contamination is likely to spread irreversibly and globally. The difference from local contamination in Antarctica is that this is happening in a planet that may have never seen terrestrial life or never seen modern terrestrial life.
The SSB Review didn't originate the debate on the risk of a human mission starting an anthropocene on Mars and never suggests this idea. Rather, it marked a milestone in the growing body of research that informed their identification of the knowledge gaps in SR-SAG2. This led on to this debate a couple of years later.
A future comprehensive review of forward contamination of Mars would be sure to look at this topic of whether or not humans landing on Mars can start a martian anthropocene.
All participants in this debate, which spans numerous papers in the journals, agree that the first human landings could start the martian anthropocene, The debate focuses on whether or not we should relax planetary protection for robots. Both sides recognize the science per mission will be less valuable, but those advocating "dirty robots" see it as a way to send missions to the regions of Mars most likely to have potential for native martian life, to get more science done before the anthropocene likely ends our opportunities to study Mars as it is before the martian anthropocene begin . NASA's first and second planetary protection officers John Rummel and Cassie Conley argue that it is some time still before humans get to Mars. They say this gives us time to do careful science in regions with a higher chance of present day life and we should press for NASA to use clean robots sterilized to Viking standards rather than dirty robots even though this can add 10% per mission to the cost. See below:
Not all astrobiologists agree that the first human mission would start an anthropocene on Mars. NASA scientist astrobiologist / engineer Chris McKay presents as his expert opinion that Mars can be explored reversibly after a human expedition. Others may be of the same opinion but his is the only paper I've seen published arguing this way. This is a significant expert opinion, but it seems to be a minority opinion of one author, at least in the published papers on the topic.
The knowledge gaps raised in the SSB review need to be resolved before we can know who is right, astrobiologists who agree with Chris McKay or the astrobiologists who debate in astrobiology journals how much science we can do before the martian anthropocene.
These discussions seem to be carried out in parallel, at least in the literature. I haven't found yet any papers debating the cases for or against the potential for a martian anthropocene on Mars.
As for the relevance of all this to backwards contamination of Earth by martian life; as a forwards contamination study the main focus of SR-SAG2 and the SSB review is whether terrestrial life might be able to grow there. It doesn't look at the potential for native martian life that may have novel capabilities terrestrial life doesn't have. It just says
At present there are no Special Regions defined by the existence of extant martian life, and this study concentrates only on the first aspect of the definition.
(Rummel et al., 2014. A new analysis of Mars “special regions”: findings of the second MEPAG Special Regions Science Analysis Group (SR-SAG2) : 888)
However, the same knowledge gaps also increase the potential for native martian life to be there already, with native life using biofilms or spreading in the dust storms just as terrestrial life might do after a human landing.
If there is any life on Mars, it has had millions of years to find habitats, adapt to them and build up biofilms which it too could use to colonize new places. It also has had billions of years of evolution to adapt to the surface conditions, so what terrestrial life from a human mission might be able to do, native martian life could potentially do too, and perhaps more easily.
Neither SR-SAG2 nor the SSB review of it looked at Martian life which would have had time to colonize new microhabitats over timescales of millennia or even millions of years. It would also be adapted to the martian conditions over billions of years.
However there is much less effect of these knowledge gaps on the isolated microbes on our partially sterilized rovers to Mars. If there are microhabitats there, they are far less likely to find them with the low bioloads, and wouldn't have the protection of other microbes in a biofilm for local habitats or for transfer in dust storms.
What Perseverance's planetary protection classification IVa means: likely very low risk for a partially sterilized rover to introduce life irreversibly and not known to have a high potential for martian life
This takes us to NASA's second cite for its statement that the martian surface is too inhospitable for life.
Consensus opinion within the astrobiology scientific community supports a conclusion that the martian surface is too inhospitable for life to survive there today, particularly at the location and shallow depth (6.4 centimeters [2.5 inches]) being sampled by the Perseverance rover in Jezero Crater, which was chosen as the sampling area because it could have had the right conditions to support life in the ancient past, billions of years ago (Rummel et al. 2014, Grant et al. 2018).
[Rummel et al., 2014 is often referred to as SR-SAG2]
(NASA, 2023. Mars Sample Return final PEIS : S-4)
Grant et al. is about the process of selection of the landing site. This is its only mention of planetary protection, explaining why one of the proposed sites was excluded, Vastitas Borealis:
Vastitas Borealis exceeds the latitude limits and violates planetary protection issues related to near-surface water ice.
(Grant et al.,, 2011 . The science process for selecting the landing site for the 2011 Mars Science Laboratory)
Though what NASA's second cite says is uncontroversial, the implications are often not well understood. Perhaps this cite is included to draw attention to the fact that Jezero crater doesn't have planetary protection issues according to the COSPAR requirements.
It's easy to assume, falsely, that since there are no planetary protection issues for Jezero crater, the crater must be uninhabitable. But that is not how the COSPAR classification system for Mars works. Instead, it means that there are no planetary protection issues, so long as NASA is careful to make sure the rover is partially sterilized.
Perseverance does have to have a level of sterilization for Jezero crater. The current COSPAR guidelines don't permit sending an unsterilized rover to Jezero Crate, as we don't know that it is impossible for terrestrial life to spread there.
Jezero crater is so inhospitable, the risk of terrestrial contamination of Mars from the Perseverance mission is expected to be significantly below 1 chance in 1000. However even very dry terrestrial deserts have traces of life and the risk of forward contamination is not known to be zero. A human mission to the same site might have a far higher risk of terrestrial contamination.
So let's look at this in a little more detail. To expand on that sentence, the reason Vastitas Borealis had to be excluded is that Perseverance isn't sufficiently sterilized to visit any "Special Regions", locations on Mars where terrestrial life is likely to grow, or considered to have a high potential for present day martian life
A Special Region is defined as a region within which terrestrial organisms are likely to replicate ...
Any region which is interpreted to have a high potential for the existence of extant martian life forms is also defined as a Special Region.
(COSPAR,2020, COSPAR Policy on Planetary Protection : 6).
An example would be a region with a habitable layer of fresh water with nutrients sufficient to support terrestrial life.
As for martian life, it's currently impossible to have a clear idea of what independently evolved life on Mars might be able to do. This part of the definition is not currently used for planetary protection assessments.
In the absence of specific information, no Special Regions are currently identified on the basis of possible martian life forms. If and when information becomes available on this subject, Special Regions will be further defined
on that basis(COSPAR,2020, COSPAR Policy on Planetary Protection : 7)
Special Regions require a higher level of sterilization. This higher level of sterilization is also needed for missions searching for present day martian life.
We don't have any known "Special regions" on Mars so far. However we have uncertain regions with potential to turn out to be special regions on closer investigation. This is why Perseverance couldn't visit Vastitas Borealis.
All missions to the Mars surface are category IV under COSPAR which means a significant chance of contamination with terrestrial life.
Category IV missions ... to a target body of chemical evolution and/or origin of life interest and for which scientific opinion provides a significant chance of contamination which could compromise future investigations.
(COSPAR,2020, COSPAR Policy on Planetary Protection : 2)
The three categories used to decide the level of sterilization for landers on Mars are:
- Category IVa. Lander systems not carrying instruments for the investigations of extant martian life ...
[All missions so far except the Phoenix lander and Viking]- Category IVb. For lander systems designed to investigate extant martian life ...
[Viking and the Phoenix lander arm]- Category IVc. For missions which investigate Mars Special Regions
[So far no missions sent to Mars to investigate uncertain regions that may be special regions - Phoenix lander was sent to a region which is now classified as an uncertain region due to its discoveries, but wasn't classified in that way at the time of the mission](COSPAR,2020, COSPAR Policy on Planetary Protection : 5)
All our rovers are still sterilized to at least Category IVa standard because we do not know enough yet to drop the sterilization requirement altogether for any location on Mars.
Categories IVb and IVc require sterilization of either the entire spacecraft, or part of it to a high level of sterilization, the same level of sterilization as the Viking landers (Beauchamp, 2012. Assessment of planetary protection and contamination control technologies for future planetary science missions : 14). These were baked in an oven at 112°C for 30 hours (Viking 1) or 23 hours (Viking 2) (DeVincenzi et al., 1998. Planetary protection, sample return missions and Mars exploration: History, status, and future needs : appendix). . The aim of the sterilization for the Viking mission was to reduce the per-mission risk of forward contamination of Mars with life that can replicate there to less than 1 in 1,000 (DeVincenzi et al., 1998. Planetary protection, sample return missions and Mars exploration: History, status, and future needs : 3.3).
Category IVa requires sterilization to the pre-sterilization stage for Viking, before it was put in the oven. The reasoning is that the harsh conditions on Mars reduce the number of viable spores on the spacecraft at least 10,000 fold, reducing the risk of forward contamination to a level similar to the target probability for Viking (Coustenis, 2020. COSPAR Policy on Planetary Protection Prepared by the COSPAR Panel on Planetary Protection and approved by the COSPAR Bureau on : 6). As Cassie Conley described it:
She said that at launch, there were probably 20,000 to 40,000 heat-resistant bacterial spores on Curiosity, and perhaps 100 or 1,000 times more microbes not counted. Many of them would have since perished in the vacuum of space. Intense ultraviolet radiation on the Martian surface would have killed many more — but not all, and some drop in the soil as Curiosity trundles by and performs its science work.
(Chang, 2015, Mars Is Pretty Clean. Her Job at NASA Is to Keep It That Way)
The requirement for a per mission calculation of the risk of contamination was dropped with this new approach of sterilization by category of the mission sent to Mars (DeVincenzi, 1998. Refinement of planetary protection policy for Mars missions). Individual microbes likely do drop off into the surface still viable, but an individual microbe is not likely to find it easy to survive in the very harsh conditions it encounters. Even if life there is possible, the microbes are not likely to be pre-adapted to the conditions which also likely require biofilms, colonies of microbes for a decent chance of survival according to the SSB review as we'll see below:
Perseverance's Category IVa classification is the reason for its focus on past life. Astrobiologists would prefer for NASA to send it to a region which might have a higher potential for present day life, but NASA can't do this because it is not sufficiently sterilized.
So, in short, Perseverance's Category IVa classification does NOT make it impossible for terrestrial life to grow in Jezero crater. It just means it is considered unlikely terrestrial life can grow there.
In more technical detail, it means that based on our understanding of Mars, we consider that a rover with a Viking pre-sterilization bioload, further reduced by the harsh conditions on Mars, will be at least as sterile as the Viking landers were after they were baked in an over for 23 hours / 30 hours at 112°C. This in turn likely means the chance of contaminating Mars with replicating terrestrial life is less than 1 chance in 1000 per mission (probably considerably less than a 1 chance in a 1000 given how harsh the conditions are in Jezero crater).
Similarly it does NOT mean there is no martian life there. It just means Jezero crater is not currently thought to have a high potential for present day martian life based on our knowledge so far, which is very limited. Martian life might conceivably be capable, for instance, be able to live in cold salty water beyond the limits for terrestrial life, or be better adapted in other ways to the stresses of the martian surface conditions. It would also have had opportunities for millions of years to colonize any microhabitats there and could colonize them with biofilms or propagules adapted to martian conditions.
This is why it is reasonable for NASA to plan to test returned samples from Jezero crater for present day martian life as a kind of "stretch goal", as we saw in the previous section.
The SSB Review of SR-SAG2 didn't change the classification for Jezero crater which is why it didn't get much attention at the time. But the knowledge gaps it identified do change the picture substantially There is a greatly increased potential for martian life in Jezero crater even though the potential for life there is still considered to be low. A low potential but greater than previously thought.
The SSB Review also changes the planetary protection situation for human landings on Mars. The advances in science it highlighted lead to a picture of Mars where there is a greatly increased risk of forward contamination by a human landing.
NASA's team seem unaware of the contemporary debate in astrobiology journals about forward contamination, focused on how to ensure robotic spacecraft can study potential habitats before they are irreversibly contaminated by terrestrial life from human missions
As we saw, from 2017 to the present, one of the most vigorous debates on the topic of forward contamination in the astrobiology journals is about how much astrobiology can be done before humans get to Mars. See above:
Let's look a bit closer at this debate, which spans numerous articles in astrobiology journals. It is based on the premise that it is likely that after a human landing on Mars, the human caused forwards contamination of Mars can't be localized to a human landing site, and is irreversible.
We will go into this in more detail later in this book when we discuss forwards contamination issues more. However it seems appropriate to cover it briefly right now because of the relevance of discussions of the potential habitability of Mars for terrestrial life, and so also, for martian life which may be better adapted to the conditions there.
This debate started in 2017 with a controversial article in Astrobiology saying we should drop all planetary protection in the forwards direction in order to get our rovers to the most interesting areas for life on Mars before the far more serious contamination from human missions gets to them (Fairén et al., 2017. Searching for life on Mars before it is too late). This got a reply published simultaneously on the same day, 1st October by both of NASA's former planetary protection officers saying that it would risk inadvertently finding Earth contamination on Mars instead of native life (Rummel et al., 2017, Four Fallacies and an Oversight: Searching for Martian Life)The debate continues to the present with the articles raising many points on both sides of the debate. The concern for both sides of this debate is that it may no longer possible to find uncontaminated potential habitats on Mars once humans land there. There is also a concern amongst participants in this debate that martian life might go extinct before we can study it.
The reason for this debate is that NASA has not prioritized access to the special regions, for reasons of cost. It would add a cost permission equivalent to the cost of one large science instrument. The advocates for a careful approach say that we need to find the funding for planetary protection somehow to access these regions. This book covers the issue of funding below, with a proposed solution, starting at:
Of course it is possible participants on both sides of this debate are incorrect in their views. However, this is a strong and persistent strand of thought in the modern debate on planetary protection published in the highest ranked astrobiology journals and so needs to be considered.
One would never guess such a debate was going on from the PEIS. It is another example of how the Mars PEIS team seems from the evidence in the PEIS itself and their replies to public comments to be out of touch with the contemporary planetary protection literature.
I didn't think to mention this debate in my comments on the draft PEIS, so I don't have an official reply on the topic from the PEIS so have no way to know if the team are aware of the debate or not, or why they don't mention it if they are aware of it. However it seems unlikely they can be aware of this debate because of its obvious relevance to the PEIS, the need to mention it if they did know about it, and the many other indications we've seen of a lack of familiarity with many details of the planetary protection literature.
The debate is currently phrased as a question about how much science we can do before the martian anthropocene, i.e. the point after which Mars is no longer in its original unmodified state but is irreversibly biologically affected on a global scale by human astronauts landing there. I cover this below in:
Both sides in the debate are in agreement that human astronauts on Mars potentially could start a martian anthropocene.
However, as we mentioned, NASA scientist Chris McKay is still of the view that Mars can be explored reversibly with robots and even after a human expedition.
Chris McKay's view is consistent with the data so far. It is something we can resolve but it likely needs further studies on Mars to find out who is correct here.
The knowledge gaps identified by the SSB review also make it harder, though not impossible to reverse contamination after a human landing. Some of the microbes would end up in micropores, fractures, microcaves and potentially also caves that the human astronauts hadn't noticed such as erosion or fracture caves with small hard to detect surface openings. Also the biofilms would be harder to sterilize than individual microbes, so reversing contamination may be harder than he envisioned. I cover these potential issues here, and potential ways to address them, if the surface of Mars does turn out to be inhospitable enough for a biologically reversible human landing.
If Chris McKay is right, the chance of life in Jezero crater is likely very low. But in light of the knowledge gaps identified by the SSB review, and the contemporary discussion of the potential for a martian anthropocene, we can't say it is impossible. Not without further research to fill those knowledge gaps.
So we do need to look into whether microbial life from Mars could harm us.
Gaia, Swansong Gaia, or Anti-Gaia? How Mars's thin atmosphere near the triple point of water could be a weak biosignature of a Swansong Gaia stabilized through photosynthesis to keep Mars just barely habitable
If there is no life on Mars then we have nothing to worry about for returned life. The scenario of a lifeless Mars also makes Mars much less interesting for astrobiology, of less commercial interest to humanity too, and reduces its interest as a destination to explore. Then, even in the lifeless Mars scenario we do need to consider carefully if we want to start the anthropocene on Mars. We cover this below in:
- How introducing microbes in the wrong order in an accidental anthropocene could harm human prospects on Mars
- Mars’ potential uninhabited habitats as precious resources, the only nearby analogues of early life, prebiotic or abiotic chemistry on a terrestrial planet, vulnerable to contamination by terrestrial life
However we don't know that Mars is uninhabited. Given that Mars is certainly only barely habitable if habitable at all, can we deduce from that already that it is very likely to be uninhabited?
Some would say yes. There are three main lines of reasoning here.
First, based on Lovelock's Gaia hypothesis reasoning that if there was life on Mars it would have made it more habitable for itself than the apparently lifeless Mars we see today. He reasoned that it would adapt the atmosphere away from chemical equilibrium to be optimal for growth. It should have put the atmosphere out of balance and made the planet far more habitable for itself. If we can't spot life easily today, it's likely not there at all.
With this reasoning life could alternatively still be there but below the surface in habitats not connected to the surface since Lovelock's reasoning only applies to a biosphere that we can observe. A subsurface biosphere could be well out of chemical equilibrium but impossible to detect yet.
Second is the idea of an "anti-Gaia". Life on Mars could have pushed the planet rapidly into an uninhabitable for itself. With this reasoning, even if there are habitats today, life would have made itself extinct long ago. We would expect life to form a Gaia or an anti-Gaia on early Mars. If it did form a significantly more habitable Gaia, it hasn't survived to the present, so both possibilities seem to come to the conclusion that Mars is likely lifeless today at least on the surface.
Third, there's a simpler line of reasoning that can also lead to a view of a currently uninhabited Mars, or one only inhabited well below the surface. It's unlikely that the current barely habitable Mars is at its least habitable. By random variation in the amount of CO2 delivered by volcanoes or comets, there is near certainty that it had times in the past where it was even less habitable, and likely completely uninhabitable. So there almost certainly were times in the past when any surface life went extinct. With these ideas we'd only find life on Mars today if it can evolve from scratch very fast, or perhaps if the surface can be recolonized from a subsurface hidden biosphere.
With all these ideas it's possible Mars has habitats today but if so, they most likely are uninhabited habitats. There may be a small possibility that some could have been recolonized from below the surface in the recent past few million years.
Based on an of these three lines of reasoning, there seem to be many reasons to expect Mars to be uninhabited and the chance of any life on the surface seems low. If it has habitats they seem likely to be uninhabited habitats.
However there is a fourth line of reasoning which we can find in the literature but rarely discussed, that may lead us in the other direction. The Martian atmosphere is intriguingly very close to the triple point of water. Could that be more than coincidence? It's undoubtedly been warmer in the past at times with water flowing, but perhaps there might be some mechanism in play to stop it drying out completely?
Several authors have suggested abiotic feedback processes that could prevent Mars drying out completely. In this book we will introduce a new idea that strengthens this fourth approach. The new idea we will look at here is that biology could also prevent Mars from drying out completely. That leads to the idea of a "Swansong Gaia". This is the idea that Mars entered a "Swansong" phase of a dying ecosystem as the planet became less habitable, but then it remained in that Swansong phase because of biological feedback processes that kept it habitable but only marginally so at close to the triple point of water. In that way it could maintain low levels of life indefinitely for the last 3 billion years or more.
The word "Swansong" here is a commonly used phrase in English for the last work of an author, or composer, and is based on the ancient Greek legend that swans sing only once and beautifully just before they die (Nelson, 2019, "Most musicall, most melancholy": Avian aesthetics of lament in Greek and Roman elegy : IV. The Elegiac Swan). O'Malley-James coined the term "Swansong biosphere" to refer to the phase of a planet's history when a once very habitable planet is still marginally habitable but is gradually becoming less habitable before life is finally extinguished. The swansong phase for Mars started billions of years ago as it cooled down (O'Malley-James et al., 2013. Swansong biospheres: refuges for life and novel microbial biospheres on terrestrial planets near the end of their habitable lifetimes) (O'Malley-James et al., 2014. Swansong biospheres II: The final signs of life on terrestrial planets near the end of their habitable lifetimes). Combining "Swansong" with "Gaia" seems a natural term to use for a biosphere that persists in its barely habitable "Swansong" state for billions of years.
So let's look at all these ideas, Gaia, anti-Gaia, intermittently habitable Mars, an abiotic indefinite Swansong, and a life based Swansong Gaia in more detail.
James Lovelock with his Gaia hypothesis suggested that life makes a planet more habitable for itself, changing conditions to those optimal for its needs, maximizing the probability for growth.
In this, the Gaia hypothesis, the biosphere is seen as a cybernetic control system able to adapt the Earth environment to an optimum for its needs. In the hypothesis this optimization applies not only to atmospheric abundance but also to the climate, the surface pH, and other important planetary properties
(Lovelock, 1975. Thermodynamics and the recognition of alien biospheres).
Also:
We review the evidence that the Earth's atmosphere is regulated by life on the surface so that the probability of growth of the entire biosphere is maximized.
…
We believe that Gaia is a complex entity involving the earth's atmosphere, biosphere, oceans and soil. The totality constitutes a feedback or cybernetic system which seeks an optimal physical and chemical environment for the biota.(Margulis et al., 1974. Biological modulation of the Earth's atmosphere).
He suggested life is able to make a planet more habitable by controlling various parameters such a the surface temperatures, atmospheric composition, for instance photosynthetic life cools down Earth by removing CO2 to prevent it becoming too hot, converting it to oxygen which is a reactive gas which would be lost from our atmosphere over timescales of tens of thousands of years without life. So life makes our planet more habitable by pushing it well out of the equilibrium state it would be in without life.
Lovelock compared this with the equilibrium or close to equilibrium states of the Mars and Venus atmospheres and suggests these are representative of lifeless planets (Lovelock et al., 1974. Atmospheric homeostasis by and for the biosphere: the Gaia hypothesis). He proposed this as a "life-detection” method for alien biosphere
It might be that life was too sparse or existed below the planetary surface, in either case neither it nor its effects might be visible. There are reasons for believing, however, that once life is initiated on a planet it can only persist if it is able to control the planetary environment. This ability may be an important evolutionary step in the early stages of life.
…
If it is a property of biospheres to optimize their use of raw material and free energy to control the planetary surface conditions at those most favourable for survival then this form of biosphere or an alien chemical one should be recognizable. Except by a purposeful act of camouflage any life system will reveal its presence through the chemical disequilibria caused by its contrivances.
(Lovelock, 1975. Thermodynamics and the recognition of alien biospheres)
This Gaia hypothesis was influential at the time of the two Viking lander missions to Mars with the first and only life detection experiments ever done on Mars. Lovelock's hypothesis was published two years before the Viking landing and the Viking landers discovered what seemed to be a lifeless Mars though with a confused signal because of the controversial results from the Viking labeled release experiment. All the other Viking results were as expected for the Gaia hypothesis, showing an atmosphere in equilibrium with no signs of any modification by life, suggesting Mars not only was lifeless but never had life.
Even with Lovelock's hypothesis, there are limits to the capabilities of a planetary Gaia, for instance in the far future, Earth will become too hot for life, as the sun gets warmer, hundreds of millions to billions of years from now. Similarly, Mars could have had a planetary Gaia early on and lost life as a result of getting colder.
However Mars does still have some atmosphere. The CO2 from the original Martian atmosphere would have disappeared long ago if it weren't for emissions from volcanoes. As far as we can tell all the Martian volcanoes are currently geologically completely inactive, or are active at such a low level we haven’t yet detected any signs even of fumaroles or other minor forms of activity. However, they were active in the very recent geological past, with some evidence of localized explosive steam based volcanism as recently as 53 to 210 thousand years ago (Horvath et al., 2021. Evidence for geologically recent explosive volcanism in Elysium Planitia).
Mars also gets CO2 from infall of organics from meteorites (Frantseva at al., 2018. Delivery of organics to Mars through asteroid and comet impacts) (Goetz et al., 2016. MOMA: the challenge to search for organics and biosignatures on Mars. International Journal of Astrobiology). These organics will react with superoxygenated surface layers, and ionizing radiation will also split the surface organics back to gases such as methane and carbon dioxide and water vapour. Mars gets more CO2 from comets, directly or as a result of methane and organics in the comets reacting with the surface organics.
So Mars does still have emissions of warming gases and infall, but none of these are continuous supplies as far as we can tell. Sometimes Mars will get more and sometimes less CO2. Its habitability will also vary depending on other factors like the tilt of its axis and how circular or elliptical its orbit is.
If that was the complete story, it would seem Mars must surely go through times with less CO2 than today unless we see it by remarkable coincidence at its least habitable. For instance, starting from the present, if Mars continues to remain volcanically inactive for tens or hundreds of millions of years, more CO2 will gradually be removed until the pressure falls below the triple point of water. Eventually, even the atmospheric pressure at low altitudes like the floor of the Hellas basin will be so low that the Martian surface is completely inhospitable to life.
This simple argument, that we are unlikely to see Mars at its least habitable, suggests that though we have clear evidence that water flows and forms lakes on Mars sometimes, at other times the Martian surface is likely to become completely inhospitable to life.
So this leads to the modern picture of Mars that it might have had life early on, but it might never have been able to set up a resilient enough Gaia to survive to the present. So Mars could have been inhabited early on and uninhabited later or sporadically sometimes inhabited and sometimes not inhabited with life evolving anew each time. Or it could have life but only below the surface. With this picture, any surface life there today had to get to the surface from below, or evolve anew over the last few millions or tens or hundreds of millions of years since whenever the surface was last completely uninhabitable. Charles Cockell explores some of these scenarios (Cockell, 2012, Trajectories of martian habitability : fig. 6).
With this picture of a swansong phase of an early rich Gaia biosphere that rapidly became less habitable, It would be quite a coincidence for Mars to continue as a barely habitable swansong biosphere over 3 billion years after it last had a thick enough atmosphere for oceans.
However we get a different picture if we look at how Gaia cycles would work on Mars in more detail. The same cycles that keep Earth close to the optimal conditions for life, over a very wide range of CO2. emission scenarios have the opposite effect on Mars. Photosynthetic life would reduce the CO2. in the atmosphere by sequestering it as carbonates. There seems to be no way for it to return back to the atmosphere, because of the lack of continental drift.
That would seem to make things even worse for present day habitability. Kleidon coined the term “anti Gaia” for life that makes a planet less habitable for itself in a continuous feedback ending up with an abiotic planet (Kleidon, 2002. Testing the effect of life on Earth's functioning: how Gaian is the Earth system?).
However there is one curiosity about the martian atmosphere that may give one pause for thought. Mars is indeed barely habitable but its atmosphere is almost exactly at the triple point of water, the combination of temperature, and pressure, that lets water exist in either its solid, liquid or gaseous phase, at a temperature of around 0°C and a pressure of a little under a hundredth of Earth's atmospheric pressure.
Mars could be far less habitable than this. It's because of this almost but perhaps not quite completely uninhabitable state that Mars has such a diverse potential for microhabitats. See the section above:
The Martian atmospheric pressure at 0°C is remarkably close to 6.1 millibars, the triple point of water, the balance of temperature and pressure where water can exist as ice, water vapour or liquid water. Low lying regions have pressures high enough for water to be stable briefly for a few hours at some times in the year. Meanwhile the pressures at the mountain tops are so low that liquid water in any form, even the brines, is likely impossible. In Hellas basin the atmospheric pressure by one model is 12.4 millibars, so water would boil at 10°C (NASA, 2000, Making a Splash on Mars).
At present it is because of a physical process that keeps the atmospheric pressure at 6 millibars which rapidly restores the atmosphere to the current 6 millibars by depositing dry ice at the poles, whenever it goes above that pressure. However most of the time, at times of higher axial tilt, the dry ice sublimes completely and raises the atmospheric pressure to 12.5 millibars which is the current maximum achievable with a runaway greenhouse effect (Bierson et al, 2016, Stratigraphy and evolution of the buried CO2 deposit in the Martian south polar cap : 4176). That is enough to have liquid water in many more locations but is still barely habitable.
When the axial tilt reaches 35 degrees this also leads to large deposits of ice condensing on the regolith down to within 30 degrees of the equator. (Head et al., 2004. Recent ice ages on Mars). The atmosphere then becomes briefly thick enough for running water to flow at times.
There is evidence that some of the gullies might have formed from a mix of dry ice evaporating and water flowing. This may have happened as recently as 630,000 years ago (Dickson et al., 2023. Gullies on Mars could have formed by melting of water ice during periods of high obliquity).
As professor Jim Head put it:
“We know from a lot of our research and other people's research that early on in Mars history, there was running water on the surface with valley networks and lakes. But about 3 billion years ago, all of that liquid water was lost, and Mars became what we call a hyper-arid or polar desert. We show here that even after that and in the recent past, when Mars’ axis tilts to 35 degrees, it heats up sufficiently to melt snow and ice, bringing liquid water back until temperatures drop and it freezes again.”
( Siliezar, 2023. Gullies on Mars could have been formed by recent periods of liquid meltwater, study suggests)
It would be a remarkable coincidence to observe the atmosphere of Mars in this state, if the atmospheric pressure on Mars randomly fluctuates, depending on infall from space and volcanic emissions. Wouldn’t such low levels persist only for a short while after a pulse of carbon dioxide, either as infall from comets and meteorites, or emissions from volcanoes. Wouldn’t Mars most often have either no carbon dioxide at all, or far more than just 12 millibars when the atmosphere is fully inflated at high axial tilts?
Suppose it is more than a coincidence?
This observation of an atmosphere so close to the triple point of water has lead to some authors proposing that there is some process removing CO2 from the atmosphere. If this process involves liquid water, it could explain how the atmosphere has stayed so close to the triple point of water. Nolan has suggested that the Mars atmosphere could stabilize at around the triple point of water, because the water enhances carbonate formation. He suggests that it doesn't go far below this point because as Mars gets drier, the amount of carbon dioxide it can remove from the atmosphere falls greatly (Nolan, 2008. Mars: A cosmic stepping stone. In MARS A Cosmic Stepping Stone : 137). Kahn and Haberle et al. have a similar suggestion,with abiotic photosynthesis as another process with the same potential effect of keeping the atmosphere close to the triple point of water (Kahn, 1985. The evolution of CO2 on Mars) (Haberle et al., 2001. On the possibility of liquid water on present ‐ day Mars).
Mars can lose CO2 in at least two main ways.
Mars can lose its CO2. to interplanetary space through carbon dioxide sputtering in the upper atmosphere. This is not going to be sensitive to atmospheric pressure.
However, it can also lose CO2.by dissolving into water and forming carbonates (Hu et al., 2015. Tracing the fate of carbon and the atmospheric evolution of Mars). The rate of loss through carbonate formation could be sensitive to the presence of liquid water. If the atmosphere increases above the triple point for water as a result of volcanic outgassing, this leads to liquid water forming on the surface of Mars, either as open springs, rivers and lakes, or as less obvious short term pockets of water or moisture in the soil. This increases the rate of carbonate formation through carbon dioxide dissolving in the water. Then as CO2 is removed, the atmosphere thins, and with hardly any water left, the rate of carbonate formation drops almost to zero. At that point the atmosphere stabilizes, and it never goes all the way to a vacuum like the Moon, so long as there is enough CO2 still emitted to keep the atmosphere from vanishing altogether.
There is some evidence that this process is going on in present day Mars. It has formed significant quantities of carbonates, which we find in analyses of the Martian dust. From thermal infrared spectra, , the dust contains 2-5% of carbonates by weight. Scaled up to the surface of Mars, that is enough to sequester several times Earth's atmospheric pressure (several bars) of CO2 atmosphere (Bandfield et al., 2003. Spectroscopic identification of carbonate minerals in the Martian dust) (Niles et al., 2013. Geochemistry of carbonates on Mars: implications for climate history and nature of aqueous environments : section 3.1). This calculation just accounts for carbonates in the dust. There are more carbonates in surface rocks (Niles et al., 2013. Geochemistry of carbonates on Mars: implications for climate history and nature of aqueous environments : section 3.2.4), and yet more detected in the subsurface through study of Martian meteorites (Niles et al., 2013. Geochemistry of carbonates on Mars: implications for climate history and nature of aqueous environments : section 2). So Mars has lost enough CO2 to the carbonates to maintain a far more habitable Mars if the CO2 could somehow be returned to the atmosphere .
We could examine the chemical composition of carbonates in the dust to help reveal when they were formed. Bandfield et al. say we can expect to find a mix of magnesium and calcium carbonates and oxides if they formed in a dry and thin atmosphere like the present day climate on Mars, while we can expect to find calcium carbonates associated with clays (symmetries clays) if the weathering occurred in wetter conditions with a thicker CO2 atmosphere (Bandfield et al., 2003. Spectroscopic identification of carbonate minerals in the Martian dust).
Curiosity has found some evidence suggesting abiotic photosynthesis on Mars.
Both δ13C and δ18O values predicted by analogy to terrestrial photosynthetic processes fall within the range of values measured by SAM, supporting abiotic photosynthesis as a potential source of compounds evolving CO2 at low and medium temperatures.
...
It is feasible that both abiotic photosynthesis and delivery of exogenous material are ongoing on modern Mars, actively replenishing the surface with small quantities of organic compounds. Abiotic photosynthesis could also yield small amounts of methane and contribute to the variability in background methane detected by TLS (see Supplementary Information).
(Franz et al., 2020. Indigenous and exogenous organics and surface–atmosphere cycling inferred from carbon and oxygen isotopes at Gale crater)
These researchers have proposed that over a wide range of emissions scenarios for Martian volcanoes, and for most of the geological history of a Mars-like planet, we expect to see its atmosphere stabilized close to the triple point of water. This abiotic feedback, process slows down carbonate formation and fixing of organics when the atmosphere thins and the water dries up. This could extend the swansong phase of a Mars-like planet for billions of years and perhaps almost indefinitely.
So in this abiotic scenario, which doesn't involve life processes, a smaller planet like Mars with no plate tectonics would be likely to reach its swansong biosphere phase early on, as described by O'Malley Jones (O'Malley-James et al., 2013. Swansong biospheres: refuges for life and novel microbial biospheres on terrestrial planets near the end of their habitable lifetimes) , as it wouldn’t have enough volcanism to maintain a thick atmosphere, losing its atmosphere to carbonates which then can't be cycled back to the atmosphere in volcanoes as they are on Earth. However, by this hypothesis even such a small planet with no plate tectonics might have enough volcanism to extend its swansong phase for billions of years, because of the reduced levels of sequestration of CO2 in such dry conditions.
So what if we introduce the idea of biotic photosynthesis in addition to the abiotic photosynthesis of Kahn and Haberle et al?(Kahn, 1985. The evolution of CO2 on Mars) (Haberle et al., 2001. On the possibility of liquid water on present ‐ day Mars). That leads to the idea of a Swansong Gaia where life itself is actively involved in maintaining the Swansong biosphere phase.
For as long as there is abundant water, carbonate formation removes CO2 from the atmosphere. But that would continue only until it is reduced to the minimum that still lets life persist, which is what we observe, around the triple point of water.
If CO2 emissions and infall stop completely, eventually over long periods of time, even the small amount of life left uses up the CO2 for as long as the planet is even marginally habitable, then some of it is sequestered by abiotic processes,, and some of it is lost to space and the planet becomes uninhabitable.
But now suppose there is enough input of CO2 from volcanoes to maintain the atmosphere at a barely habitable level even when the volcanoes are at their least active. When volcanoes become more active they add a pulse of CO2 and the planet briefly becomes more habitable with liquid water, but then the photosynthetic life rapidly removes it, restoring the barely habitable conditions close to the triple point of water that we see today.
Just as for the abiotic processes, photosynthetic life would be far more effective at removing CO2 when there is lots of water and the water is warmer. As the CO2 is removed this cools down the planet, the water turns to ice, migrates to the ice caps or is buried underground, until there is hardly any water left on the surface. At that point just as for the abiotic processes the feedback slows down and almost stops and the atmosphere stabilizes close to the triple point of water.
This Swansong Gaia works in the same way as the abiotic prolonged Swansong - but it can amplify the effect, and so can maintain a swansong biosphere for billions of yeas over a much wider range of emissions scenarios for the volcanoes. In this model, if life had never evolved on Mars, and depending on how much CO2 and H2 the volcanoes emit, it could have a much thicker atmosphere. Depending on volcanic emission rates and depending on the efficiency of the abiotic sequestration processes, it might even have a one bar atmosphere or more still, and might even still have water in the form of lakes, and rivers, maybe even small oceans surviving to the present.
So with Swansong Gaia, life can make a planet much less habitable but unlike the anti-Gaia hypothesis it remains barely habitable for billions of years.
Text on Graphics: Swansong Gaia hypothesis.
Modern Mars looks sterile, but photosynthetic life might take CO2 out of the atmosphere when it gets warm enough for liquid water, keeping Mars barely habitable.
This would work with a wide range of CO2 emission scenariosEarly Mars with water and possibly early life
Image credits: NASA’s Goddard Space Flight Center from: (Steigerwald, 2019, New Insight into How Much Atmosphere Mars Lost )
This same cycle helps to keep Earth close to its most habitable state. Photosynthetic life (including cyanobacteria, and forams which photosynthesize in symbiosis with unicellular algae) creates a carbon sink which helps keep the planet from getting too warm (Richardson, 2019. Mechanisms and pathways of small-phytoplankton export from the surface ocean). This keeps Earth in a very habitable state by converting most of the CO2 to oxygen, reducing the greenhouse warming. Earth can maintain such high habitability because the process of continental drift and plate tectonics constantly returns carbonates and buried organics to the surface in volcanic eruptions.
However even Earth's Gaia is not necessarily optimally habitable. Earth was significantly less habitable during the ice ages. Also in the opposite direction, if the continents were warm enough to be covered in forests everywhere with no ice caps or tundra, it would increase biomass, and the amount of carbon sequestered in the biosphere (Kleidon, 2002. Testing the effect of life on Earth's functioning: how Gaian is the Earth system?). Schulze-Makuch et al reason that the highest biomass is in tropical rainforests and by analogy with the carboniferous era it’s possible a 5°C warmer Earth could sustain more biomass depending on which organisms inhabit it (Schulze-Makuch et al., 2020. In search for a planet better than Earth: Top contenders for a superhabitable world: 1397).
We see already for Earth that life doesn’t necessarily make a planet optimally habitable. On an already cold Mars-like planet with a thin atmosphere, with no tectonic drift to return CO2 to the atmosphere via volcanoes, cooling down is the opposite of what is needed for life.
Cyanobacteria precipitate carbonates both internally and externally (Benzerara et al., 2014. Intracellular Ca-carbonate biomineralization is widespread in cyanobacteria). They also sequester some of it as organics, and though secondary consumers of the photosynthetic life might return some of the CO2 to the atmosphere, they are not likely to return all of it. Some organics and carbonates are likely to accumulate, as they do on Earth, however, on Mars there are no tectonic processes to return them to the atmosphere.
Our rovers on Mars have photographed many structures that some experts on similar terrestrial fossils say could be algal mats and stromatolites, though at present it is not yet possible to distinguish them reliably as formed by biological rather than non life processes, especially in the unfamiliar context of the martian environment. Nora Nofke who studies MISS, acronym for "Microbially Induced Sedimentary Structures" has found many potential candidates on Mars that to her expert eye look like the structures she studies on Earth and are distinctively different from other geological structures . This is an article about her work from 2015, (Bontemps, 2015, Follow Up - Signs of Ancient Life in Mars Photos?). Other experts at the time said they didn't see anything that couldn't be explained as erosional processes, but hadn't looked at the structures as closely as she had. She has published her results in the Astrobiology Journal in a paper from 2021 where she doesn't conclude that they are produced by life but presents evidence they might be, saying in the abstract " MISS serve as possible templates for the
decryption of ancient life-processes on Mars." (Nofke, 2021,
Microbially Induced Sedimentary Structures in Elastic Deposits: Implication for the Prospection for Fossil Life on Mars)
This takes careful work as the conditions are so different on Mars, with the lower gravity, the thin atmosphere, light winds, the surface chemistry is different and so on. We have to use the Knoll criteria to evaluate them. It's not enough that they look like fossils.
"The Knoll criterion is that anything being put forward as a fossil must not only look like something that was once alive -- it must also not look like anything that can be made by non-biological means.”
Oliver Morton, author of (Morton, 2003, Mapping Mars: Science, Imagination, and the Birth of a World) as interviewed by James Oberg in: (Oberg, 2004, avoiding the F word on Mars)
James Oberg explains that this criterion is named after Andrew Knoll, author of (Knoll, 2003, “Life on a Young Planet"), a book about past Earth life, who is on the Curiosity mission science team.
For this section we are especially interested in life that could have sequestered carbonates, such as algal mats or stromatolites and other structures produced by algae.
Our rovers on Mars have found many intriguing structures for experts to look at. These white curving lines are an example of several structures interpreted as possibly fossils produced by early martian algae in a paper published by Vincenzo Rizzo from the National Research Center in Cosenza, Italy, in the International Journal of Astrobiology. (Rizzo, 2020, Why should geological criteria used on Earth not be valid also for Mars? Evidence of possible microbialites and algae in extinct Martian lakes).
This shows a detailed photo with Curiosity's equivalent of a geologists's hand lens, Mars Hand Lens Imager (MAHLI) camera. it shows elongated white curved structures which Rizzo interprets as fossil structures formed by ancient life
To his expert eye these closely resemble encrusted filaments of blue-green algae and the author sees a resemblance specifically to structures produced by the cyanobacteria Cyanophyta as well as structures produced by green algae. They don't look like natural rock structures.
"At first, they may resemble plagioclase phenocrysts [large naturally formed crystals of an aluminium bearing mineral] in basaltic or trachyte lavas. However, phenocrysts typically exhibit more tabular and straight crystal surfaces, while such curved spots are more similar to certain microbiological settings like encrusted filaments of blue-green algae (the arrow shows one of these curved spots). In particular, they are reminiscent of Cyanophyta or of certain green algae, such as the mm-sized fragments of Gymnocodiaceae or some cisted forms of Euglenaceae"
(Rizzo, 2020, Why should geological criteria used on Earth not be valid also for Mars? Evidence of possible microbialites and algae in extinct Martian lakes : figure 3)
The astrobiologist Dirk Shultze Makuch says about Rizzo's work:
In principle I’m very skeptical of any evidence based only on visual resemblance or morphology, because the human brain has the tendency to see or fill in familiar patterns, even where they don’t quite exist. Rizzo goes well beyond appearances with his analysis, however. He made me realize that if—and it’s a big if—stromatolites did in fact exist on early Mars, they would probably look like the specimens he found in the rover images.
Carl Sagan’s saying, “Extraordinary claims require extraordinary evidence,” comes to mind. For every structure produced by biological processes, there might be some geochemical or physical process that mimics it. Some of these processes may be unknown to us on an alien planet such as Mars.
...
What, then, is the more “extraordinary” claim? That Mars during its early habitable period had life forms similar to those on Earth, which produced similar biogenic structures? Or that Mars is and always has been a dead planet?
Still, the bar for claiming to have detected life on Mars, past or present, has to be set high. Even on Earth it is sometimes difficult to decide whether a particular structure is the result of biology. Even though our ability to explore Mars has improved, we’re still not able to do what we routinely do on Earth—go out with a hand lens or other tool and study some enigmatic feature.
(Schulze-Makuch, 2020, The Case for Past Life on Mars Gets Stronger)
Rizzo also found curved laminations which are hard to explain by other methods. They resemble the annual layers built up by stromatolites. It is hard to get such small scale continuously curved layers from wind blown deposits, which tend to have sharp discontinuities when the wind changes direction, rather than small scale smooth curves. Mars had no plate tectonics, to bend the rocks after they formed. They form as curved structures on top of flat lake sediments so can't be explained as lake sediments. They are exactly what one would expect from domed structures built up by algae in shallow water. Rizzo says they closely resemble the terrestrial stromatolite Conophyton (Rizzo, 2020, Why should geological criteria used on Earth not be valid also for Mars? Evidence of possible microbialites and algae in extinct Martian lakes: f288-9).
Curved millimeter scale laminations - the white bar is one centimeter. It is hard to explain smooth curves at this scale based on either lake sediment or wind while they are natural shapes to form from layers of algae building on top of each other in a shallow lake.
(Rizzo, 2020, Why should geological criteria used on Earth not be valid also for Mars? Evidence of possible microbialites and algae in extinct Martian lakes : Figure 5)
These structures also resemble micro-atolls which form in shallow water when there's a diverse community exchanging nutrients with the most vigorous growth at the edge of the community.
Possible fossil micro-atolls on Mars and compared with a terrestrial microatoll fossil (Rizzo, 2020, Why should geological criteria used on Earth not be valid also for Mars? Evidence of possible microbialites and algae in extinct Martian lakes: figure 1)
image D reproduced under the license Wikimedia Creative Commons (Creator Bahnfrend).
We certainly can't deduce from these structures that Mars did have life. But if it did have life there may be a reasonably good chance that these are fossils of it.
If this is life there may be a significant biological carbon pump in shallow lakes on Mars when the atmosphere is thick enough for liquid water, through formation of stromatolites, algal mats and so on. These photos are from Gale crater where Curiosity landed which had a lake and running water 3 billion years ago. However Mars has been active even in the last few million years which might boost the atmospheric pressure significantly for a while. This book covers some of the evidence for recent volcanic and geothermal activity in the Supplementary information section under:
This biological pump would also sequester some of it as organics, and though secondary consumers of the photosynthetic life might return some of the CO2 to the atmosphere, they are not likely to return all of it. Some organics and carbonates are likely to accumulate, as they do on Earth, however, on Mars there are no tectonic processes to return them to the atmosphere.
Carbonates might also accumulate as protective coverings of calcite if martian life has ever made use of calcite in this way, as for the coccolithophores (single cell shell forming marine organisms which formed deposits such as the White Cliffs of Dover).
Text on graphic: Photosynthetic life on Mars could take carbon dioxide out of the atmosphere by forming carbonates, either precipitated by cyanobacteria or possibly used as a covering as for the coccolithophores
This could cool it down whenever it gets warm enough for photosynthetic life to flourish. Not an anti-Gaia but a Swansong Gaia keeping Mars barely habitable.
Credit Tim Bralower © Penn State University licensed under CC BY-NC-SA 4.0
(NASA, n.d.,Marine Biota Exchange — The Biologic Pump).
Terrestrial life also includes forams which similarly produce protective calcite cells and are responsible for removing 2.9 gigatons a year of the terrestrial carbon dioxide (Jacob et al., 2017. Planktic foraminifera form their shells via metastable carbonate phases).
If life did evolve on Mars, it may well have evolved similar calcite shells in some form.
In this scenario, Mars remains barely habitable by rapidly sequestering the CO2 from volcanoes as organics and carbonates whenever the atmosphere bulks up to be warm enough for life to flourish. We already have good evidence for the carbonates as we saw with the abiotic hypothesis. The suggestion is that some of them may be produced by life processes.
If Mars ever had microbial life that formed calcite shells like the coccolithophores, it would be easy to spot as soon as we can look at it with a microscope. However, so far we have only sent the equivalent of geological hand lenses to Mars. Spirit and Opportunity had cameras with pixel size of 31 microns and resolution of about 100 microns (NASA, n.d., MI). Curiosity improved on that with pixel size of 12.5 microns (NASA, n.d., MAHLI) while Perseverance's resolution is between the two (Edgett et al., 2019, Mars 2020 Perseverance SHERLOC WATSON Camera Pre-delivery Characterization and Calibration Report : 31).
These carbonates wouldn't need to be in the form of easily recognizable fossils. But because we haven't yet sent a microscope to Mars able to detect microfossils, we wouldn't know yet, even if Mars has calcite fossils as easy to spot as the coccolithophoers as well preserved microbial fossils in the carbonate deposits.
Carbon dioxide isn't the only gas that can get involved in a Swansong Gaia. We need to look at other gases like nitrogen, hydrogen and methane. We also need to look at what happens to the organics and the oxygen produced by photosynthesis.
Boris Sauterney headed a team that modeled a simple biosphere for early Mars based on a hydrogen / methane biological cycle. Their results at first sight seem to support a strong form of the anti-Gaia hypothesis for Mars but on closer examination it turns out their model may be able to provide a basis for another form of Swansong Gaia.
He describes it as an anti-Gaia, as a potentially self destructive tendency for life:
“Basically what we say is that life, when it appears on the planet and in the right condition, might be self-destructive. It’s that self-destructive tendency which might be limiting the ability of life to emerge widely in the universe.”
(Gaspirini, 2022, Life on Mars May Have Been Its Own Worst Enemy)
In this model, on early Mars as on early Earth, hydrogen is produced from volcanoes and then life turns it into methane, Details in (Sauterney, 2021, Co-evolution of primitive methane-cycling ecosystems and early Earth’s atmosphere and climate : Figure 4e).
In their previous model for early Earth these same reactions help make Earth warmer because methane is a warming gas when introduced into a simulated early terrestrial atmosphere of nitrogen at 1 bar (i.e. the same as modern atmospheric pressure). In this nitrogen rich atmosphere, hydrogen isn't a warming gas. They found that for early Mars, methane warms the atmosphere slightly by 4°C (Sauterney, 2021, Co-evolution of primitive methane-cycling ecosystems and early Earth’s atmosphere and climate : Figure 4e).
However it's the other way around on Mars. A CO2 atmosphere turns hydrogen into a much stronger greenhouse gas than methane (Sauterney, 2022, Early Mars habitability and global cooling by H2-based methanogens : 6). That's because of collision effects of the carbon dioxide with the hydrogen
"On ancient Mars, hydrogen was a very potent warming gas because of something we call the collision-induced absorption effect where molecules of carbon dioxide and hydrogen interact with each other,"
(Pultarova, 2022, Ancient Mars microbes triggered climate change that made it hard for them to survive).
In their model biosphere, methanogens rapidly make early Mars -33°C to -45°C cooler
While hydrogen methanogens may have contributed to maintaining temperate conditions on Earth, they would have cooled the early Martian surface, with a reduction of the maximum possible temperature by 33 to 45 K
(Sauterney, 2022, Early Mars habitability and global cooling by H2-based methanogens : 6)
Sauterey presents this as a counterexample to Lovelock's ideas that life makes a planet more habitable. First he mentions the "Gaian bottleneck" theory that life needs to get off to a quick start to make a planet habitable for itself and perhaps seldom manages to reach that point fast enough (Chopra et al., 2016, The Case for a Gaian Bottleneck: The Biology of Habitability).
He presents their finding as even more pessimistic than that, that life would quickly make the planet uninhabitable for itself rather than habitable.
The Gaia hypothesis has since prompted the emergence of the “Gaian bottleneck” idea. This posits that the universe does not lack the necessary conditions for life, but that when life does appear, it is seldom able to sustain the long-term habitability of its planetary environment.
The findings of our study are even more pessimistic. As shown in the example of Martian methanogenesis, even the simplest life forms can actively jeopardize the habitability of their planetary environment.
(Sauterey, 2022, Mars: could life itself have made the planet uninhabitable? )
This is for a simple biosphere and Sauterey does say it could be different with a mix of more forms of life.
“You can imagine that a more complex, more diversified [Martian] biosphere would not have had the negative effect on planet habitability that just methanogens would have had,”
(Gaspirini, 2022, Life on Mars May Have Been Its Own Worst Enemy)
However with this new idea of a Swansong Gaia, what Sauterey describes could also be understood as an extra mechanism leading to an early forming barely habitable Swansong Gaia. Such a biosphere could have made Mars almost uninhabitable early on, However some life would persist in small ice-free regions at or very close to the surface in the life-atmosphere steady state with relatively weak biological feedback.
At life- atmosphere steady state, the relatively high brines freezing point and relatively weak biological feedback balance out with a large ice coverage yet relatively warm ice-free surface, resulting in methanogenic life being limited to small ice-free regions but at or very close to the surface. With brines freezing at 252 K, Hellas and Isidis Planitiae and Jezero Crater are among the few regions that may remain free of ice, where the median minimum depth of Noachian methanogens would then be within the top few meters
(Sauterey, 2022, Early Mars habitability and global cooling by H2-based methanogens : 6).
As volcanoes add hydrogen to the atmosphere, the methanotrophs remove it and convert it to methane, cooling the atmosphere until it is barely habitable. Then as for photosynthetic life for modern Mars the methanotrophs would reduce to relic populations that have minimal effect on the hydrogen, in the small melting ponds.
So long as there is a continuing input of hydrogen the atmosphere never cools enough to be completely uninhabitable. So, as long as there is enough input of hydrogen into the atmosphere to sustain this feedback, those small ice-free pools or near surface pools would continue indefinitely.
This simple picture for early Mars assumes there are no methanotrophs. Sauterey et al say that later on in the history of early Mars, biological consumption of methane (methanotrophs) could have lead to warming episodes. He suggests this as a possible explanation of the transient warming episodes that seemed to have happened in early Mars. (Sauterey, 2022, Early Mars habitability and global cooling by H2-based methanogens : 6)
So it seems we have two potential mechanisms for a Swansong Gaia. One involving methanogens for early Mars, and a different one involving photosynthesis for a present day Mars.
As Mars gradually loses its internal magnetic field and the early sun warms, the atmosphere would transition from reducing to oxidising, as in all the models of early Mars [ADDCITES], because of the effect of the solar wind splitting the water vapour with hydrogen lost to space, perhaps assisted by photosynthesis sequestering organics and producing oxygen. Hydrogen and methane would no longer last long in the atmosphere, and the warming gases are then carbon dioxide and sulfur dioxide and the biological pump is now photosynthetic life removing carbon dioxide rather than methanogens removing hydrogen. In between there'd be a period with both methanogens and photosynthetic life involved in the biological pump. It wouldn't matter how later photosynthetic life evolved though if it evolved later the early Mars could have liquid water for longer once it transitions to an oxidising atmosphere with a slightly warmer sun.
In both cases we can expect more complex interactions especially if life evolves many different forms of life in a more complex biosphere. For instance, what would be the effect of methane emissions in modern Mars, as the climate warms up after a pulse of CO2? Microbes could produce methane from buried organics, or it could be released from clathrates where it might have been trapped in the past adding a pulse of methane to any CO2 pulse.
Methane would be warming in present day Mars, though not as much as hydrogen. It could help warm the planet more than CO2 alone can do, on the other hand methane is short-lived in the present day oxidising Martian atmosphere and surface chemistry. Also any warming might be counteracted by photosynthetic life spreading as the planet gets more habitable.
We do see methane spikes on present day Mars, so how would this work with a Swansong Gaia and how would it interact with the photosynthetic life?
We also need to look at the nitrogen cycle, if Mars has one, and how that interacts with all this,as life depends on nitrogen, nitrates are very soluble in water, and after an episode of warming leading to streams and lakes briefly, then photosynthetic life might only be able to colonize the water by fixing nitrogen. Is this possible for the low levels of nitrogen in the Martian atmosphere? We'll look into this in the next section.
We'll find that if Mars has nitrogen fixing microbes and also microbial denitrification that behaves as it does in terrestrial dry deserts, this would actually reinforce the Swansong Gaia effect.
All this needs careful modelling. Here we will just make preliminary observations, but we will find that the ideas seem plausible enough to consider in more detail. Photosynthesis could keep the atmosphere in a barely habitable state by removing CO2 after an episode of volcanic eruption. It seems plausible that methane cycles would only briefly add to warming during warming pulses and the nitrogen cycle might, depending on assumptions, reinforce the Swansong Gaia effect.
If life is commonplace in the universe, we might detect Swansong Gaia's on exoplanets. There is of course no way we can detect unambiguous biosignatures of life indirectly on a distant Mars-like exoplanet when we can't even detect it on Mars in our solar system. However we could detect the atmospheric composition of Mars-like planets. In this context Mars-like would mean planets towards the cool edge of their habitable zone that are too small for active plate tectonics. If the Swansong Gaia hypothesis is correct we should find that such planets often have atmospheres close to the triple point of water, more often than we'd expect by chance.
While if life on a Mars-like planet leads to an anti-Gaia rather than a Swansong Gaia it's the other way around. It would be rare to have a planet with an atmosphere similar to present day Mars and the effect of life as an anti-Gaia would be that more than expected numbers of planets have no atmosphere or are well below the triple point of water. There "expected number" would be based on physical modelling once we have a better idea of the population of such planets and typical levels of volcanic activity and so on.
Both of these observations would be potential weak biosignatures for either present day life for the planets near the triple point of water or for past life for planets that are uninhabitable more often than expected.
Finally if there are any feedback processes that can return the warming gases to the surface, mimicking the effects of the volcanic emissions for plate tectonics, we might find Lovelock style Gaias even on a Mars-like planet.
Also once we have a good understanding of emission scenarios and of the abiotic processes on Mars-like planets, we could also hope to distinguish the abiotic swansong biosphere from the Swansong Gaia hypothesis. A few planets might be able to stabilize in conditions similar to Mars using abiotic photosynthesis so long as the emissions levels aren't too high, However, because life can remove far more CO2 far faster, then if the Swansong Gaia hypothesis is true, we can expect planets with a barely habitable atmosphere over a far larger range of emissions scenarios if life evolves on them or they are seeded with life from other planets.
The final proof would be if we can find and study life bearing Mars-like planets. We couldn't detect the life at a distance for a Swansong Gaia. But we might be able to find Mars-like planets with detectable early life (with molecules that can only be produced by life), in the process of transitioning to the Swansong Gaia biosphere stage.
This is very hypothetical at present. It would be some time before we could have evidence for it. But it's an interesting counterbalance to Lovelock's Gaia hypothesis.
This Swansong Gaia hypothesis predicts a Mars very like what we see. Life would be hard to spot, but still present. There would still be some photosynthesis going on which could perhaps explain the oxygen cycles
The main evidence we have in favour of this Swansong Gaia hypothesis is that the atmosphere of Mars is close to the triple point of water. However we do also have other possible signs of it to consider, the observations of methane plumes and also of a seasonal excess of oxygen, which may be due to abiotic processes but could also be due to low levels of life present. Low levels of photosynthesis and methanogenesis are exactly what we would expect from the Swansong Gaia hypothesis.
If we do have a Swansong Gaia on Mars we can also hope eventually to detect life on Mars, though it is likely to occur at low levels and be hard to detect Then we can study the workings of it directly based on those observations.
If the Swansong Gaia hypothesis is true, a Mars-like planet in our solar system with an atmosphere around the triple point of water might even be a weak biosignature suggesting presence of life. It is just a hypothesis but if it is true, it greatly increases the chance that there is life on Mars there today.
This book will expand on these ideas the supplementary information, and also look at potential nitrogen and methane cycles that could reinforce or weaken the Swansong Gaia feedback. See Supplementary information under:
This Swansong Gaia hypothesis is also be relevant to terraforming plans, even if Mars is lifeless, with uninhabited habitats. Perhaps life is rare and never arose on Mars or got introduced here, or it got extinct before it could set up a Swansong Gaia.
In that scenario, if we try to terraform Mars but accidentally set up a Swansong Gaia, we might find that the life we introduce frustrates our end goals. Instead of assisting terraforming, introduced life may work to restore the current conditions of a barely habitable Mars as soon as it can.
If these ideas are valid, we could at least delay a Swansong Gaia if we can choose the order in which we introduce the microbes, though this is probably only possible if humans stay in orbit around Mars during the process of terraforming. For instance if we can delay introducing photosynthetic life until later, and use artificial greenhouse gases to boost the CO2 of the Martian atmosphere early on, the atmosphere may thicken more than if we introduce photosynthetic life immediately at the outset.
Also, even if it is true that photosynthetic life would naturally set up a Swansong Gaia if established on Mars, we can explore to see if there any way to set up an artificial warming Gaia even in a cooler planet like Mars. The main issue is that it has low levels of volcanic emissions and no way to use continental drift to return carbonates to the atmosphere as carbon dioxide. But as we saw it has several bars of carbonates in the dust.
Carbonates are easy to convert to carbon dioxide using acid which could be produced by lime=boring microbes, so maybe there is some potential for a biologically based cycle to restore them to the atmosphere? We look at this in
This cycle will be difficult to get started because there seems to be only 12.5 millibars of CO2 available for a runaway greenhouse effect (Bierson et al, 2016, Stratigraphy and evolution of the buried CO2 deposit in the Martian south polar cap : 4176). It might take megatechnology to liberate the carbon dioxide from the carbonates. But if we do this, it would need to be looked at carefully to make sure that the end result is stable, and does work without any interactions that might frustrate it and re-establish a Swansong Gaia. For details see:
This suggests there may be many complexities to consider if we do attempt to terraform Mars. Amongst those complexities, it is also possible we may need to work to delay a Swansong Gaia or if possible, to avoid creating a Swansong Gaia. See below:
Brief statement of the Swansong Gaia Hypothesis
The Swansong Gaia hypothesis states that life on a terrestrial planet near the cool end of the habitable zone and with low levels of emissions and infall of warming gases will limit itself by taking warming gases out of the atmosphere until the planet is in a barely habitable equilibrium state.
The hypothesis is that a Swansong Gaia can maintain itself in a barely habitable state for billions of years over a very wide range of scenarios for the emissions from volcanoes and infall from comets for the warming gases and a wide range of possible metabolisms and biospheres. After a pulse of infall or emission, the planet would warm up for long enough for life to spread over any oceans or lakes but it would then rapidly remove the warming gases to restore the barely habitable state. The biological pump may weaken to the point where it almost ceases to function at 12.5 millibars with dry ice deposition when Mars' axis has low levels of tilt taking it the rest of the way to the current 6 millibars which is currently maintained at that level by equilibrium with the vapor pressure of the deposits of dry ice at the south pole. For details see:
This might be a common natural end state of a biosphere on a Mars-like planet.
If this hypothesis is correct what we see on Mars, a planet with the atmosphere close to the triple point of water, would actually be a weak biosignature. It suggests a planet where there is life still present, in habitats open to the atmosphere, in equilibrium with the current rate of infall and emission of warming gases, and growing fast if necessary after any warming pulse to cool it down to maintain the current barely habitable state. The rapid cooling of early Mars might suggest that it's had a Swansong Gaia that's persisted for billions of years.
If this hypothesis is correct, it would also suggest any life on Mars would be hard to spot. It would still be present to continue to remove gases contributed by infall and occasional volcanic emissions and hydrothermal vents, but it would be likely to have reduced itself to very low levels with the amount of life present there at whatever levels are needed to remove the current rates of emission or infall of warming gases.
For more on this see Supplementary information under:
Earth's Gaia keeps warm through a constant high input of warming gases from carbonates returned in volcanoes via continental drift, so could we achieve this on Mars using acid from lime-boring microbes instead to liberate carbonates?
With this hypothesis, the original Lovelock weak Gaia then applies to planets with higher levels of infall or emissions of warming gasses. For Earth those high levels of emissions are achieved mainly by the processes of plate tectonics and continental drift returning sequestered carbonates to the atmosphere as carbon dioxide through volcanic emissions. Mars can't achieve high enough levels of emissions for a Lovelock Gaia because it is too small to start the process of plate tectonics to return its sequestered carbonates and organics to the atmosphere as carbon dioxide, or any other warming gases. Chris McKay puts it like this:
On an active planet like the Earth, subduction of ocean sediments at plate boundaries followed by thermal decomposition of carbonates deep below the surface is the primary mechanism for completing the long-term geochemical CO2 cycle .... Mars does not have, nor is there any sign that Mars ever had, crustal dynamics akin to plate tectonics; rather, Mars's features are consistent with a one-plate planet ... Without these processes there appears to be no long-term geological mechanism on Mars to recycle CO2-sink materials back into the atmosphere.
... On Mars, the only feasible way to generate CO2 from carbonates appears to be by acidification. Fortunately, there are some known microorganisms that seem to be able to decompose carbonates (Friedmann et al., 1993). However, while this biological source of CO2 may be of importance in the ultimate functioning of a martian biosphere, it cannot be a source of CO2 as Mars is warmed from its present cold state, which is too dry and cold to support the growth of microorganisms.
(McKay, 2001, The Physics, Biology, and Environmental Ethics of Making Mars Habitable :95-6)
The cite there is to (Friedmann et al., 1993.Terraforming Mars: dissolution of carbonate rocks by cyanobacteria). Friedmann et al. propose using Matteia sp., a lime-boring cyanobacteria isolated from the Negev desert to complete the carbon cycle to return carbon dioxide to the atmosphere.
Perhaps that may be possible to some extent even today. We have found that the Martian surface is somewhat more habitable than it was thought to be in 2001..
Even without plate tectonics, Mars may have enough emissions for a Swansong Gaia because it is large enough to have occasionally active volcanoes even today, supplemented by infall such as from comets and asteroids and possibly also by carbonate dissolving microbes if there are any on Mars.
If this hypothesis is correct and we wish to terraform Mars by establishing an Earth-like Lovelock Gaia on a long timescale, we need to find a way to artificially increase its emissions or infall of warming gases, We could keep Mars warm with artificially produced greenhouse gases. We could also boost infall by diverting comets to hit Mars though that would require a large number of comets, a million at 1 km in diameter (McKay et al., 1991, Making Mars Habitable : 352).
Perhaps we might even be able to set up some biosphere that uses life processes to return carbonates to the atmosphere to substitute for continental drift using lime boring microbes or similar. Perhaps such a system could arise naturally too.
Given the complexity of interactions in a biosphere, and given that there is a potential way for life to return carbon dioxide to the atmosphere, this hypothesis doesn't rule out the possibility that there might be ways to establish a warmer and more productive biosphere on a Mars-like planet that is able to return the warming gases to the atmosphere. If so it may be a matter of chance whether a Mars-like planet establishes a Swansong Gaia early on or a more habitable Gaia warmed by gases somehow returned to the atmosphere by life processes instead of through continental drift and volcanic emissions. However a Swansong Gaia seems to be a possibility and may be strongly favoured and if so that may be what we see on Mars today.
For more on all this see:
Sources of nitrogen on Mars as a potential limiting factor – potential for Martian life to fix nitrogen at 0.2 mbar – and “follow the nitrogen
So far we've focused on water in the discussion of habitability of Mars. Life also needs energy, and trace elements, and it also needs nitrogen.
There are many sources of energy on Mars. The blue-green algae chroococcidiopsis is one of the main candidates for a Mars analogue terrestrial microbe (Billi et al., 2019. Dried biofilms of desert strains of Chroococcidiopsis survived prolonged exposure to space and Mars-like conditions in low Earth orbit). It can use light as a source of energy, and as a prime producer, could survive almost anywhere on Mars with access to liquid water, sunlight, and some protection from UV (Billi et al., 2019. A desert cyanobacterium under simulated Mars-like conditions in low Earth orbit: implications for the habitability of Mars), and access to trace elements and nitrogen.
Alternatively, non photosynthetic life can use chemical redox gradients as a source of energy, for instance surface layers of sand dunes are superoxygenated while the slowly moving sand dunes constantly bring subsurface reducing layers to the surface (Fisk, et al., 2013. Habitability of Transgressing Mars Dunes).
There are many possible electron donors and acceptors for a metabolism. This is a table of some of them.
electron donors, any of: | electron acceptors, any of: |
---|---|
FeSO2+ : available in Fe-rich silicates | Fe3+ : available in numerous alteration minerals |
H2 : available in subsurface? | SO42− : available in salts |
CO : available in atmosphere | O2 : partial pressure too low |
organics : meteoritic, present at surface | NO3− : presence or abundance unknown |
organics: endogenous available in subsurface | ClO4+ : available but not shown to support life |
A candidate metabolism would use one of the electron donors in the first column paired with one of the electron acceptors on the right as a source of energy. (The final dash on left hand side is there just because the list of electron donors is shorter than the list of electron acceptors). Table adapted from (Nixon et al., 2013, Plausible microbial metabolisms on Mars).
The paper also mentions CO2 which is used as an electron acceptor by methanogens with molecular hydrogen as the donor.
As for trace elements for life, basalt is a good source for them all, and it is common on Mars (McMahon et al., 2013. The habitability of vesicles in martian basalt).
This leaves nitrogen. All life on Earth requires nitrogen. Also, there are theoretical reasons for expecting alien organic life to use nitrogen. That's because they are essential for keeping the spiral structure of DNA, RNA and proteins.
For instance in a protein when two amino acids join together as part of a protein they form a peptide bond - CO - NH - ... The hydrogen in the NH then can form a hydrogen bond with the oxygen in the CO in the next turn of the spiral.
Diagram of helical structure of a protein, showing how the nitrogen mediated hydrogen bonds hold it together
For more, see (Schirber, 2013 Searching for Organics in a Nibble of Soil). Diagrams credit J. Bada.
These bonds are also used to join the two strands of DNA together like this:
These bonds are easy to break and restore and are essential to the many functions of DNA where the two strands have to unzip and then zip up again.
Jeff Bada explained it like this, interviewed for an article for NASA’s Astrobiology Magazine . .
“Organic nitrogen compounds are central for biology as we know it. No other class of compounds plays a more important role.”
“Nitrogen atoms in nucleobases and amino acids form hydrogen bonds that stabilize the structure of both nucleic acids and proteins. Without these hydrogen bonds there would be no helical structure to DNA and RNA and no alpha-helix structure for proteins.”[Also the nitrogen-hydrogen bonds are easy to break helping enable the chemical processes of life]
“No other atom than nitrogen can form the diverse set of hydrogen-bonded compounds found to be fundamental in the biologically central processes of replication and catalysis.
(Schirber, 2013 Searching for Organics in a Nibble of Soil)
So it is likely nitrogen is essential to life on Mars if it works similarly to terrestrial life, even if it is based on an independently evolved alien biology. It's certainly essential if Martian life is related to terrestrial life.
Then life needs the nitrogen to be fixed as nitrates and other nitrogen compounds before it can use it. There are some natural abiotic nitrates. However nitrates dissolve readily in water. The largest accumulations of abiotic nitrates are in the Atacama desert where the dry conditions prevent the nitrates getting washed away (Reich et al., 2018. Nitrate deposits of the atacama desert: A marker of long-term hyperaridity).
However, most terrestrial life gets a lot of its nitrogen through nitrogen fixing plants and microbes, for instance legumes fix nitrogen in the soil.
Mars has only 0.2 mbar of nitrogen in the atmosphere, far less than the 780.90 mbar in Earth’s atmosphere.
Is it possible for life to fix nitrogen at such ultra low levels? In laboratory experiments, some cold tolerant microbes from Antarctica are able to fix nitrogen at a partial pressure of 0.2 mbar similarly to the partial pressures on modern Mars, as reported in unpublished research (Mancinelli, 1993, personal communication cited in Thomas, D., 1995, Biological aspects of the ecopoesis and terraformation of Mars: Current perspectives and research) following (Klingler et al., 1989. Biological nitrogen fixation under primordial Martian partial pressures of dinitrogen). Sakon et al also found that some cold tolerant life (psychrophiles) can still fix nitrogen at these low partial pressures when the temperature and UV flux of Mars is simulated (Sakon at al., 2005, March. A Further Analysis of Potential Photosynthetic Life on Mars) (Sakon at al., 2006. An analysis of potential photosynthetic life on Mars. ).
These experiments involve a partial pressure of nitrogen, in an atmosphere of other gases at normal terrestrial atmospheric pressures. Before we can apply these results to Mars we need follow up experiments to duplicate the Martian atmospheric pressure in a Mars simulation chamber and find out if these microbes from Antarctica or any other microbes can still fixate nitrogen at the same partial pressure of 0.2% at a total pressure of 0.6% . Sakon et al. proposed these experiments but I have not been able to find a record of anyone doing them (Sakon at al., 2006. An analysis of potential photosynthetic life on Mars. ).
So it seems that more experimentation is needed on the possibility of nitrogen fixation in Mars conditions.
If it is possible, this also depends on whether martian life developed nitrogen fixation. Nitrogen fixation is quite a late development on Earth. From various clues we know that nitrogen fixation first evolved in methanogens (methane producing microbes) when life had used up the naturally produced (abiotic) supply in hot hydrothermal vent type conditions in early Earth vents in sulfur rich conditions without oxygen. It was later on taken up by oxygen using life (Onstott at al., 2019. Paleo-rock-hosted life on Earth and the search on Mars: a review and strategy for exploration).
Early Mars may have had oxygen rich surface layers similar to those for Gale crater lake (Doyle, A., 2017, Ancient Lake On Mars Was Hospitable Enough To Support Life) (Lanza et al., 2014. High manganese concentrations in rocks at Gale crater, Mars) (NASA, 2017, A guide to Gale crater (video)).
We have three main possibilities here for early life on Mars.
Martian life might have never developed nitrogen fixation in the past.
- Scenario: no nitrogen fixation: life limited to deposits where abiotic fixed nitrogen is available,
Or perhaps early Martian life develop nitrogen fixation earlier than for Earth, but assuming a similar evolutionary path to Earth, perhaps the ability to fix nitrogen was never taken up by aerobes. If so, nitrogen from the atmosphere may be only available to life in deposits from anoxic environments in early Mars.
- Scenario: nitrogen fixation in anoxic environments only: life also in environments low in oxygen where it could access nitrogen from the atmosphere
And then finally if the ability to fix nitrogen was taken up by oxygen based life:
- Scenario: nitrogen fixation as fully developed as on Earth: everywhere on Mars with access to nitrogen, energy and trace elements
Even if Martian never developed nitrogen fixation and never developed photosynthesis in either the past or the present, this scenario need not mean a lifeless Mars.
Mars could still have had past life and may even have present day life, but both would be challenging to detect because they would be limited to habitats with
- chemical sources of energy
- sources of fixed nitrogen from comets, or non life processes on Mars
In this case,any life there may depend on naturally occurring nitrates, which may be a limiting factor for life.
Curiosity has discovered surface nitrates in low concentrations (~0.01–0.1 wt % NO₃) in Yellowknife bay drill sites. This overlaps the lowest end of the range for nitrates in the Atacama desert (~ 0.1 to greater than 1 wt% NO₃) (Ster et al., 2015. Evidence for indigenous nitrogen in sedimentary and aeolian deposits from the Curiosity rover investigations at Gale crater). These concentrations are consistent with impact generated nitrates from early Mars (Ster et al., 2015. Evidence for indigenous nitrogen in sedimentary and aeolian deposits from the Curiosity rover investigations at Gale crater).
Mars could also have an abiotic nitrogen cycle with photochemically produced HNO₃ fixed in thin (0.2 to 5 nm) pure water metastable interfacial films, potentially supporting up to one kilogram of fixed nitrogen per square meter (Boxe et al., 2012. An active nitrogen cycle on Mars sufficient to support a subsurface biosphere)
So, nitrogen might or might not be a limiting factor for present day life on Mars.
If life on Mars can fix nitrogen readily it could be found anywhere, not limited by the presence of nitrates or other forms of fixed nitrogen,
If it can fix nitrogen at such low levels, but only slowly, it may be present in low concentrations in regions where there is no fixed nitrogen available already.
On Earth nitrogen is returned to the atmosphere by microbial denitrification, which converts fixed nitrogen back to nitrogen gas. This would help to keep enough nitrogen in the atmosphere for widespread photosynthesis.
However interestingly in terrestrial deserts denitrification stops in the driest deserts. This leads to a possible extra "Swansong Gaia" feedback process involving denitrification that could keep nitrogen levels on Mars at low levels, with just enough nitrogen for nitrogen fixation throughout Mars, but so little nitrogen that it proceeds only slowly in habitats without access to nitrate deposits.
See Supplementary information under:
Or Martian life might be limited to habitats that already have nitrates or other sources of nitrogen. Nitrogen can also be a clue to the presence of traces of life organics, for instance, in amino acids/ So a preliminary detection of nitrogen in a potential habitat could be a clue to follow up to look for biosignatures though this needs caution because meteorites and comets also have nitrogen.
With all these scenarios, nitrogen is an interesting element to look for on Mars.
Several authors have suggested variations on a “follow the nitrogen” strategy to look for life. Capone et al. say that in a planet with both land and oceans, the presence of nitrogen in any form on the land is hard to explain without life supplied chemistry, because nitrates tend to dissolve in the presence of small amounts of water, and nitrogen has few geological reservoirs, apart from a few clays with ammonium substituted for potassium. Except for nitrates in very dry places, most would end up in the oceans .
The presence of nitrogen in any form should be a signal to planetary scientists to take notice. It may well be that the form and amount of nitrogen could constitute a roadmap for understanding whether chemical or biological processes were involved in its deposition. At least on a body that has had a separation of continental and oceanic components, the existence of nitrogen on continents is not easy to explain without special life-supplied chemistry.…
Thus, our recommended approach might be to search for the nitrogen; characterize and quantify it; if its abundance and chemistry cannot be explained by abiotic processes, do not leave until it is explained; and when it comes to sample return—bring back anything that is enriched in nitrogen!
(Capone et al ., 2006. Follow the nitrogen)
Shannon puts it like this
An oasis of liquid water on Mars would still be presumed sterile if no nitrogen—and therefore no nitrogen containing organic compounds—were detected. In this way, nitrogen might be a better target than the water itself.
(Shannon, 2006 . Elemental analysis as a first step towards “following the nitrogen” on Mars)
Bada et al suggested a “follow the nitrogen” strategy to look for biosignatures such as chirality by first looking for specific nitrogenous compounds used by life such as amino acids which may be preserved as components of stable fatty acids (such as lipids), sugars, and peptidoglycans (mureins, constituents of cell walls) (Bada et al., 2009. Seeking signs of life on Mars: In situ investigations as prerequisites to a sample return mission).
We may only find past or present day life after a long search of many geologically identical rocks - "every sample is a microbial island"
[FIXCITES]
The situation is very different for life and for geology. The geologists can see their rocks from space and we know Mars has rocks. But we can't detect life even with Perseverance's instruments because it doesn't have any in situ life detection. So we have no way to know if we are returning anything of interest for astrobiology past or present.
With many scenarios the chance of detecting past life in samples returned from Mars is very low until we are able to search for it first in situ on Mars (Bada et al., 2009. Seeking signs of life on Mars: In situ investigations as prerequisites to a sample return mission) (Carrier et al, 2020, Mars Extant Life: What's Next? Conference Report (html) : 802). The life needs to be
- deposited, not eroded away, not chemically altered (Hays et al., 2017, Biosignature preservation and detection in Mars analog environments : 4.4. Strategies and priorities)
- remain there for billions of years (McMahon et al., 2018, A field guide to finding fossils on Mars)) (Grotzinger, 2013, Habitability, Taphonomy, and Curiosity's Hunt for Organic Carbon)
- not have too much organics added from space to make it unrecognizable (Westall et al., 2015, Biosignatures on Mars: what, where, and how? Implications for the search for martian life : 999 - 1000)
First, in the present day on Earth, microbes may inhabit millimeter scale features in the rock. Cockell used terrestrial obsidian to gain insights into colonization of Martian rocks. Although obsidian hasn't been directly detected on Mars, the processes are similar. He found that many cubic centimeter samples are lifeless. He recommended a minimum sample size of ten cubic centimeters from a Martian rock, for a reasonable chance to detect life in it.
As he put it: Every sample Is a ‘microbial island’
In context:
5 - Every Sample Is a ‘Microbial Island’—the Need for Multiple Samples from the Same Material Type
With respect to sample collection, a notable observation is that in all these studies individual samples of the same material exhibit variations in microbial diversity ... In that sense, we can regard each rock or sample as a microbial island.
As sample size becomes smaller, especially in outcrops where rock heterogeneities are on a smaller scale compared to sample size, one might expect that diversity differences could increase as smaller patches of colonies are targeted. At large scales, biogeographical factors (such as environmental differences) will come into play.
...
Thus, within any given sample size, physical, chemical and biological factors are likely to make it extremely unlikely that two samples will exhibit exactly the same microbial diversity.(Cockell et al., 2019, Sample Collection and Return from Mars: Optimising Sample Collection Based on the Microbial Ecology of Terrestrial Volcanic Environments)
What about the past though? Might Mars have the equivalent of our coal beds or shale deposits, vast thick layers of life?
Well, it’s true Mars was far more habitable for terrestrial life at the time when the deposits in Jezero crater formed than today. However, Earth’s coal and shale deposits are relatively young. It is harder to find traces of life from the distant past, and we don’t know in advance where to look in the geological record for past life on Mars (Beaty, 2019) (Cockell et al., 2019c).
Even on Earth, we have little by way of organics from the most ancient stromatolites, and finding those was a huge challenge (Allwood, 2009). It is especially hard to find terrestrial organics that predate photosynthesis. The problem is most past organics are destroyed by geological processes and ionizing radiation from radioactive elements in the rocks.
On Mars also, most organics would be destroyed over billions of years, though some of the processes are different. The main processes include ionizing radiation, radioactive decay of elements in the rock, and reactive chemistry of the hydrogen peroxide and perchlorates (Grotzinger, 2013) (McMahon et al., 2018)..
This is especially so for surface or near surface organics. In less than half a billion years of surface exposure, grams of past life would be reduced to attograms (a billionth of a billionth of a gram) by its ionizing radiation levels far higher than for Earth, as we saw above.
Perseverance and Curiosity both found organics indistinguishable from organics from meteorites (JPL, 2021). Indeed, most Martian organics are expected to come from meteorites, interplanetary dust, and comets, or from indigenous organics from abiotic processes including abiotic photosynthesis (Mulkidjanian, 2015) and electrochemical reduction of mantle materials (Westall et al., 2015) (Franz et al., 2020).
We may need to look for organics that contain nitrogen, which is an essential part of any terrestrial cells and likely is required for similar non terrestrial biology. We look at this in:
So, we may need to look for past sources of nitrogen as well as organics. Even then we may also need to look for a source of energy in the past which might not necessarily be sunlight.
Then there’s the issue of preservation mentioned in the introduction to this section. We don’t yet know which Martian processes are most conducive to preserving fine structure and organics from past life. The preservation of biosignatures of past life depends on the history after deposition (Grotzinger, 2013) (McMahon et al., 2018). First, the organics can be degraded, altered or removed.
- Organics can be degraded by cosmic radiation if they remain close to the surface for hundreds of millions of years before they are buried, or are temporarily exposed to surface conditions at some later stage in their history.
- Later flooding can wash out organics.
- Warm conditions can flip organics to the mirror form of the molecule until there is a mix of both forms losing the chiral signature it may have had originally.
- Then the organics can be modified by chemical reactions, for instance with perchlorates, hydrogen peroxide and oxygen.
Preservation of the biosignatures also depends on the conditions in which the organics are deposited (Hays et al., 2017).
Preservation of the biosignatures also depends on the conditions in which the organics are deposited (Hays et al., 2017).
- The very conditions that make a habitat suitable for life, such as the flowing water that creates chemical redox gradients in a hydrothermal vent which life exploits for energy, can also degrade the biosignatures that help us to recognize that life.
- Similarly, the water that deposits the clays and other minerals that help preserve the biosignatures can also in turn destroy those biosignatures.
So we may need to find a sweet spot, where deposits from past life were also not degraded too much by the fluids that provided the habitat for life, for it to be preserved well enough to be recognized. As Hays et al put it
“Many of the conditions that make an environment more habitable are destructive to one or more of the biosignatures of interest”
Cockell et al. say ( Cockell et al., 2019b) that the processes that could preserve life on Mars are different from Earth because amongst other things, Mars
- Has no tectonic subsidence or burial to preserve life
- High concentrations of oxidizing agents such as perchlorates
- Tendency for acidic chemistries in habitats (which leads to less preservation of organics) and lower temperatures (which leads to less biomass)
Because of the acid conditions and the oxidising chemistry, amongst other reasons, a thriving past ecosystem could leave no preserved biomass on Mars. However, Cockell et al. consider that it is unlikely that nothing has been preserved of past ecosystems anywhere on Mars if it was inhabited on a planetary scale. If many lifeless sample are returned from many contexts, it would suggest that Mars never had life.
Cockell et al. say that a more likely scenario resulting from these challenges is that potential past life signatures are found but are ambiguous or close to detection limits, leading to similar debates as for ALH84001, where some think life was found and others think it wasn’t ( Cockell et al., 2019b).
Grotzinger, Project Scientist for the Curiosity Mars Science Laboratory mission, suggests we may need to find a “magic mineral” on Mars that preserves ancient life. He says that for Earth this key mineral was chert, a discovery from 1954. There may be similar key discoveries that open up a window into past life on Mars (Grotzinger, 2013) (Grotzinger, 2014).
The situation isn’t hopeless. Indeed these astrobiologists are reasonably optimistic we will find martian life eventually if it was there in the past. However, the general impression from the literature is they expect it to be a major challenge to find even abundant past life on Mars.
That is why so many of the astrobiologists say we should search for past life in situ first. Our first objective is to find it at all. Once we find it, that’s then when we would start looking into using the greater resources of our labs back on Earth to study it.
With this background we should be careful not to raise hopes with the public that we will find out whether or not Mars had life in the past with a few samples from a mission like Perseverance. We may do or we may find present day life, with a great deal of luck. Or we may not. We should NOT present this as a sure thing or a way to find out yes or no, if Mars had life in the past or has life at present.
Rather it is a first look for life which might find life from the past or the present but if so it will likely be due to a fortuitous fluke since Perseverance is not designed to look for life and rather is optimized for geology. It is only a first step which most likely leads to many more questions than it answers but can help us to prepare future astrobiology missions to answer the many questions it is likely to raise.
Life would be hard to find in a past or present day Mars without photosynthesis
Earth’s great deposits of shales and coal depended on photosynthesis, as did the stromatolites. Martian life might never have developed photosynthesis, or if it did, it might not have done so until after the Jezero crater deposits formed 3 billion years ago
If Mars evolved a biosphere, it may not have progressed to photoautotrophy or a dependence on photoautotrophy as it did on Earth. Thus, in the consideration of Martian environments conducive to producing molecular biosignatures, targeting depositional environments that had a strong chemical energy flux and sustained redox gradients for long periods by biogeochemical cycling is a most promising strategy.
( Summons et al., 2011:21).
In one plausible scenario early life on Mars hadn’t yet achieved photosynthesis back when it was at its most habitable. It is possible that it never progressed to photosynthesis even today. If that is the situation, it could take a lot of searching to find past life.
Ancient martian environments may not have remained habitable long enough for photosynthesis to evolve, and a worthy avenue of research would explore how biosignature signals would be expressed and preserved in this different energy regime.
(Biosignature preservation and detection in Mars analog environments : Section 5. Urgent Needs and Future Research)
Westall et al. suggest evolution of photosynthesis would have been more challenging on Mars than on Earth as the water might have been covered in ice most of the time because of the lower levels of sunlight. (Westall et al., 2013:894). In the scenario without photosynthesis (Hays et al., 2017), its past or present day distribution is likely to be even more heterogeneous (patchy) than in Earth’s deserts.
There is some evidence in the other direction of possible fossil stromatolites (Rizzo, 2020, Why should geological criteria used on Earth not be valid also for Mars? Evidence of possible microbialites and algae in extinct Martian lakes) as we saw above.
But these might be a late development. If we look at terrestrial samples from over 3 billion years ago, this is well before the Great Oxygenation Event, and we have very little evidence of organics or life from those times. For instance the banded iron deposits in Greenland are likely due to anoxygenic (non oxygen producing) photosynthesis but we don't have any direct evidence of the organisms that formed them. We look at this in the section on the Great Oxygenation Event below
If we do return samples that originally contained past life from Mars it may be as hard to interpret as samples on Earth that predate the Great Oxygenation event which they may resemble more closely than the very obvious traces of life in the coal and shale beds, or the fossil calcite coccolithophores shells in the chalk cliffs of Dover etc.
Proposal for bonus samples collected in 100% clean containers by the NASA Sample Retrieval Lander to help tease out fine details relevant to astrobiology
[FIXCITES]
The priorities of astrobiologists are different from geologists. All NASA's rovers and landers are guaranteed to find rocks. We can see them. So geologists at least know they have something to study. But astrobiologists need life, or biosignatures or something more than habitability assessments to make a start on their topic. So far we don't know if the subject matter of astrobiology exists on Mars. It may be there amongst the rocks, dirt, dust, salts and ice but we can't see it with any of the instruments sent to Mars so far, and could be hidden almost anywhere in the landscape, so we don't know where it is if present.
Also we will find that any past organics are likely to be too degraded to be noticed in the Perseverance samples
Astrobiologists can also detect a likely absence of life but only if they have a wide enough range of sampling locations to make deductions from a lack of detection.
- Present day life: with no in situ life detection, we'd likely need to drill into lots of boulders before we find patches of present day life if any.
- Past life: we need to find rocks with a young exposure age.
This is why in my NEPA comment (Walker, 2022, Comment posted December 20th) I suggested NASA and ESA collect bonus samples in STERILE containers sent on the Sample Retrieval Lander. In the comment I called it an ESA fetch rover but the plan now is a NASA Sample Retrieval Lander.
It will be assisted by two Sample Recovery Helicopters (NASA, n.d., Mars Sample Return Mission). (NASA, n.d., Mars Sample Retrieval Lander)
The marscopters for the Sample Retrieval Lander are discussed in this NASA news briefing:
Video: News Briefing: NASA's Perseverance Mars Rover Investigates Geologically Rich Area (Sept. 15, 2022 : 1: 02 : 00) also briefly at (31:44)]
So the proposal is to add capabilities to the lander to take bonus samples.
Text on graphic: Bonus samples:
- Thimbleful of dirt
[sterile scoop]- Salts [marscopter
- pebble from recent crater
[marscopter]- litre of atmosphere compressed
[air compressor as used for MOXIE]- a few grams of dust
[caught in air compressor filter]Needed because of 8.1 ppb contamination of all Perseverance samples with organics from terrestrial life
Background graphics from: (NASA, 2021. Mars Perseverance Sol 46: WATSON Camera )and (NASA, 2021. NASA's InSight Mars Lander Gets a Power Boost)
The aim of these bonus samples is to return
- A thimbleful of dirt (the more the better of course)
[needs sterile scoop] - Salts either in the dirt or ideally a second sample if there is a nearby salt concentration
[collected by marscopter] - A pebble from a recently excavated crater - based on cratering statistics for Mars there should be a crater within reach only a few tens of thousands of years old excavated to a depth of 2 meters or more
[collected by marscopter] - A litre of compressed atmosphere
[using similar compressor to MOXIE] - A few grams of dust
[ caught in the filters for the atmospheric compressor]
The dirt, dust and atmosphere can be sampled at the landing site. That would also include some pebbles for clean samples of rock from the landing site. However for the salts and for a pebble from a recently excavated crater we would need to use the Sample Retrieval Helicopters.
Pavlov et al suggested looking for recent impact craters and rocks exposed by rapid wind erosion or
perhaps in a deep valley.
(Pavlov et al., 2022, Rapid Radiolytic Degradation of Amino Acids in the Martian Shallow Subsurface:
Implications for the Search for Extinct Life : 1113)
The youngest crater our rovers have visited to date may be Concepción crater which Opportunity discovered and investigated. It may be only 1000 years old.
Proposal to use a marscopter to return a small pebble from a recently excavated crater to find organics not damaged by surface ionizing radiation.
10 meters diameter Concepción crater visited by Opportunity is likely only 1,000s of years old. Other craters it didn’t visit were as young as only decades old.
Image: (Bell, 2010. Look back at Concepcion). Superimposed: Ingenuity helicopter graphic from (NASA, n.d., Mars helicopter tech demo: highlights)
The 1,000s of years age for the Concepción crater is only approximate, between decades for the youngest craters and less than 100,000 years for craters they could date by the motion of sand dunes which hadn’t covered them (Lakdawalla, 2010. Opportunity’s thousand-year old crater)
There is a decent chance of finding a recently excavated crater close to the lander.
- Cratering rate for 16 to 32 meter diameter craters of 2.57 craters per square kilometer every ten million years [ (1.9 + 0.67 for first two rows in table 1 of (Utilizing decameter scale crater populations to study Martian history]
- An observational study by Daubar et al. found that this size of crater excavated the surface to depths of between 2 and 9 meters [based on seven newly formed craters at this size in (The morphology of small fresh craters on Mars and the Moon. : Figure 4).]
So there would be around 8 craters of this size younger than 10 million years within one kilometer of the landing site on average. Ideally we would want to find a crater only a few tens of thousands of years old. We may be able to find a crater from orbit, that looks young, and get Perseverance to inspect it close up.
The main issue would be whether the lander can land close enough to a crater for the Marscopters to get to it no matter where it is positioned in the landing ellipse. That may be easier if we find several young craters in the same area of Mars, for instance excavated by the ejecta from a nearby larger impact or just by random coincidence .
Assuming we want Perseverance to study the same crater (s) close up, we can do a rough calculation assuming Perseverance can be diverted to travel to an interesting crater at its maximum speed for 90 days.
- Perseverance can travel 18 km in 90 days at 200 meters a day (NASA, 2020 Mars 2020 Perseverance Landing Press Kit) if it is a priority
- Based on the cratering rate figures, 2616 craters of this size formed in the last 10 million years in any radius of 18 km,
- Which leads to a 99.9999999996% chance of a crater less than 100,000 years old within 90 days travel of Perseverance
This graphic shows the results of the calculation (not yet peer reviewed)
Details in my preprint: (Walker, 2023. NASA must protect Earth's biosphere even if Mars samples hold mirror life): With yet more ambition we can search for past organics using its Sample Recovery Helicopters to return pebbles excavated by a recent small impact crater to a depth of 2 meters or more with near certainty of exposure age less than 30,000 years)
(not yet peer reviewed, illustrative rough calculation)
A crater excavated to 2 meters may not be deep enough
Specifically, in a rock at 2 m depth and the exposure age of 500 million years, the original (primordial) abundance of amino acids would be decreased by a factor [a thousand] if a rock is a silicate and perchlorate-free and by a factor of [100,000] if a silicate rock at that depth contained 1% [of] perchlorate.
(Pavlov et al., 2022. Rapid Radiolytic Degradation of Amino Acids in the Martian Shallow Subsurface: Implications for the Search for Extinct Life : 1111)
However, this size of crater, 16 to 32 meters in diameter excavates to a depth from 2 meters up to 9 meters. There seems a reasonable chance of a crater excavated to many meters with an exposure age less than 100,000 years.
It's more tricky to return a sample from a young crater when we only have the two Sample Recovery Helicopters than it was with the plan for an ESA fetch rover. However the marscopters are able to communicate at a distant enough range to reach the edge of the landing ellipse for the ESA Sample Retrieval Lander, it may be possible to land it within range of a young crater, and then send the marscopters on multi-day journeys to travel to the crater - after a preliminary reconnaissance of the route by Perseverance which could also return a sample of organics from the crater.
The ingenuity marscopter is far faster than any of our rovers we sent to Mars. It traveled 12.1 kilometers. in its first 52 flights, or an average of a little over 4 flights to travel a kilometer. Perseverance's landing ellipse was 7.7 kilometers by 6.6 kilometers (NASA, 2021. Perseverance Rover Landing Ellipse in Jezero Crater).
If ESA can achieve a similar landing ellipse and the young crater and salt deposits are identified by Perseverance before the landing, then it should be possible to land within 3.3 kilometers. The marscopters could then retrieve a sample in at most 29 days if Perseverance scouts out the path first and communication is possible at 3.3 kilometers, or within a month allowing for a few days to explore the crater before returning.
Ingenuity's longest flight is 704 meters on April 8, 2022. (NASA, n.d., Mars Helicopter Technology Demo : Flight log). If the Sample Retrieval Helicopters can travel as fast as that they can return a sample from 3.3 kilometers away within 10 days round trip flight, or within two weeks, allowing for a few days to explore the crater to select the pebbles and take photographs to give it context before returning.
This would seem to be a high priority target for astrobiology, to
- find such a crater, with a young exposure age,
- send Perseverance to investigate it
- send a Sample Retrieval Helicopter to return a clean sample from it.
The Mars sample tubes are not designed for collecting the headspace gases and the Mars Sample Return teams don't yet know how they will be able to extract the gases from the tubes.
The sample tubes do not have a valve or port that can be used for gas extraction, so the tube will have to be penetrated by a yet-to-be-designed apparatus that will collect the headspace gas. There are several important considerations that must be factored into this process including
- the need to collect ALL of the headspace gas, as this is a very small volume of material, every molecule that can be collected is important for science and any incomplete collection may cause fractionation,
- this extraction needs to be performed without introducing terrestrial gaseous contamination into the gas samples or the sample tubes,
- the extraction processes must not introduce contamination into the sample tubes and compromise the pristine nature of the solid samples, which means that the materials used in the extraction system may need to be limited to the materials that are permitted to contact pristine samples
[converted the bracketed numbers to an ordered list]
(Carrier et al., 2022. Science and Curation Considerations for the Design of a Mars Sample Return (MSR) Sample Receiving Facility (SRF) : S-230)
But Swindle et al. say that the headspace gases are not likely to be useful for science because they will already be too contaminated by gases from Earth's atmosphere, the Martian atmosphere, the seals of the sample tubes are not guaranteed to be air-tight for the return to Earth with tests showing so there might be some leakage both ways, and outgassing from the samples and the walls of the sample tube.
The quantity of gas present within the sample tube volume is insufficient for many investigations, and there will be exchange between solid samples, headspace gas, and tube walls. Importantly, the sample tube materials and preparation were not designed for optimal Mars atmospheric gas collection and storage as they were not sent to Mars in a degassed evacuated state and have been exposed to both Earth’s and Mars’ atmospheres. Additionally, there is a risk of unconstrained seal leakage in transit back to Earth, which would allow fractionation of the sample (leak-out) and contamination (leak-in).
(Swindle et al., 2022. Scientific Value of Including an Atmospheric Sample as Part of Mars Sample Return (MSR) :abstract)
Findings (1) When considering the head space gas in tubes with samples in them, we conclude that the relatively small volume, potential of seal leakage, and especially the likelihood of interaction of atmospheric gas with the sample and tube will make it difficult to answer any fundamental questions about the martian atmosphere
(Swindle et al., 2022. Scientific Value of Including an Atmospheric Sample as Part of Mars Sample Return (MSR) : S-167)
Swindle et al. also found that the insides of the tubes were coated with titanium nitride and nitrogen was used to deposit this too. This coating will absorb and release gases. Nitrogen is one of the priority gases to measure in the martian atmosphere. Also there would be issues with detecting ratios of the noble gases Krypton and Xenon because Krypton is absorbed more easily than Xenon which will skew the ratio. The ratio of krypton to xenon is another key measurement for the atmosphere (Swindle et al., 2022. Scientific Value of Including an Atmospheric Sample as Part of Mars Sample Return (MSR) : S-169).
They also found that because of the high partial pressure of nitrogen in Earth's atmosphere relative to any nitrogen in the tubes from the martian atmosphere that measurements involving nitrogen are likely to be especially suspect with even a small leak of nitrogen making some of the most important measurements impossible.
They calculated that probably about 5% of the atmospheric samples in each tube will be lost to the vacuum of space during the journey back, but there were many uncertainties in this calculation based on the limited data they had about tests of the sample tubes. The data they had was only for sealed blank tubes. If dust from the samples or the atmosphere gets into the seals then this could lead to a much more leaky seal and the samples could lose virtually all the headspace gases during the journey back.
In Appendix 3, we calculate the amount of leakage that could occur during a two-year period when exposed to the vacuum of space and find that 5% of the gas would be lost, assuming a He leak rate of 1 · 10-8scc/s [i.e. equivalent to a 100 millionth of a cubic centimeters per second at atmospheric pressure].
This amount of loss would be a concern, but marginally acceptable. The tests performed to date suggest that it is likely that most tubes will have lower leakage rates, which would diminish the problem. However, we would not know the leak rate of a specific seal until the sample is returned to Earth, if then, and if only 80% of the sample tube seals are that tight, then 20% would be at higher levels.Furthermore, the likely event of dust from the martian environment settling onto the seals will increase the likelihood of leakage. So, it is also possible that virtually all the sample could be lost, rendering the effort useless. In addition, after arrival in the Earth’s atmosphere, the pressure of Earth atmosphere will be two orders of magnitude higher than the content of the sample tube, with the partial pressure of nitrogen displaying an even larger difference, as nitrogen is a trace constituent in the martian atmosphere and the major constituent in Earth’s atmosphere. Contamination, even with a small leak, could render some of the most important measurements impossible.
(Swindle et al., 2022. Scientific Value of Including an Atmospheric Sample as Part of Mars Sample Return (MSR) : S-169)
In short the samples of headspace gases likely won't let us make several of the most important measurements. In the worst case if the dust gets into the tube seals and makes them particularly leaky, all the gas is lost during the journey back. So, based on he data they had available to them, which is incomplete, we should be prepared for the possibility that there may be no headspace gas left to analyse.
Swindle et al. recommend sending a sample tube purpose designed to contain an atmospheric sample to Mars with either of the two spacecraft sent to collect the samples. . They conclude that (Swindle et al., 2022. Scientific Value of Including an Atmospheric Sample as Part of Mars Sample Return (MSR) : S-167) (summary paraphrase of their conclusions):
- It will be difficult to answer any fundamental questions about the martian atmosphere from the headspace gas in sample tube because of interactions with the sample and the tube.
- If Perseverance returns a sealed sample tube containing only atmosphere, it can provide significant science return about the martian atmosphere though less than with a sample tube designed to minimize outgassing.
- Much more science return is possible with a sample tube custom designed to collect atmosphere and sent to Mars. These are normally baked for at least 200°C for several days and sealed before use. The Perseverance tubes weren't prepared in this way.
This bonus sample tube would be valved, the valve opened when it arrives on Mars to collect the sample, and closed again before the return journey, and the valve mechanism would be tested before the flight to make sure the leak rate is very low when it is closed.
So if we want good data about the martian atmosphere it does seem of high importance to add a bonus atmospheric sample. The atmospheric compressor approach is a step up from a dedicated sample tube sent to Mars to collect atmosphere, of greater science value than an uncompressed sample, and it has the major advantage for astrobiology that it gives an opportunity of a bonus sample of dust also collected at the same time, which we could collect in a clean container too, like the atmospheric sample.
This shows how the atmospheric compressor works. It’s using a proposal submitted to the decadal survey in 2020 by Jakosky et al.
First it uses the getter to remove evolved gases from the container wall. Then it closes one microvalve and opens another to get an atmospheric sample. Finally it closes both microvalves to the gas container and opens the vent to run more atmosphere through the compressor to collect dust in the filter
(Jakosky, 2020. Scientific value of returning an atmospheric sample from Mars)
Swindle et al. recommend this approach as better than their suggestions because it is purpose designed to collect the atmosphere and would collect far more atmosphere making it easier to detect trace amounts of important gases.
An alternate solution to filling a single tube and/or utilizing the head space gas of sample tubes would be to collect a 100 cc sample and compress it by a factor of 100, as proposed by Jakosky et al. (2020).
- Such a sample would make all the measurements discussed here possible, as well as enable measurement of the carbon isotopes in the enigmatic martian methane, a preferred solution from a scientific standpoint.
- The potential for contamination will also be less if a concentrated sample, containing far more moles, is analyzed.
- There are also numerous analytical advantages of collecting the present-day martian atmosphere sample in a container that is treated and sealed prior to launch rather than having been exposed sequentially to the terrestrial atmosphere, the vacuum of space, and the martian atmosphere.
[split up into bullet points for presentation here) (Swindle et al., 2022. Scientific Value of Including an Atmospheric Sample as Part of Mars Sample Return (MSR) : S-168)
Grady et al. calculate that using the turbopump suggested by Jakosky, 10 hours of pumping for 10 days could collect about 1 milligram of dust with a total of between a million and a billion dust particles (Grady et al., 2022. The Scientific Importance of Returning Airfall Dust as a Part of Mars Sample Return (MSR) : S-183)
We can detect life from the Gobi and Taklimakan Deserts in dust in Japan (Aeolian dispersal of bacteria associated with desert dust and anthropogenic particles over continental and oceanic surfaces).
Text on graphic: Microbes in dust from the Gobi desert detected in Japan (Yonago).
Martian dust storms might transport dead or alive microbes great distances.
Graphic: distance Gobi desert to Yonago
So we may have a chance of detecting viable or recently dead life from far away on Mars brought to Jezero crater in the dust storms.
For this we need:
- A sterile scoop for the NASA Sample Retrieval Lander
- Sterile containers and the atmosphere compressor also sent with the fetch lander
- A way for a Marscopter to pick up a pebble from a newly excavated crater without contaminating it and drop it in front of the NASA Sample Retrieval Lander so that it can pick it up in the scoop.
The pebble would ideally be from a rock that has organics in it, To try to ensure this, Perseverance could analyse the same stratum - but far from the pebble so as not to contaminate it.
It would help us to understand past organics better if Perseverance also collects a sample of organics from a recently excavated crater. There might be enough of the undegraded organics to not be overwhelmed by the forwards terrestrial contamination of the Perseverance samples.
However the levels of organics in Mars meteorites are not necessarily the levels of organics we'd expect for past life. Most of any organics we return may be produced natively on Mars in an abiotic fashion, infall from space or altered. If there are past organics they may be in trace amounts mixed in with all the other organics produced on Mars or from space that are nothing to do with life. See below:
We need to collect this bonus sample from the most interesting geological layer we can find in a clean container for useful astrobiology looking at the very minute traces they are interested in for any remaining traces of past life or of the prebiotic chemistry at the time, bearing in mind that Mars may have had uninhabited habitats at the time, especially if life hadn't yet developed such capabilities as photosynthesis or nitrogen fixation.
To keep the sample collection apparatus clean, the whole apparatus - the air compressor, dust sample collector, the sample container(s) for the salts, pebble and scoop of dust and the scoop itself could be sent in a sealed container. The simplest approach would be to cover them in a membrane that would be broken on Mars to remove them as for the Phoenix arm (Bonitz et al., 2008, NASA Mars 2007 Phoenix Lander Robotic Arm and Icy Soil Acquisition Device : Figure 6). See below:
However a far higher level of cleanliness is possible than for the Phoenix arm. For the cleanest possible samples, the final stage of sterilization on Earth can be done inside the biobarrier in a large vacuum chamber which would ensure that there are almost no air molecules left inside the membrane before it is sealed and all the containers use coatings that are designed not to absorb or emit gas so that any gases in the samples come from the samples themselves. It would be 100% sterile, clean and vacuum sealed with the seal only broken on Mars.
Alternatively or as well these could all be designed to resist heating and be heated for a few minutes to 300 C with a small inbuilt heater on Mars.
For the return to above GEO they would need to be kept in a larger container that is sealed and leak free so that they remain at mars atmospheric pressure, since if the pressure is reduced to vacuum conditions the samples might well be altered and life that is used to martian conditions might die. Perhaps this could involve a flexible biobarrier, enclosing a physical container, if this can be sealed adequately on Mars.
Then based on the Viking experiments, it would seem to be important to have a window to let natural sunlight into part of the sample of dirt so that the active agent responsible for the Viking labeled release data, whether it is chemical or biological, remains active for the six months journey back to Earth, giving some chance of replicating the Viking labeled release experiment results and understanding them better, which otherwise would be impossible. That's because the Viking Labeled Release experiment found that its samples were inactivated by being kept in darkness and at a temperature of at least 10°C for 141 sols (Viking 1 cycle 4) or 84 sols (Viking 2 cycle 5) (Levin et al., 2016, The case for extant life on Mars and its possible detection by the Viking labeled release experiment : 3 The Viking LR Data).
For details see the end of the section below:
- The Viking results still challenge the chemical explanation, an issue for clean samples to help resolve which continues from:
- Even iMost's proposed more sensitive version of the Viking labeled release experiment can be done by sterilizing the evolved gases for analysis on Earth
The Mars surface never goes down to below 170°K or around -100°C (Atri et al., 2022, Diurnal variation of the surface temperature of Mars with the Emirates Mars Mission: A comparison with Curiosity and Perseverance rover measurements : Figure 3). If the return vehicle goes into the shadow of Earth on the way back it might have temperature drops to -196°C (NASA, n.d. Environmental Conditions for Space Flight Hardware - A Survey : Table 7 and page 6), which might need attention in case there are lower temperature limits to survivability of the martian biochemistry whatever it is.
This suggestion to grab some dirt and return it is similar to a suggestion for a minimal sample return mission once made by Chris McKay to just “grab a sample of dirt” (McKay, 2015, interviewed by Leonard David, for Space News (Q&A with Chris McKay, Senior Scientist at NASA Ames Research Center), but with additions to enhance the interest for astrobiology using the capabilities of the marscopters to return materials from further away and the technology of an atmospheric compressor already demonstrated on Mars with MOXIE.
We should expect these first clean samples to be as ambiguous as the martian meteorites we have already
Also if we do detect features that look like past life, what we return is most likely to remain ambiguous at this stage as for the martian meteorite ALH 84001
A more likely scenario is that potential signatures of life might be equivocal, ambiguous or close to detection limits. Such samples might be considered lifeless by some workers and not by others.
…
Although Mars sample return and rover missions are strongly focused on finding evidence for life, lifeless samples returned from Mars will yield important constraints on the extent of habitable conditions and whether those environments were inhabited.
The scenarios discussed here show that it is critical to acquire many samples. Multiple samples must be obtained from each locality, from similar (paleo)environments elsewhere across Mars, and from different potentially habitable environments in multiple locations across Mars for the scenarios presented here to be disentangled and the reasons for lifeless samples ascertained.
(Cockell et al., 2017, Lifeless martian samples and their significance).
Clean samples as just the second step after the Viking landers in Carl Sagan's "Vigorous program of unmanned exobiology"
Even with clean bonus samples, it is important to present this to the public as just the second step in Carl Sagan's "Vigorous program of unmanned exobiology"
Carl Sagan: Because of the danger of back-contamination of Earth, I firmly believe that manned landings on Mars should be postponed until the beginning of the next century, after a vigorous program of unmanned martian exobiology and terrestrial epidemiology.
(Sagan, 1973, The Cosmic Connection - an Extraterrestrial Perspective)
It would take a significant element of luck to find either past or present day life with the bonus samples - but we are guaranteed to find out much more about subtleties of surface chemistry with clean samples. Mars could have very complex abiotic or prebiotic chemistry even if it doesn't have life, and if we are lucky we return life from a microbial oasis or find viable or recently dead life in the dust. For the abiotic or prebiotic chemistry see above:
The two Viking landers were the first step. We have sent no life detection missions to Mars since then. We can expect to make some progress in understanding what Viking saw. But we may well end up with as many questions as we resolved. The data the Viking missions collected suggested at the least that the chemistry is complex and if it didn't find life it may leave unanswered questions about the chemistry.
Mars is far more complex now than we thought when Carl Sagan wrote "The Cosmic Connection" three years before the Viking landers landed on Mars. The surface of Mars is similar in size to the land area Earth's continents and islands. And from a microbial perspective it may be similar in complexity to Earth. See:
- ALL four of the currently confirmed or uncertain novel microenvironments found on Mars relevant to native martian life were surprises
- Potential for distant microbial oases as sources of ultra low concentrations of viable spores in dust storms
- Scenario of a microbially diverse Mars with microbial oases with unique species analogous to the Galapagos finches
So we are likely to need many missions for a reasonably thorough exploration of Mars. We are at great risk of forward contamination if we send many robotic explorers to sensitive areas of Mars using the Perseverance level of cleanliness or even the far more rigorous Viking mission level of cleanliness.
It’s important not to present this to the public as the mission that will settle central questions of astrobiology. With better samples in clean containers, it may have some potential to detect past or present day life, but it would take a significant element of luck to be so successful with a first mission.
Rather, this is the second step in our exploration of Mars to search for life (the Viking missions would be the first step as no mission since then has tried to find life).
It is also just the first step for the search for past life.
Even iMost's proposed more sensitive version of the Viking labeled release experiment can be done by sterilizing the evolved gases for analysis on Earth
[FIXCITES]
The idea of the Viking labeled release experiment was to test for microbial respiration. Earth organisms can produce a wide variety of gases such as methane and carbon dioxide, which both contain carbon, ammonia and hydrogen sulfide.
The Viking labeled release experiment worked by feeding the samples with organics labeled with radioactive carbon 14, This made it easy to test for carbon in the evolved gases by testing for radioactivity. This diagram from the Viking press handbook shows how it worked: .
Droplets of food ‘labelled’ with radioactive carbon 14.
Checks to see if anything ‘ate’ the food and ‘breathed out’ radioactive CO2 or methane.
Results were hard to explain as life or as non-life – never sent a follow up experiment to find out more.
Controversial to this day – a few think it did find life.
(NASA, Viking Press Handbook: 61)
We can also test for isotopes of other gases like hydrogen and nitrogen in the Martian atmosphere but for the most sensitive measurements we need to use particle accelerators. These are too large to send to above GEO at present.
We use these accelerators for carbon dating and other sensitive measurements of isotope ratios. They split the sample into component atoms, by hitting the sample to be analysed with a stream of Cesium ions (Cs+) which detaches individual charged ions by sputtering. These are then separated by accelerating them in a particle accelerator. Cesium ions are used because Nitrogen 14 isn't ionized when hit by them. (McWade, n.d., Accelerator Mass Spectrometry)
However sterilization doesn't change the isotope ratio. So we could return samples from above GEO for analysis in the same way. These photographs show three of the mass spectrometry at CAMS, the Lawrence Livermore National Laboratory Center for Accelerator Mass Spectrometry.
(CAMS, n.d., The CAMS Accelerator Facility)
Some Accelerator Mass Spectrometers can be miniaturized to some extent. The Yale MICADAS accelerator mass spectrometry system for carbon dating can be placed on a lab bench 2.6 meters by 3.4 meters, simplified because it is designed only for testing isotope ratios for Carbon 14 dating.
Caption: The MIADAS miniaturized accelerator mass spectrometer specialized for ultrasensitive study of Carbon 12 / Carbon 14 ratios for carbon dating
Image from (CNA, n.d., MiCcDaS Accelerator) with early version described in (Synal et al., 2007. MICADAS: A new compact radiocarbon AMS system) It's available from IonPlus with dimensions 3.2 m x 2.6 m x 2 m (IonPlus, n.d., MICADAS)
However we need a general purpose accelerator able to test many different atoms for these experiments. Even a decade from now we may have novel technology we can use but at present the only solution seems to be to collect headspace gases at regular intervals and then sterilize all those samples and return them to Earth from time to time for analysis. We could do preliminary analysis in space however.
In more detail, the Viking experiment tested for radioactive carbon in the evolved gases.
The LR procedures for the Viking landers … measured evolved 14 CO 2 gas given off by 14°C -labeled carbohydrates reacting with constituents of the martian regolith. The widespread importance of the Krebs cycle in aerobic metabolism and the Embden-Meyerhof pathway in anaerobic metabolism makes the use of 14°C -labeled metabolites highly efficient in detecting microbial metabolism because both pathways produce carbon dioxide
(Schuerger et al., 2003, (Viking biology experiments: lessons learned and the role of ecology in future Mars life-detection experiments : 235)
Here the Krebbs cycle also known as the citric-acid cycle is used by eukaryotes as part of the process of using glucose to produce ATP in mitochondria, using up oxygen and producing CO2 as a byproduct (Citric acid cycle). The Emgden path is a simpler faster process that metabolizes glucose to ethanol and CO2 used in yeasts in the process of fermentation and by anaerobic microbes generally - microbes that don't or can't use oxygen. It's also known as anaerobic glycolysis (Energetics of Anaerobic Glycolysis)
This much is an experiment that can be done in the orbital laboratory already since it was done on Mars with 1970s technology. At first sight the experiment seemed to detect life, but neither of the other experiments seemed to detect organics and and the experiment didn't show the response of increased activity after a second injection of organics that we see with nearly all terrestrial samples of life.
The results gave “classic” biological responses for the two active cycles, and a negative response for the heat-sterilized cycle (cycle #2). These results were accepted as strong evidence for biological activity in martian regolith by Levin and Straat.
The controversy on this interpretation is based on two key points: (i) following a 2nd injection of the 14°C -labeled nutrient solution, the radioactivity decreased instead of increased as would be expected if the 14 CO2 gas was derived exclusively from biological activity (Levin and Straat 1977), and (ii) no organics were found in the Mars regolith with the Viking GCMS experiment. Although Levin and Straat have responded to these and other criticisms, the biological interpretation of the Viking LR response is considered unverified by most of the exobiological community
(Schuerger et al., 2003, (Viking biology experiments: lessons learned and the role of ecology in future Mars life-detection experiments : 235)
Levin and Straat's response to those two criticisms is that (Levin et al., 2016, The case for extant life on Mars and its possible detection by the Viking labeled release experiment]
- their experiment was able to detect as little as 30 cells in a sample, a population so small that the other two experiments wouldn't detect the organics.
- A sample of soil from Antarctica duplicated the result of a fall in CO2 after the second injection (though not such a deep fall) with a sample from Antarctica. So though the response they found is rare with terrestrial samples, they were able to replicate it with a sample from a Mars analogue environment.
In the labeled releaser experiment the gas evolution leveled off at 2 sols and then the total CO2 in the headspace region began to plateau at 7 sols, suggesting much less CO2 was produced at that point, and stopped completely by 25 sols. That could show that it was running out of substrate in the soil, or that the active agent "died" or both. The additional wetting would have absorbed CO2 into the wetted alkaline soil.
[ILLUSTRATION NEEDS PERMISSION (Levin et al., 2016, The case for extant life on Mars and its possible detection by the Viking labeled release experiment : Fig 4 and Fig 2)]
Side by side comparison of the Viking experiment with an experiment using soil from Antarctica. The Antarctic soil shows a similar null response though with less of a dip in the radioactive CO2 which Levin attributes to the CO2 taken up by alkaline soil. +
Levin agreed that the Viking labeled result is not conclusive
The general consensus at the time of Viking was that the LR positive response was nonbiologically caused. However, since then, information from later missions to Mars has called the basis of that consensus into question, and it is timely to reconsider a biological explanation for the LR results.
(Levin et al., 2016, The case for extant life on Mars and its possible detection by the Viking labeled release experiment : section 5)]
He proposed a follow up experiment on Mars, a chiral version of his labeled release experiment, which would detect any preference for one symmetry of the organics over the other (Anbar et al., 2012, A Chiral Labeled Release Instrument for In Situ Detection of Extant Life ).. Life on Mars might well be able to use organics of both symmetry, since some terrestrial life is able to metabolize organics of the opposite symmetry, but it is likely to show a preference for one symmetry which would be hard to explain chemically. (Levin et al., 2016, The case for extant life on Mars and its possible detection by the Viking labeled release experiment : section 5). This experiment has never been sent
The iMost team agreed on the importance of repeating and improving on the Viking experiment with the Perseverance samples, saying that modern instruments can achieve a major improvement in sensitivity and can test a far wider range of organics for any present day martian life to use, summarizing it like this
A primary objective of NASA’s Viking Mission to Mars was to search for evidence of extant life.
Two Viking landers carried out a series of biological experiments, including the labeled release experiment, which was designed to detect metabolic activity. In this experiment, simple 14C-labeled substrates, water, and martian soil were combined and monitored for the evolution of radioactive gases (Levin & Straat, 2016) as evidence that microorganisms had metabolized the substrate. Viking instruments detected 14C labeled gas, however, it is widely accepted that those data were due to oxidizing agents in the regolith rather than microbial activity (Lasne et al., 2016).
Despite the controversy surrounding the labeled release experiment and the eventual conclusion that it did not find evidence of life, the rational underpinning of the experiment may be useful in guiding the search for life in the M-2020 returned samples—the idea to search for evidence of active metabolic processes. The orders of magnitude improvements in technology and the availability of sophisticated Earth laboratories would greatly improve sensitivity and allow us to test for metabolic processes beyond the ability to catabolize a handful of simple carbon substrates (a metabolism not likely to exist on the surface of Mars). It would also attenuate the probability of ambiguous and controversial results as seen during the Viking mission.
In this investigation, samples would be incubated with ‘heavy’-isotope-labeled substrate using various combinations of deuterated water, 13C- or 15N-labeled substrates, and other stable isotope labeled substrates (Trembath-Reichert et al., 2017).
(Beaty et al., 2017, iMOST : 92)
Replicating the Viking experiment is also important for study of sensitive abiotic chemistry. In the chemical explanation, the gases were evolved due to interactions with perchlorates. If that is what happens then analysis of the headspace gases can help to examine subtle details of the process which we can then attempt to replicate in terrestrial labs with Mars analogue soils.
This is another paper going into details on the method the iMost team describe, using labeled hydrogen and nitrogen as well as carbon. This is quoting from the source the iMOST team cited:
Taking advantage of the ability to measure three different stable isotope tracers in parallel with NanoSIMS, we applied combinations of 13C- and 15N-labeled substrates in tandem with deuterated water (2H2O) as a passive tracer for growth. The use of 2H O with NanoSIMS is an effective method for measuring single-cell microbial biosynthesis with high sensitivity, capable of detecting extraordinarily slow rates of growth … predicted to be characteristic of subseafloor biosphere ecosystems
This multiple-isotope SIP-NanoSIMS study of microbial life in deep subseafloor environments recovered evidence for methyl substrate metabolism in deep coal and shale beds and exceedingly slow growth within this low-abundance, high-temperature microbial community.
(Trembath-Reichert et al., 2017, Methyl-compound use and slow growth characterize microbial life in 2-km-deep subseafloor coal and shale beds)
The Viking results still challenge the chemical explanation, an issue for clean samples to help resolve
In more detail the Viking labeled release experiment involved dropping the nutrient solution into the dry soil, creating a humidity gradient (Levin et al., 2016, The case for extant life on Mars and its possible detection by the Viking labeled release experiment). The experiment was done at 7 to 10°C in darkness with the martian atmosphere pressurized up to 85 millibars (8.5% of terrestrial atmosphere) with helium (Levin et al., 2006, Modern myths of Mars : Figure 1).
The method they used is one of our most exquisitely sensitive methods for testing for the presence of life on Earth. In their tests with terrestrial samples, Levin et al. were able to detect the respiration of as few as 30 biologically active cells in the sample (Levin et al., 2016, The case for extant life on Mars and its possible detection by the Viking labeled release experiment).
Most of the results can now be explained as complex chemistry. Quinn et al. in 2013 suggested that the perchlorates in the soil detected by the Phoenix lander were decomposed through gamma radiation to hypochlorite (ClO -), trapped oxygen, and chlorine dioxide. Then the hypochlorite reacted with the 14C-labeled alanine to produce chloroalanine which then decomposed to produce the 14C-labeled CO2. (Quinn et al., 2013. Perchlorate radiolysis on Mars and the origin of martian soil reactivity). This didn’t explain everything and a follow up paper by Georgiou et al. filled out the picture some more but is still not a complete explanation (Georgiou, 2017. Radiation-driven formation of reactive oxygen species in oxychlorine-containing Mars surface analogues)
There are points in favour of the hypothesis of life too. Levin and Straat in a paper published in 2016 review some of the issues they have found with this and other abiotic proposals (Levin et al., 2016, The case for extant life on Mars and its possible detection by the Viking labeled release experiment).
- Two of the labeled release experiments got inactivated after storage in darkness for 141 sols (Viking 1 cycle 4) or 84 sols (Viking 2 cycle 5) at and above 10 °C
- Activity of the soil is significantly reduced if heated first to 50 °C.
- Heating to 160 °C inactivates the active component
Two of these three points are answered by Georgiou et al. Their explanation is that the chemical explanation needs to take account of the effects of ionizing radiation and also of UV radiation on the perchlorates. This leads to a complex chemical situation including very reactive chlorates (ClO3), chlorites (ClO2), hypochlorite (ClO), and hydrogen peroxide amongst others. They found that one of these reactive chemicals, sodium hypochlorite (NaCLO) is thermally unstable and in Martian conditions. They find less of this hypochlorite after heating at 20 to 50C and almost all gone after heating to 160 C. They don't attempt to reproduce the Viking experiments exactly but these findings are close enough to the Viking lander results to be suggestive that a chemical explanation could match the Viking results (Georgiou, 2017. Radiation-driven formation of reactive oxygen species in oxychlorine-containing Mars surface analogues : 333).
I haven't found any attempt at an explanation in the literature for how chemicals could be inactivated by being kept in darkness at 10 °C and above for 2 months. So the first of those three points I highlighted in Levin's paper awaits a chemical explanation. It doesn't seem to have been discussed yet in the literature on a chemical explanation of the Viking results.
Another interesting sidelight here is Miller ’s reanalysis of the old Viking data in 2002 found an offset of the evolved gases from the diurnal maximum temperature by two hours. This is especially hard to explain by abiotic processes, as the evolved gases would take only 20 minutes to reach the detector. As an expert on circadian rhythms, Miller said they look like circadian rhythms (Miller, 2002, Periodic analysis of the Viking lander Labeled Release experiment). He suggested this may be a biosignature in the data. A later complexity analysis seemed to support this interpretation (Bianciardi et al., 2012, Complexity analysis of the Viking labeled release experiments).
This two hour offset of the peak of the evolved gases doesn't seem to be discussed further in the literature on the topic, just one sentence mentions, for instance in (Levin, 2021, The case for extant life on Mars and its possible detection by the Viking labeled release experiment). and in (Teunis et al., 2021, Clays and the Origin of Life: The Experiments).
The Miller and Bianciardi papers are not cited by Georgiou (Georgiou, 2017. Radiation-driven formation of reactive oxygen species in oxychlorine-containing Mars surface analogues) or Quinn (Quinn et al., 2013. Perchlorate radiolysis on Mars and the origin of martian soil reactivity). So it seems this observation also awaits a chemical or physical explanation.
The majority view is Viking didn't detect life. However it doesn't yet appear to be a consensus. We don't have enough information to convince everyone with 100% certainty of the majority view, or indeed the minority view. But this doesn't matter for the experiments. We need to do the same experiments whatever ones view on the Viking experiments. We can't yet decide for a certainty between:
- it detected life [minority view],
- there is no life there and Viking detected complex chemistry [majority view]
- there was life there that Viking didn't detect but more sensitive experiments might detect, including modern more sensitive versions of the Viking labeled release experiment using a wider range of isotopes
[the hypothesis the iMost team wish to test]
This is something we can in principle resolve. That then can be a basis for future studies on Mars. The iMOST team are agreed that we need to do these experiments.
The summary for the 2019 conference on Extant life What's Next came to a similar conclusion to the iMost team about the value of using advanced forms of the Viking instruments with the nitrogen and sulfur also labeled (with non radioactive isotopes in those cases).
The results of the Viking biology experiments were equivocal—some aspects of the results were consistent with what would be expected if extant life were present in the samples, but other results were not, thus allowing for multiple interpretations.
Subsequently, scientists proposed explanations for the Viking biology experiment results that do not require the presence of life.
Another type of biosignature of familiar life is the presence of an active metabolism through direct detection of known metabolites such as adenosine triphosphate (ATP).
Metabolic activity of familiar life could be determined by measuring trace gases such as methane and complex volatile organic compounds (VOCs) respired or consumed by extant life.
The uptake of labeled isotopes (C, N, S) such as in the Viking Labeled Release experiment can provide data on metabolic activity and the enantiomer ratio (chirality) of the sample.
Finally, it is important to note that the definitiveness of these results would be dependent on our ability to distinguish signals from noise and would depend on individual results within the context of the entirety of the results.
(Carrier et al, 2020, Mars Extant Life: What's Next? Conference Report (html) : 801)
We can find isotope ratios by in situ measurements using mass spectrometers as for the Viking, Phoenix and Curiosity measurements of isotope ratios in the martian atmosphere (Mahaffy et al., 2013. Abundance and Isotopic Composition of Gases in the martian Atmosphere from the Curiosity Rover).
One of the issues with interpreting the Viking results is that it detected chlorinated organics which were initially dismissed as contaminants from Earth. Were these the result of the reaction of the perchlorates with surface organics during the analysis which involved heating in an oven? From Curiosity results it now seems that they may be indigenous but there may be traces of organics too.
Following the detection of perchlorates, it was questioned whether the chlorinated organic molecules detected with Viking’s TV-GC-MS experiment were oxidation products of organic molecules present in the pyrolyzed samples. More recently, the Curiosity rover of the MSL mission confirmed the presence of oxychlorine compounds in the regolith of Mars and detected traces of indigenous organic molecules. However, the structure of the molecules present in the regolith may have been altered over geological time in the harsh environment of Mars and may also have been modified during their extraction prior to their detection by in situ analysis instruments.
Consequently, a good knowledge of the reactivity of the martian regolith is essential to understand the possible transformations and ultimately the origin of organic molecules.
(Lasne et al., 2016, Oxidants at the surface of Mars: a review in light of recent exploration results)
In more details. The Viking labeled release experiment seemed to detect life. Two other life detection experiments didn’t detect life, but were less sensitive than the labeled release.
Video: Viking's Biological Experiments
The Viking chemical analysis experiment (TV.GC .MS) didn’t detect any likely looking organics. It heated the samples to 200°, 350°, and 500°C to evaporate small organic molecules and break up large ones which it then analysed by separating them chemically and then by mass. The only organics it found were (Navarro - González et al., 2010. Reanalysis of the Viking results suggests perchlorate and organics at midlatitudes on Mars):
- Dichloromethane CH2Cl2 (Viking Lander 1).
- Chloromethane CH3Cl (Viking Lander 2)
The experimenters dismissed these as likely due to terrestrial contamination, even though they weren’t detected in blank runs on Mars, because the chlorine 37 / 35 isotope ratios were similar to Earth isotope ratios. .
However we now know that Mars has perchlorates, first discovered by Phoenix in 2009 (Hecht et al., 2009. Detection of perchlorate and the soluble chemistry of martian soil at the Phoenix lander site). These chlorohydrocarbons are exactly what you’d get from 0.1% organics reacting with the perchlorates when heated in the Viking ovens for analysis (Navarro - González et al., 2010. Reanalysis of the Viking results suggests perchlorate and organics at midlatitudes on Mars).
If there is 0.1% organics this is well above the permitted level of contamination in the sample tubes of 10 ppb with 8.1 ppb likely achieved (Boeder et al., 2020, Mars 2020: mission, science objectives and build : table 6). However it would be optimal to do the tests with a clean sample of dirt.
Perchlorates also figure in the most developed non biological theory for the apparent detection of life. Quinn et al. in 2013 suggested that the perchlorates in the soil were decomposed through gamma radiation to hypochlorite (ClO -), trapped oxygen, and chlorine dioxide. Then the hypochlorite reacted with the 14C-labelled alanine to produce chloroalanine which then decomposed to produce the 14C-labelled CO2. (Quinn et al., 2013. Perchlorate radiolysis on Mars and the origin of martian soil reactivity). This didn’t explain everything and a follow up paper by Georgiou et al. filled out the picture some more but is still not a complete explanation (Georgiou, 2017. Radiation-driven formation of reactive oxygen species in oxychlorine-containing Mars surface analogues).
However there is another issue with replicating the Viking labeled release. Levin et al. found that the dirt samples were inactivated by being kept in darkness and at a temperature of at least 10°C for 141 sols (Viking 1 cycle 4) or 84 sols (Viking 2 cycle 5) at and above 10 °C
The fourth and final LR run at Viking site 1 (VL1-4) was on a sample collected from the same area that had produced the positive response in VL1-1. However, at the time of the injection for this run, the soil had been stored in the sample distribution box, in the dark, open to the martian atmosphere, but maintained at temperatures ranging between 10°C and 26°C for 141 sols. Two nutrient injections were made about 3 h apart, which allowed time to observe a response from the first injection before administering the second. Each injection resulted in a null response (Fig. 6). Apparently, long-term storage of an active soil at 10 - 26°C inactivates an initially active sample. This, again, is consistent with biology.
We would not expect to see such losses in terrestrial analog soils because these temperatures are within normal range for most terrestrial soils, even over long periods of time. On Mars, however, although soil may temporarily see 10°C or even higher temperatures during a normal diurnal cycle, at least during the warmer times of the martian year, the daily exposure to such elevated temperature would be brief; martian organisms would therefore be expected to be much more likely to succumb to long-term storage at 10 - 26°C than would terrestrial microorganisms. Thus, possibly stressed by long-term isolation from their natural environment at elevated temperatures, the putative martian organisms may have died.
...
Viking 2, cycle 4 (VL2-4) used the last of the four LR test chambers. Since nutrient solution was still available, an additional run was improvised whereby stored soil collected for VL2-4 was added on top of a previously tested soil sample. This VL2-5 soil was taken from the same area as that for VL2-4, but the soil had been held in the sample distribution box for 84 sols at approximately 10°C before the onset of VL2-5. As seen in Fig. 7, long-term storage of the sample used for VL2-5 totally inactivated the soil, producing a negative response.
(Levin et al., 2016, The case for extant life on Mars and its possible detection by the Viking labeled release experiment : 3 The Viking LR Data).
[ILLUSTRATION NEEDS PERMISSION (Levin et al., 2016, The case for extant life on Mars and its possible detection by the Viking labeled release experiment : Fig 6)]
Shows radioactivity of the headspace gases for the first 7 days after the injection of nutrients and water for Viking 1. The sample stored in darkness for two months, cycle 4,shows almost no radioactivity and is similar to the control, cycle 2, while cycles 1 and 3 show a similar pattern of rising radioactivity which starts to plateau by day 7.
The journey back from Mars is several months, and the samples would normally be kept in complete darkness for this time, even if the dirt is freshly collected just before the sample is loaded on the Mars Ascent Vehicle.
Whatever the active agent is, chemical or biological, we might need to arrange to try to keep it active during the journey back by simulating martian surface conditions during that time. Since we don't know why darkness inactivated the samples, this would need to be an informed guess, but we could perhaps add a small window to the sample container to let in some of the light from the sun to part of the dirt sample, if this is feasible and make sure it doesn't spend sustained periods of time at or above 10°C in the dark.
If it is life that caused the evolved gases for the Viking experiments, and it is no longer viable after the journey back to Earth,we need clean samples to be sure that any biosignatures we find come from Mars.
Then for the motivation for the dust, let's look at a few of the scenarios that have been suggested based on our discoveries about the knowledge gaps in SR-SAG2.
A scenario of propagating biofilm fragments from a more habitable past, and its relevance to Jezero crater
We looked above at four main findings in the 2015 review of SAR-SAG2 relevant to Jezero crater. In short they are:
- transport via the atmosphere from distant parts of Mars, for instance in dust storms.
- microhabitats that we can't see from orbit and many may be invisible even to rovers in situ
- biofilms able to make use of conditions that would otherwise be too inhospitable
based on all those
- maps from orbit only represent our current state of knowledge, subject to change
See above:
Combining these ideas we get an especially interesting scenario for Jezero crater which combines together three of those knowledge gaps, transport in the atmosphere, biofilms and microhabitats. Amongst several relevant discoveries, later research found small fragments of biofilm, thin layers of a microbial colony three hundredths of a millimeter thick, can travel 100 kilometers in daylight in the light martian winds before it is sterilized (Billi et al.., 2019, A desert cyanobacterium under simulated Mars-like conditions in low Earth orbit: implications for the habitability of Mars)
Text on graphic:
Areas of Mars could be colonized by biofilm fragments
May have formed in distant parts of Mars or a more habitable pastBlown in dust storms or at night
Annotations added to (Asma et al., 2022, (An Overview of Biofilm Formation - Combating Strategies and Mechanisms of Action of Antibiofilm Agents : Figure 1)
Mosca et al. suggest that a biofilm could still propagate on Mars in this way as complete biofilm fragments, even if local conditions don’t permit it to establish a biofilm today by slowly growing from a few microbes. All that is needed is that at some time in the past biofilms were able to form, propagating ever since then using these broken off fragments (Mosca et all., 2019, Over-expression of UV-damage DNA repair genes and ribonucleic acid persistence contribute to the resilience of dried biofilms of the desert cyanobacetrium Chroococcidiopsis exposed to Mars-like UV flux and long-term desiccation)
These authors don't look at transport at night (the winds are as strong at night as in daytime during martian dust storms) or in the dust storms which blocked out 97% of the UV for three weeks in the great dust storm of 2018. These could turn the survivable distance from 100 km to 1000s of kilometers.
How bouncing sand grains could enable microbes to travel great distances on Mars
The newer research also turned up a new way that microbes could be transported on Mars, in bouncing sand grains.
Text on graphic: Bouncing sand grains or propagules would travel 250 to 850 kilometers per day in a dust storm (at typical saltation speed of 3 to 10 meters per sec).
Sand grains on Mars of 500 microns diameter can bounce up to several meters with each bounce with a height of tens of cms.
A biofilm propagule this size covered in iron oxide microparticles for protection from UV could contain over 24 million microbes at 1 micron diameter.
Artist’s impression of a typical bounce based on figure 2b from (Giant saltation on Mars) superimposed on photograph of the top of a large sand dune taken by Curiosity on December 23, 2015 (NASA Rover's Sand-Dune Studies Yield Surprise)
Bouncing grains work differently in the low gravity and thing atmosphere of Mars. There are two wind speeds here, first the fluid threshold to get the grains into the air to start grains bouncing. Once the grains have started bouncing, the wind only needs to stay above the lower impact threshold to keep the bounces going after each impact. The impact threshold on Mars can be a tenth or less of the fluid threshold (Kok, 2010. Difference in the wind speeds required for initiation versus continuation of sand transport on Mars: Implications for dunes and dust storms : Figure 1). On Earth where the wind speeds can get fast enough to set the grains bouncing at only a little over the impact threshold, with a ratio of 0.82 for loose sand.
The wind adds energy to the grains with each bounce to compensate for the energy lost through impact with the surface. Kok et al. did a detailed analysis for Proctor crater. If the wind goes above the fluid threshold, which is enough to keep the dust grains bouncing once they start bouncing, then it will only take an ejection velocity of 3 meters per second or so or about 10 km / hour for the grains set into motion to keep bouncing which can be sustained by winds at a velocity of only 1 meter per second, or 3.6 km / hour. See (Kok, 2010. Difference in the wind speeds required for initiation versus continuation of sand transport on Mars: Implications for dunes and dust storms : Figure 2)
From experiments using simulated Mars gravity in parabolic flight (Kruss et al., 2019.Wind Erosion on Mars and other Small Terrestrial Planets : 4), a few dust grains may move at even lower wind speeds, with a measured threshold value of only ~0.7 m/sec or 2.6 km / hour (1.6 miles per hour) which agrees with one of the models of the motion of martian dust grains.
Experimenters have found the dust starts moving at very low wind speeds with other experiments, e.g. with crushed walnut shells to simulate low gravity, and this also fits with measurements of the pressure drop needed for the dust devils (Lorenz, 2023. Gentle Perseverance Lifts the Veil on Martian Dust : table 1)
This is also confirmed by the downdraft from Perseverance’s Ingenuity helicopter. This raised very tenuous dust clouds which can only be seen by enhancing the photographs. The clouds during take-off and landing consistent with that 0.7 m/second. However it also raised dust clouds during traverses while flying across the surface of Mars at a height of 5 meters above the surface which Lorentz et al. say suggests the dust can be lifted with a wind speed of only 0.3 meters / second (1 km / hour, 0.7 miles per hour).
These images are processed to bring out the moving dust in the images. The ingenuity helicopter is circled in each frame. The dashed orange arrow shows the direction of the winds as inferred from the movement of the cloud after it formed. The solid yellow arrow shows the direction of motion of the particles within the dust cloud
(Lemmon et al., 2022. Lifting and Transport of Martian Dust by the Ingenuity Helicopter Rotor Downwash as Observed by High-Speed Imaging From the Perseverance Rover : figure 10)
At such slow wind speeds, Ingenuity probably created the dust by breaking up clumps of very fine dust which then got caught up in the resulting winds, as the wind speeds were too low to start grains bouncing.
During traverses, dust lifting was observed from heights at which saltation was unlikely, suggesting the likelihood of break-up of dust aggregates and entrainment of the resulting dust.
(Lemmon et al., 2022. Lifting and Transport of Martian Dust by the Ingenuity Helicopter Rotor Downwash as Observed by High-Speed Imaging From the Perseverance Rover)
Curiosity was able to observe an active dune field close up, the Bagnold dune field. This had meter scale ripples and smaller centimeter scale ripples. The smaller ripples are also found on Earth but the larger ripples are unique to Mars:
The Bagnold dune field consists of fine to very fine sand, with minor but variable fractions of coarser particles near larger ripple crests. Many aspects of dune and superimposed ripple morphologies are recognizable from terrestrial settings. One exception is the prevalence of large ripples of meter-scale wavelengths without coarse, creep-limited grains covering their crests (Fig. 7), distinct from the smaller-scale impact ripples that are common to both planets
(Vasaveda, 2020. Mission Overview and Scientific Contributions from the Mars Science Laboratory Curiosity Rover After Eight Years of Surface Operations : 4.2)
Curiosity at the Gohabeb site in Bagnold sands. The image shows primary ripples which are meter scale and secondary ripples that are centimeter scale. The indentation close to the rover was created intentionally using Curiosity's wheel to "dig" it.
These ripples move slowly with the winds on Mars and Curiosity was able to observe particles moving from 0.5 to 3 mm in size. The sands were also enriched in nitrates and other chemicals that astrobiologists have said are of interest to habitability studies.
(Vasaveda, 2020. Mission Overview and Scientific Contributions from the Mars Science Laboratory Curiosity Rover After Eight Years of Surface Operations : Fig 7) : .
It found that particles did indeed start bouncing for wind speeds well below the fluid threshold, which obscured the mast cam. It found that even particles as large as 3 mm in diameter moved in the dune field.
Abundant grain and ripple motions were observed despite wind speeds that only rarely (if ever) exceed the fluid threshold friction speed required to initiate saltation. This led to the development of a new saltation model that accounts for the initiation and sustainability of low-flux sand transport with wind speeds below the fluid threshold but above the impact threshold (Sullivan and Kok 2017).
...
Similar to what was observed for smaller particles in the dune field, particles 0.5 to 3 mm in diameter also were observed to move in spite of modeled winds falling below theoretical thresholds. Baker et al. (2018a) argue that such observations highlight the need to broaden interpretive tools beyond classical transport models in order to account for gusts and alternate modes, such as drag-induced rolling and impact-driven creep.
(Vasaveda, 2020. Mission Overview and Scientific Contributions from the Mars Science Laboratory Curiosity Rover After Eight Years of Surface Operations : 4.2)
Other experiments show that though most microbes that get trapped in a dust grain are quickly destroyed by the shock of the impact bounces, a fraction of a percent remain viable after transport for the equivalent of hundreds of kilometers travel in bouncing sand grains (Finster, 2018, Wind-driven saltation: an overlooked challenge for life on Mars).
Martian life might also develop adaptations to resist the bounces, for instance coatings of iron oxide particles or chitin (an exceptionally hard material used in insect exoskeletons but also produced by lichens). See above:
The sand dunes may be of special interest for local microhabitats. Curiosity noticed a sudden drop in the water volume mixing ratio whenever it traveled over sand dunes which the team interpreted as evidence of water exchanging with the soil. It noticed this at three locations, the Rocknest dune, the Dingo gap dune, and the sand ripples at the Windjama site,
ILLUSTRATION NEEDS PERMISSION
Caption from original figure: "The sudden increase in H2O VMR as the rover leaves the sand areas, shaded in grey, is consistent with the existence of a local exchange of H2O between the soil and atmosphere."
(Martín-Torres et al., 2015. Transient liquid water and water activity at Gale crater on Mars : fig 2b).
We look at the potential habitability of these brines below in:
The sand dunes covered in the 2015 paper are all static (Vasaveda, 2020. Mission Overview and Scientific Contributions from the Mars Science Laboratory Curiosity Rover After Eight Years of Surface Operations : 4.1) but later on Curiosity was able to study the active Bagnold sand dunes (Vasaveda, 2020. Mission Overview and Scientific Contributions from the Mars Science Laboratory Curiosity Rover After Eight Years of Surface Operations : 4.2) .
Large active sand dunes on Mars move at a speed of about half a meter per year on average though with a lot of variation (Chojnacki et al., 2019. Boundary condition controls on the high-sand-flux regions of Mars) and press release (Stolte, 2019, On Mars, Sands Shift to a Different Drum)
. That's not for the ripples, that's the entire sand dune. Some of the faster moving dunes move 3 metes a year or more .
(Chojnacki et al., 2019. Boundary condition controls on the high-sand-flux regions of Mars : Figure 1(detail))
So, over time martian sand dunes can move hundreds of meters which can churn the surface and return large amounts of material to the surface. This also provides chemical redox gradients which life can use as a source of energy, for instance surface layers of sand dunes are superoxygenated while the slowly moving sand dunes constantly bring subsurface reducing layers to the surface (Fisk, et al., 2013. Habitability of Transgressing Mars Dunes) .
Curiosity found that all the sands were enriched in nitrates, sulfates, hydrogen sulfides, hydrogen,and other chemicals which have been mentioned by astrobiologists as of interest for habitability. These are the figures for the Bagnold sand dunes for the most abundant chemicals found in the Bagnold scooped samples in order of abundance. All of these are of interest for habitability:
Cl-ClO4- 731 ± 199 ppm
Cl-HCl: 614 ± 378 ppm
NO3: 277 ± 72 ppm
H2S: 47 ± 16 ppm
H2 27 ± 8 ppm
CO 17 ± 4 ppm
SO3 1.2 ± 0.3 ppm
(Stern, et al., 2018. Major Volatiles Evolved From Eolian Materials in Gale Crater : table 1)
I have been unable so far to find information about whether Curiosity also detected subsurface brines for Bagnold sands. But since Curiosity found the brines in the static sand dunes and the amounts of perchlorates in Bagnold sands are similar to the static sand dunes, one would expect the brines to be similar too.
If the brines Curiosity found are indeed habitable to biofilms, these seem to have potential for localized microbial oases that may form temporarily then be altered or destroyed. Then life could move from one oasis to another in biofilm fragments up to 3 mm in diameter, transported along with the moving sand grains.
Scenario of a microbially diverse Mars with microbial oases with unique species analogous to the Galapagos finches
It is likely to be a major challenge for even native martian microbes to travel in its dust storms. One scenario is that while some species of life might be found almost everywhere globally on Mars, there could be other species that colonized more widely on Mars hundreds of millions of years ago when it was more habitable and are now confined to local oases. They could have diverged significantly through evolution since then.
This suggests an analogy with islands in the Pacific. If microbial life can be transferred in dust storms - but perhaps rarely and only some species make it, perhaps every 100,000 to 500,000 years, while other species were only able to travel about Mars millions of years ago, Mars could have islands of habitability similarly to the Galapagos islands. These microbial oases could have locally evolved niche species living alongside the other species that can travel more easily around modern Mars and are shared with other oases. We rarely encounter this with microbes on Earth but some species of fresh water diatoms are local to particular continents because they can’t travel across the seas easily (Spaulding et al., 2010. Diatoms as non-native species).
Text on graphic: In a best case for astrobiology, worst case for planetary protection assessment – Mars could have microbial islands of diversity as distinctive as the Galapagos island finches are for birds
It's a common theme in the astrobiological literature that life on Mars could survive in oases such as caves, ice based habitats and salts.
A powerful theme that permeated the conference is that the key to the search for martian extant life lies in identifying and exploring refugia (“oases”), where conditions are either permanently or episodically significantly more hospitable than average.
Based on our existing knowledge of Mars, conference participants highlighted four potential martian refugium (not listed in priority order): Caves, Deep Subsurface, Ices, and Salts.
(Carrier et al, 2020, Mars Extant Life: What's Next? Conference Report (html) : 804)
It could be that these z have developed different species, for instance each cave may have evolved different species, genera or even right up to novel domains of life on Mars that may have potential to live in many locations but have just not got to all the most recent habitats that have formed temporarily in the hostile surface conditions on Mars.
When we look for the most challenging scenarios for planetary protection, this patchy distribution could also happen with alternative forms of biology like mirror life. Mars could have a relic population of mirror life that does well in terrestrial conditions but is not able to transport easily in the dust storms and only lingers in a few remaining surface niches.
Mars has conditions that might favour multiple origins of life, for instance early life there might have evolved independently in Hellas basin and in the northern sea. Perhaps multiple origin life has persisted through to the present day, or new forms of life independently evolved from time to time in biologically isolated conditions in caves or impact melt lakes and so on.
These could be variations on DNA with extra bases similarly to Hachimoji DNA, or extra amino acids terrestrial life never uses, or a novel codon table (translation table from genetic code to amino acids), leading to novel enzymes and proteins terrestrial life can never make. It could include mirror life.
More speculatively evolution on Mars could have explored RNA world life based on ribozymes instead of ribosomes, without DNA, or it could have explored novel backbones for the genetic informational polymers like PNA, TNA, and other XNAs. It could have explored hybrid or hotchpotch life, either two strands with different backbones interweaved or a strand that starts with one backbone and switches to another part way which is possible for early life especially. Or it could have explored life that has both ordinary and mirror DNA in the same organism, with enzymes similar to Joyce’s enzyme which works with DNA of the opposite symmetry. There are numerous possibilities explored by experts on the topic.
Text on graphic: Example scenario of multiple origins of life on Mars which would make the planet of enormous interest for astrobiology … but makes planetary protection very complex
Early Mars had relatively biologically isolated seas which could favour multiple origins of life.
DNA based life similar to terrestrial biology
DNA with
- extra bases like Hachimoji DNA
- extra amino acids
- new ways to interpret and error correct the DNA
- leading to novel enzymes and proteins terrestrial DNA can never make.
Each lake exploring a different “dialect” or “language” of DNA and slightly different vocabularies of bases and amino acids.Mixed symmetry life using an analogue of Joyce’s enzyme.
Mirror life, PNA life, RNA life
Hybrid life containing elements from different biochemistries such as TNA, HNA, GNA and other XNAs
Early Mars has relatively biologically isolated seas which could favour multiple origins of life.
Graphic illustrates the likely quantity of water on early Mars and how it might have been distributed 4.3 billion years ago, Credits: NASA/GSFC
Graphic from press release: ( NASA, 2015, NASA Research Suggests Mars Once Had More Water Than Earth’s Arctic Ocean)
Paper: (Villanueva et al., 2016, Strong water isotopic anomalies in the martian atmosphere: Probing current and ancient reservoirs)
So the situation on Mars could potentially be very complex in worst case scenarios for achieving a rapid understanding of the Martian biosphere, though best case scenarios for interesting astrobiology on Mars.
The situation could also be similar for abiotic, prebiotic or early life which we explore below in:
- Valuing any martian uninhabited habitats: how discovery of prebiotic chemistry or the first steps of a second genesis of early life could revolutionize understanding of life's origins
- Potential of Mars for a variety of short lived and longer lived isolated habitable "oases" in caves, surface isolated oases and so on where prebiotic, early life or abiotic chemistry might explore different pathways
How Curiosity's ultracold early morning brines could be an oasis for native biofilms that retain water to midday
Mosca's biofilm scenario is already plausible for somewhere on Mars, so long as there are microhabitats that life can exploit as oases (refugia) on the surface. If these biofilms spread in the wind they may sometimes reach Jezero crater from far away on Mars even if they can't grow in Jezero crater, and so could be returned in the dust and dirt possibly still viable. See above:
However it could also be a plausible scenario even for Jezero crater if we can find a plausible oasis for the biofilms there. We have had many surprises (e.g. the Phoenix leg droplets (Rennó et al., 2009, Possible physical and thermodynamical evidence for liquid water at the Phoenix landing site), so
- it's possible we find a new type of oasis that nobody has thought of.
- It's also possible martian life found a way to survive on Mars that nobody has thought of yet.
But we can sketch out one plausible type of oasis that we may find in places in Jezero crater based on what we know already.
Curiosity found liquid water in the salts that take up water at night - on the surface through to 6 am on the same day that it measured surface brines for the last time in the year, it registered a midday temperature of 15 °C (Martín-Torres et al., 2015, Transient liquid water and water activity at Gale crater on Mars : figure 3a and 3c) Those brines are habitable but too cold for terrestrial life at -73°C at 6 am on that day. But could life somehow retain that water through to warmer conditions?
Curiosity did register water close to the surface and at a temperature well above 0°C around day 300. It also found that water formed closer to the surface when the daily variation of temperature was highest.
[ILLUSTRATION NEEDS PERMISSION]
Notice that for a few sols around sol 300, liquid brines were detected at a depth of about a centimeter below the surface with a temperature well above 0°C (shown by the red colour coding). and with a daily ground surface temperature range of 99°C.
These are ideal conditions for a biofilm to retain enough water for the brines to still be habitable later in the day.
Original figure caption:
Estimated depth of the water layer as derived from DAN subsurface measurements compared with the amplitude of the ground surface diurnal temperature variation measured by REMS.
The depth of the water layer detected by DAN shows anti-correlation with the amplitude of the diurnal variation of the ground temperature, and thus with the TI of the soil, suggesting a role of the depth temperature profile in the mobilization of perchlorates and the stability of the hydrated states
(Martín-Torres et al., 2015, Transient liquid water and water activity at Gale crater on Mars : Supplementary data: figure 8)
The team also spotted corrosion of Curiosity's wheels which they consider could be due to interaction with perchlorate ions and byproducts. This suggests liquid perchlorate solutions close enough to the surface to be disturbed by Curiosity's wheels.
[ILLUSTRATION NEEDS PERMISSION]
The image (of about 3 cm diameter) shows not only a large crack in the wheel but also a pattern of distributed sub-millimetre sized blisters in the vertical wall of the T-print of the wheels which cannot be attributed to rock scratching.
Laboratory experiments show that Al foil contacted with a drop of very diluted CuCl2 brine (10 mM) for only 10 min, show within minutes 10-50 um-sized blisters with Cu deposits produced after Al pitting corrosion (similar to those circled in red in the figure).
The anodized protection of the aluminium wheels may protect just the upper few nm layers but the abrasion may wear out the external protecting layer and expose the internal Al to corrosion.
(Martín-Torres et al., 2015, Transient liquid water and water activity at Gale crater on Mars : Supplementary data: figure 9)
These brines are an example of the SR-SAG2
“Brine-related Liquid water in deliquescing salts, in channels within ice, on the surface of ice, within salt crystals within halite or other types of ‘rock salt’”
(Rummel et al., 2014. A new analysis of Mars “special regions”: findings of the second MEPAG Special Regions Science Analysis Group (SR-SAG2) : 904)
Nilton Rennó, an expert on Mars surface conditions, who was part of the team that discovered the Phoenix lander drops, principal investigator for Phoenix and also runs the REMS weather station on Mars for Curiosity, suggested in an interview that microbes might use biofilms to inhabit these brines
"Life as we know it needs liquid water to survive. While the new study interprets Curiosity's results to show that microorganisms from Earth would not be able to survive and replicate in the subsurface of Mars, Rennó sees the findings as inconclusive. He points to biofilms—colonies of tiny organisms that can make their own microenvironment.
(Pires, 2015, Mars liquid water: Curiosity confirms favorable conditions)
Rettberg et al. asked two questions. They asked if multispecies colonies can form biofilms to help them tolerate environments they couldn't survive in individually.
They also asked if organ isms can replicate on Mars if the temperature and water activity separately reach levels suitable for life, but not both at once, for instance by storing water when it is cold and then using it to replicate as the brines warm up. See (Rettberg et al., 2016. Planetary protection and Mars special regions—a suggestion for updating the definition : section 3.1 ).
Rettberg et al. raised this as a question in 2016, to paraphrase (Rettberg et al., 2016. Planetary protection and Mars special regions—a suggestion for updating the definition : section 3.1)
- Can multispecies colonies form biofilms to help them tolerate environments they couldn't survive in individually?
- Can organisms replicate there if the temperature and water activity separately reach levels suitable for life, but not both at once, for instance by storing water when it is cold and then using it to replicate as the brines warm up?
These would likely be a mix of many species working together for extra resilience like the grit crust in the Atacama desert (Jung et al., 2022, The grit crust: A poly-extremotolerant microbial community from the Atacama Desert as a model for astrobiology)
If we combine all this with recent research that terrestrial mosses may be good Mars analogue organisms, it leads to possible scenario where Mars has moss like organisms that absorb water fast in the early morning when Curiosity found ultra cold salty water even on the surface at -73°C - and retain it even through to midday on the same day when temperatures reached over 15°C?
These biofilms could include mosses that might take up water when its very cold just passively - and then they might retain it through to daytime when temperatures go up to above 15°C in Jezero crater. Huwe et al. tested a moss Grimmia sessitana collected in the alps in a terrestrial Mars simulation chamber in 2019 (Huwe et al., 2019. Mosses in low Earth orbit: implications for the limits of life and the habitability of Mars) and also tested in BIOMEX, the Mars simulation experiment that was attached to the outside of the ISS (De Vera et al., 2019, Limits of life and the habitability of Mars: the ESA space experiment BIOMEX on the ISS) though I can't find the results of that experiment.
Huwe et al. tested rapid day night cycles from -25°C to 60°C which had no effect (Huwe et al., 2019. Mosses in low Earth orbit: implications for the limits of life and the habitability of Mars). They didn’t need to cycle it all the way down to below -70°C as it has already been shown to be able to survive immersion in liquid helium at only 0.65°C unharmed (Lenne et al., 2010, Freeze avoidance: a dehydrating moss gathers no ice). They found that vacuum and the Mars like atmosphere had no effect on it. (Huwe et al., 2019. Mosses in low Earth orbit: implications for the limits of life and the habitability of Mars)
In this scenario, Mars could have microscopic moss like plants, which can absorb water rapidly, in seconds, and retain it for a long time The desert moss Syntrichia caninervis which is found in desert biocrusts throughout the world uses microgrooves rather than micropores, with an “upside-down” water collection system that collects water droplets which condense onto microgrooves within its leaf hair points and it rapidly funnels those down to the plant below (Tao et al., 2012, Effects of leaf hair points of a desert moss on water retention and dew formation: implications for desiccation tolerance).
Video: Demystifying desert moss hydration
The leaf hairs
- absorb water through capillary action which would otherwise evaporate, which slows down evaporation
- make the mosses more reflective, reflecting away sunlight
- expand as they hydrate and so form a 3D mesh which blocks evaporation
(Tao et al., 2012, Effects of leaf hair points of a desert moss on water retention and dew formation: implications for desiccation tolerance)
This reduces evaporation from the hydrated moss for as long as it has high water content.
Those microgrooves are like a biological analogue of the Atacama salt and gypsum pillars micropores. So - might not martian life have developed similar structures over billions of years of evolution on Mars? Microgrooves or micropores, or some other structures optimized to collect and retain water in cold conditions, passively, mechanically when it is too cold for metabolic processes.
More speculatively, some mosses can open and close pores (stomata) like plants (Chater et al., 2011, Regulatory mechanism controlling stomatal behavior conserved across 400 million years of land plant evolution). I couldn’t find a paper about terrestrial biocrust doing this, but could a biofilm be covered by a martian organism that evolved pores that close in daylight like the stomata of cactuses to hold in the water? This is interesting because the stomata close automatically as they dry out, and so could do that even when the moss is dormant, too cold for its metabolism to operate.
Text on graphic: Guard cells (swollen) Stoma opening.
Guard cells (shrunken) Stoma closing
(Opening and closing of stoma)
Exploring the potential for microbes to live at ultracold temperatures well below -20° C on Mars
Another way that martian microbes could use the ultracold brines on Mars is if they are able to live at very low temperatures. The usual -20°C lowest limit for replication isn’t well determined even for terrestrial life. This could be combined with the adaptations of the previous section. The microbes don't need to be active right down to -70°C. If the biofilms can retain some water through to warmer conditions then there will be strong evolutionary pressure for microbes that can use the retained water at ultracold temperatures, in daytime for instance even at -30°C or -40°C if that is biologically possible. No matter how effective the biofilm is at retaining water, any microbes able to use it at temperatures well below -20°C could grow for more of the day and have more habitable days per year.
To paraphrase (Rettberg et al., 2016. Planetary protection and Mars special regions—a suggestion for updating the definition : section 2.1) again:, :
- How strict is the lowest temperature for replication for Earth life? Have the experiments exploring the lower temperature limits of replication been carried out over long enough timescales to study extremely slowly replicating organisms?
It is hard to study replication at such low temperatures because of the long generation times. Experiments with yeast show doubling at -18 °C, with a doubling time of 30 days. One experiment showed ammonia oxidation at -32 °C sustained for 300 days, the duration of the experiment. Since cell division would be so very slow at those temperatures, the researchers couldn’t tell whether this is just maintenance metabolism, or whether it actually did support very slow cell division (Rummel et al., 2014. A new analysis of Mars “special regions”: findings of the second MEPAG Special Regions Science Analysis Group (SR-SAG2))
Chaotropic agents can help microbes be active at lower temperatures by disrupting the hydrogen bonding of water molecules with each other. Common examples include ethanol, urea, butanol, etc. The Mars surface has many chaotropic agents which could reduce the minimum temperatures for cell division, including MgCl2, CaCl2, FeCl3, FeCl2, FeCl, LiCl, chlorate, and perchlorate salts (Rummel et al., 2014. A new analysis of Mars “special regions”: findings of the second MEPAG Special Regions Science Analysis Group (SR-SAG2)).
Typically, these chaotropic agents reduce the lowest temperatures for cell division for many microbial species by 10 °C to 20 °C. However Rummel et al couldn't find any experiments testing their action at temperatures low enough to reduce the lowest temperature limit for cell division for life (Rummel et al., 2014. A new analysis of Mars “special regions”: findings of the second MEPAG Special Regions Science Analysis Group (SR-SAG2) : 897) . The lower the temperature, the harder it is to do the experiments to test the effect on cell division, due to the longer replication time of life at such low temperatures.
Even -40 °C (i.e. a 20 °C reduction below -20 °C ) is a long way from the inferred temperature of -73 °C for the brines unmodified by biofilms with water activity 0.525 (Martín-Torres et al., 2015. Transient liquid water and water activity at Gale crater on Mars).
Could Martian life could exploit lower temperatures than terrestrial life?
One study did find that chaotropic agents can increase the ability of microbes to survive freezing to -80 °C with no loss of viability, when frozen for 24 hours, compared with 60% loss of viability without. The test used the microbial propagules of xerophilic fungi (low water activity) (Chin et al., 2010. Solutes determine the temperature windows for microbial survival and growth) but didn’t test for cell division at low temperatures.
Some microbes are known to release ice-binding proteins (IBPs) slowly into the surrounding environment which prevent the formation of ice. Garcia-Descalzo et al have proposed that these could act as cold-brine shock proteins’’ that could help to make the microenvironment around the microbes more habitable. They also wonder whether bacteria unable to achieve cell division in cold and salty conditions could, without reproducing, slowly modify them to become more habitable (Garcia-Descalzo et al., 2020. Can Halophilic and Psychrophilic Microorganisms Modify the Freezing/Melting Curve of Cold Salty Solutions? Implications for Mars Habitability). They have not yet confirmed this experimentally but so far have only studied one species of terrestrial microbe, Rhodococcus sp. JG3 (Garcia-Descalzo et al., 2020. Can Halophilic and Psychrophilic Microorganisms Modify the Freezing/Melting Curve of Cold Salty Solutions? Implications for Mars Habitability)
More radically, Dirk Schulze Makuch et al suggested that native life on Mars might have evolved to use the cold brines on Mars with a novel cold adapted biochemistry, using perchlorates or hydrogen peroxide internally, in place of the chloride salts in our cells (Schulze-Makuch et al.. 2010. A perchlorate strategy for extreme xerophilic life on Mars).
First, with water mixed with hydrogen peroxide in the cells, cells could remain metabolically active down to -56°C (217°K). Then secondly, a mix of two salts typically has a lower freezing point than either individually. If the cells used a mix which includes perchlorates internally, the limit might be reduced further, to -70°C which is similar to the temperature inferred for the brines observed by Curiosity y (Schulze-Makuch et al.. 2010. A perchlorate strategy for extreme xerophilic life on Mars).
Houtkooper et al suggest this novel biochemistry as a possible explanation for some of the puzzling Viking results. The organics might react with the hydrogen peroxide and decompose easily, when heated or even when hydrated with too much water. This could be a challenge for detecting the organics from alien life with such reactive internal chemicals (Houtkooper et al., 2006. A po sible biogenic origin for hydrogen peroxide on Mars: the Viking results reinterpreted ).
Also, the water activity may not be such a fixed limit. Microbes in a deep-sea hypersaline anoxic basin (DHAB) can flourish at a water activity as low as 0.4, well below the inferred value for Curiosity of 0. 525 (Martín-Torres et al., 2015. Transient liquid water and water activity at Gale crater on Mars), or the usual limit of 0.6 (Steinle et al, 2018) (Merino et al, 2019: Salinity and Water Activity)
The brines in Gale crater, and probably also in Jezero crater pose a major challenge to life and it would be hard for terrestrial life to exploit them. They are likely safe from contamination with our current Mars rovers that are not likely to be able to contaminate them with terrestrial biofilms.
But as we've seen, it’s possible that native life has learnt to live in them, either through biofilms, or by adaptations to ultra low temperatures or both.
Martian perchlorates harm some microbes but are a "giant dinner plate" for others in words of Cassie Conley, 2nd NASA planetary protection officer
If the brines can’t be modified by biofilms to retain the water through to warmer conditions, the low temperature is a significant barrier to terrestrial life. But as we saw in the last section, it's possible it could be much less of a barrier to some martian life. See:
The brines in Gale crater, and probably also in Jezero crater pose a major challenge to life and it would be hard for terrestrial life to exploit them. They are likely safe from contamination with our current Mars rovers that are not likely to be able to contaminate them with terrestrial biofilms.
But it’s possible that native life has learnt to live in them, either through biofilms, or by adaptations to ultra low temperatures or both.
The low temperature brines found by Curiosity remain liquid because of a high concentration of perchlorates. So, could Martian life make use of perchlorates in such high concentrations? These concentrations aren’t found on Earth and Heinz et al, say that there has been little by way of studies to find the limits for concentrations for terrestrial life (Heinz et al., 2020. A new record for microbial perchlorate tolerance: fungal growth in NaClO4 brines and its implications for putative life on Mars). They report the discovery of fungi that can grow in a 23% weight by weight solution of sodium perchlorate, or 2.4 M(Heinz et al., 2020. A new record for microbial perchlorate tolerance: fungal growth in NaClO4 brines and its implications for putative life on Mars)which corresponds to a water activity of about 0.8 (from figure 5 of (Toner et al., 2016. Water activities of NaClO4, Ca (ClO4) 2, and Mg (ClO4) 2 brines from experimental heat capacities: water activity> 0.6 below 200 K).
Heinz et al suggest:
It is plausible to assume that putative Martian microbes could adapt to even higher perchlorate concentrations due to their long exposure to these environments occurring naturally on Mars, which also increases the likelihood of microbial life thriving in the Martian brines.
(Heinz et al., 2020. A new record for microbial perchlorate tolerance: fungal growth in NaClO4 brines and its implications for putative life on Mars)
It is a common misconception about Mars - that the perchlorates make it uninhabitable. It's the opposite. For some microbes yes, they make it less habitable but for others they make Mars MORE habitable.
Cassie Conley put it like this in 2015.
The salts known as perchlorates that lower the freezing temperature of water at the R.S.Ls, keeping it liquid, can be consumed by some Earth microbes. “The environment on Mars potentially is basically one giant dinner plate for Earth organisms,” Dr. Conley said.
(Chang, 2015, Mars Is Pretty Clean. Her Job at NASA Is to Keep It That Way)
There are many reports of perchlorate reducing microbes from perchlorate contaminated soils. However they also occur naturally in some places that are naturally enriched in perchlorates (Lynch et al., 2019. Evidence for Biotic Perchlorate Reduction in Naturally Perchlorate-Rich Sediments of Pilot Valley Basin, Utah)
If there is life on Mars, either the native martian life actually uses it, or it is very resistant to oxidants which it has to be anyway with perchlorates and a very oxidising surface. Interestingly the microbe from the Canadian permafrost that the Biological Safety Report missed is actually able to grow in 10% by weight perchlorate solution.
Furthermore, our results show the development of cohesive biofilms in perchlorate-rich media (Fig. 4A)
(Heinz, 2019, Bacterial Growth in Chloride and Perchlorate Brines: Halotolerances and Salt Stress Responses of Planococcus halocryophilus))
See also
Surface salts such as perchlorates are the only priority sample return mentioned by the"Extant life on Mars - What's Next?" conference
The report of the 3 day conference on the potential for present day life on Mars, held in Carbland New Mexico on November 5-8 mentions only one specific suggestion for a Mars sample return to look for present day life. It suggests that salts are a prime target both for their preservation potential for recent life and as a habitat for present day life. It specifically mentions perchlorates as a potential habitat.
If life exists today on Mars, or existed in the recent past, microscopic examination of such evaporite minerals and their fluid inclusions might offer a straightforward and realistic way to confirm its existence, while spectroscopic analyses may uncover biochemicals that represent biosynthetic or breakdown products of life.
Moreover, having these features as potential targets for future Mars Sample Return efforts would allow for potentially concentrated organics and extant life analyses within its mineral structure.
Salts and brines containing concentrated dissolved solutes and gas pockets could serve as energy and nutrient resources for life (e.g., perchlorates, nitrates, sulfates, organics, methane), with near-surface evaporites additionally providing access to sunlight for potential phototrophic activities.
Consequently, when considering NASA’s current mission concepts, evaporitic environments at the surface and near subsurface offer targets that are likely to be readily accessible to robotic exploration.
(Carrier et al, 2020, Mars Extant Life: What's Next? Conference Report (html) : 797)
How low temperatures, dust, and shadows could protect native microbes against harm from UV activated perchlorates and help them survive travel for long distances in dust storms
One experiment on the effect of UV on perchlorates in the dirt often gets mentioned but it's results were inconclusive and doesn't count against perchlorates as a habitat for native martian life. They only tested one species, b. subtilis. They only tested it at 25°C and 4°C when the effect was much less:
The chemical nature of this bacteriocidal effect is confirmed by carrying out the experiment at 4 °C, when the loss of viability is over ten times lower than at 25 °C, suggesting that lower temperatures lower the rate of the chemical reaction or the diffusion of activation products and reduce the rate of bacteriocidal effects. Nevertheless, the effect is still observable. The average surface temperature on Mars is approximately 218 K (−55 °C), however the Mars Exploration Rover Opportunity measured a daily maximum of 295 K (21.85 °C. Therefore, we would expect a range of reaction rates varying with latitude and time of day.
(Wadsworth et al., 2017. Perchlorates on Mars enhance the bacteriocidal effects of UV light))
- typical temperatures on Mars are below 0°C when perchlorates are far less active. The experimenters didn't test UV irradiated perchlorates with polyextremophiles that might be more resilient. Native life is likely to be more resilient to oxidants than terrestrial extremophiles.
They also found it was reduced by mixing the microbes with rock. But this is the normal scenario on Mars:
The effect is less pronounced within the rock analogue system likely caused by screening within the rock, which reduces the penetration of UV radiation compared to the liquid system.
A few microns of dust are enough to block UV completely. Then finally, biofilms screen out UV and would also protect microbes against oxidants.
The rock analogue system consisted of:
sintered discs of silica grains commercially produced to give a pore size of 100 - 160 µm
Billi et al found that their fragment of a biofilm could survive for 8 hours of daylight traveling 100 km - not tested with perchlorates but biofilms can protect microbes from oxidants (Dried biofilms of desert strains of Chroococcidiopsis survived prolonged exposure to space and Mars-like conditions in low Earth orbit. : 8-9). Billi et al. suggest
… Our findings support the hypothesis that opportunistic colonization of protected niches on Mars, such as in fissures, cracks, and microcaves in rocks or soil, could have enabled life to remain viable while being transported to a new habitat
(Billi et al.., 2019, A desert cyanobacterium under simulated Mars-like conditions in low Earth orbit: implications for the habitability of Mars).
Also, dust storms would reduce UV reducing this effect and increase the survival times for individual microbes. Curiosity was able to give direct observations of surface UV during the 2018 global dust storm, and found that it fell by 97% at the start of this storm, and remained at similar low levels for about three weeks (solar longitude 195 to 205)
UV measurements by upward pointing photodiodes on the REMS instrument suite on Curiosity. The UV fell by 97% at the onset of the dust storm (Smith et al., 2019, The Mars Global Dust Storm of 2018 : Figure 5)
Even during the dust storms, the wind speeds continue at night at 3-4 meters per second increasing to 10 meters per second or more in the day time … at least as measured from the Insight lander for the dust storm in 2019. Before the dust storms, the night wind speeds were above 5 meters per second for most of the night, increasing to a maximum of around 10 meters per second just before dawn at around 4 am. So there seems significant potential for transport of biofilms for large distances at night.
Blue dots show the wind speeds 5 days before the 2019 dust storm as measured from the Insight lander site, about 5 meters per second at night, increasing to 10 meters per second in the middle of the day.
Red dots show the wind speeds 5 days after onset which range from around 3 meters per second most of the night to above 10 meters per second in the middle of the day.
(Viúdez-Moreira et al., 2020, Effects of a large dust storm in the near-surface atmosphere as measured by InSight in Elysium Planitia, Mars : figure 5)
So during the dust storms there would be little by way of UV in daytime and none at night. Biofilms could travel a long way in the storms traveling day and night for several weeks. This could extend Billi et al.'s 100 km to 1000s of kilometers.
This suggests a scenario where martian biofilms hop from one microhabitat to another a few tens of kilometers at a time, maybe even hundreds to thousands of kilometers on rare occasions, similarly to the way desert nomads use oases to cross deserts.
If so Perseverance might detect life that is in process of hopping from one oasis to another even if it hasn't found the oases themselves (unlikely to find the oases without in situ life detection).
Hardy martian spores or propagules evolve to resist UV, with extra coatings and other strategies
It's also possible that native martian life has developed special adaptations to protect itself from UV. For instance spores with extra coatings, or special biomaterials - e.g. it could cover itself with iron oxide particles for protection from UV, and from perchlorates, chlorates and chlorites, or it could develop novel biomaterials, for instance based on chitin like the exoskeleton of an insect (lichens are able to produce chitin).
FIXCITES
Spores are already protected from reactive chemicals by multiple coat and crust layers (Cortesão et al., 2019. Bacillus subtilis spore resistance to simulated Mars surface conditions ) . This makes them far more resistant to oxidizing agents, bactericidal agents, chlorites, hypochlorites, etc., than vegetative cells (Sella et al., 2014. Life cycle and spore resistance of spore-forming Bacillus atrophaeus). Spores are also more UV resistant than vegetative cells. Some terrestrial spores can withstand many hours of UV radiation on Mars, including one strain still viable after 28 hours of simulated direct UV radiation in Mars simulation surface conditions (Galletta et al., 2010. Bacterial survival in Martian conditions). This helps protect them from high levels of UV which they encounter high in Earth’s atmosphere and in mountainous and polar regions.
However, after billions of years of adaptation in the harsh conditions on Mars, any Martian spores could be more protected, with more coat and crust layers than terrestrial spores, and resist UV for even longer than those 28 hours.
Martian life might also develop colonial ways of surviving in dust storms in the larger clusters or aggregates of microbes like the terrestrial analogues described by the Space Studies Board review
In addition, for microbes growing not as single cells but as tetrades or larger cell chains, clusters, or aggregates, the inner cells are protected against ultraviolet radiation. Examples are methanogenic archaea like Methanosarcina, halophilic archaea like Halococcus, or cyanobacteria like Gloeocapsa.
(SSB, 2015. Review of the MEPAG report on Mars special regions : 12)
Perhaps Martian microbial life might also evolve larger bacterial fruiting bodies similarly to those of the myxobacteria (“slime bacteria”). These have some bacteria that altruistically develop into non reproductive cells to protect the spores inside (Muñoz-Dorado et al., 2016: Fig 1) .
Simple multicellular martian life could reproduce by just breaking apart in dust storms (fragmentation). Fungi are able to reproduce from fragments of their filaments (hyphae). Red algae (rhodophyta) also often propagate in the same way. Their propagules are fragments of the parent plant which break off from the main body (thallus) and give rise to a new individual (Cecere et al., 2011). The evolution could begin with fragments broken off in winds due to the impact splashes of the half millimeter sand grains bouncing on the dunes of the previous section and the natural movements of the dunes. Bacteria might create propagules extended above the surface that get torn off by stronger winds in dust storms or dust devils.
A half millimeter diameter propagule, or 500 microns in diameter, typical in size for saltation on Mars (Kok, 2010:fig.3b) can contain many spores. At a maximal packing density of , which is the densest packing density possible for congruent spheres (Hales, 1998) (Hales et al., 2017), it can contain
=
= 24. 2 million spherical spores or microbes in dormant state at 1 micron diameter.
As with the biofilm fragments, larger propagules could help martian life to get off to a head start in an environment where a single microbe might not be able to survive.
The fruiting bodies might evolve extra protection against UV, perhaps an agglutinated external cyst of iron oxides to protect themselves from UV held together by secreted organics similarly to the external cysts of foraminifera (Heinz et al., 2005).
Speculating further, Martian life might also perhaps evolve specialized biological materials with much stronger protective layers than iron oxide to protect themselves from both UV and from damage from impacts during the saltation bounces. To take one very speculative example [new]: chitin is an essential component of the cell walls of fungi such as Aspergillus (Brauer et al., 2023) and the fungal component of lichens (Lenardon et al., 2010). It’s the same material insects use for their exoskeletons and jaws. Chitin has a Mohs hardness of 7 – 7.5 (Zhang et al., 2020) similar to quartz (King, n.d.). Chitin nanofibers have a Young’s modulus of elasticity of more than 150 GPa (Vincent et al., 2004), higher than copper or titanium alloy and not far below wrought iron or steel (Engineering ToolBox, 2003). There might be a strong evolutionary pressure on Martian propagules to evolve chitin materials for protection (or some similarly hard native polymer) perhaps as nanofibers in the biofilm. These might help protect the propagules as they bounce over the Martian surface in the winds and also help block out the UV.
Nilton Rennó's cold brine inhabiting biofilm scenario as just one potential oasis in Jezero crater which could have surprises
This biofilm scenario is already plausible for somewhere on Mars, so long as there are microhabitats that life can exploit as oases (refugia) on the surface. If these biofilms are spreading in the wind they may sometimes reach Jezero crater from far away on Mars.
However it could also be a plausible scenario even for Jezero crater if we can find a plausible oasis for the biofilms there. We have had many surprises (e.g. the Phoenix leg droplets (Rennó et al., 2009, Possible physical and thermodynamical evidence for liquid water at the Phoenix landing site), so
- it's possible we find a new type of oasis that nobody has thought of.
- It's also possible martian life found a way to survive on Mars that nobody has thought of yet.
But we can sketch out one plausible type of oasis that we may find in places in Jezero crater based on what we know already.
One idea is Nilton Rennó's suggestion that a biofilm could make the brines Curiosity found habitable. This was another surprise discovery on Mars. Curiosity found liquid water in the salts that take up water at night - on the surface through to 6 am on the same day that it measured surface brines for the last time in the year, it registered a midday temperature of 15 °C (Martín-Torres et al., 2015. Transient liquid water and water activity at Gale crater on Mars : figure 3a and 3c)
Those brines are habitable but too cold for terrestrial life at -73°C at 6 am on that day. But could life somehow retain that water through to warmer conditions?
These brines are an example of the SR-SAG2
“Brine-related Liquid water in deliquescing salts, in channels within ice, on the surface of ice, within salt crystals within halite or other types of ‘rock salt’”
(Rummel et al., 2014. A new analysis of Mars “special regions”: findings of the second MEPAG Special Regions Science Analysis Group (SR-SAG2) : 904)
Nilton Rennó is an expert on Mars surface conditions, who was part of the team that discovered the Phoenix lander drops, principal investigator for Phoenix and who also runs the REMS weather station on Mars for Curiosity. He is co-author of a major review of the current evidence for water and brines on Mars (Martínez et al, 2013, Water and brines on Mars: current evidence and implications for MSL)
Nilton Rennó i suggested in an interview that microbes might use biofilms to inhabit these brines
"Life as we know it needs liquid water to survive. While the new study interprets Curiosity's results to show that microorganisms from Earth would not be able to survive and replicate in the subsurface of Mars, Rennó sees the findings as inconclusive. He points to biofilms—colonies of tiny organisms that can make their own microenvironment.
( Pires, 2015, “Mars liquid water: Curiosity confirms favorable conditions”)
Perseverance can't detect these brines as it doesn't have Curiosity's Dynamic Albedo of Neutrons (DAN) instrument (NASA, 2012, DAN for Scientists), but calculations suggest they would also be found in Jezero crater; and last longer before drying out than they do in Gale crater (Chevrier et al., 2020, Global Temporal and Geographic Stability of Brines on Present-day Mars : Figure 7). Also as with Gale Crater, the ground temperature in Jezero crater often varies from well below -70 °C to well above 15 °C in a single day as measured by Perseverance (Atri et al., 2022, Diurnal variation of the surface temperature of Mars with the Emirates Mars Mission: A comparison with Curiosity and Perseverance rover measurements : Figure 3)
As with microenvironments in terrestrial deserts - most likely only some of the brines would be inhabited. Even though this salty water can be found almost every where there would be microbial oasis amongst them
- variation in composition of salts,
- variation in local conditions such as fissures in the rock, microcaves, and
- micropores in salt deposits
This would lead to more habitable patches of the brines. In Mars analogue deserts sometimes life is very patchy - it could be even more patchy on Mars with microbial oases kilometers or tens of kilometers apart or more - yet that could be enough for an occasional viable spore to reach Perseverance's sample, perhaps just a few spores in the entire collection.
In the oases where biofilms set up home on Mars, these would likely be a mix of many species working together for extra resilience like the grit crust in the Atacama desert (Jung et al, 2022, The grit crust: A poly-extremotolerant microbial community from the Atacama Desert as a model for astrobiology)
We covered this above in:
How to do a rapid 100% heat sterilized unmanned biological exploration of Mars using a simpler version of NASA's Venus HOTTech
This is just the second step of what's likely to be many steps to get an understanding of any martian biosphere or find out if it has present day life or had life in the past, see above:
It will be a huge plus for the next phase of Mars exploration if we can have 100% sterile rovers.
We can do that with some help from NASA's (NASA, n.d., HOTTech) technology, a program to build a Venus surface landers that can survive and function for at least 60 days at 500°C without any cooling.
"The goal is to develop technology areas that will enable a long-lived lander that can survive at least 60 days at 500C"
(NASA, n.d., HOTTech)
We can already build a complete surfacing surface probe on Mars that can not only be heated to 500°C without damage for months on end even function and do its job observing Mars (Kremic et al., 2021, Long-lived in-Situ solar system explorer (LLISSE) Potential Contributions to solar system Exploration).
Text on graphics: Venus HOTTECH program
Long-Lived In-Situ Solar System Explorer (LLISSE)The LSSE is designed to function for months at 500°C and relay data back to orbit.
A Mars HOTTECH program for 100% sterile landers and rovers only needs components to withstand 300°C for minutes.
Background image: (Kremic et al., 2021, Long-lived in-Situ solar system explorer (LLISSE) Potential Contributions to solar system Exploration : figure 5)
Here are some of the components in development, to work at 500°C without damage for anything from days to months on end
- electric motor and position sensor
- UV near field imaging (beyond the optical resolution limit of normal microscopes)
- radio transmitter
- solar arrays (to last at least a day at 500°C)
- batteries
- … for more see (NASA, n.d., HOTTech)
However 500°C is higher than we need to sterilize a rover 100%. We just need to be able to heat it to 300°C for a few minutes on the journey out to Mars to send 100% sterile rovers to Mars. See below:
That opens up many more possibilities that we can use right away.
- We have high temperature components commercially available for use for long periods above 200°C, developed for electric aircraft (where they save weight placed close to the engines), electric cars, and oil wells amongst other uses. This book is from 2012 and technology has advanced since then but it outlines the field (Watson et al., 2012. High-temperature electronics pose design and reliability challenges).
- Watson et al. also mentions the idea of testing components above their design temperature - especially since we just need them to resist a few minutes at 300°C we may find many components can do that already and just need to be tested to see if they comply already with our requirements.
- Fast electronics operating at 300°C with 0.35 micron resolution (Fraunhofer, n.d. High temperature electronics) (Fraunhofer, n.d. Integrated High Temperature Circuits - Fraunhofer IMS) using silicon in insulator technology (Fraunhofer, n.d. Silicon On Insulator (SOI))
Two technology pioneers for 100% sterile exploration: a proposal from 2017 to use the technology for a Venus surface rover to explore biologically sensitive habitats, and a proposed Europa cryobot from 2019
Video: NASA Engineers Design an Indestructible Venus Rover
The original idea was a wind powered almost fully mechanical rover on Mars which they called an "automaton rover" because it mainly used mechanical moving parts.
The suggestion here to use the same technology for planetary protection goes back to Venus HOTTECH researchers. They proposed (in 2017) that a sterilized automaton rover explorer deployed from a more capable but less fully sterilized master rover could be used to visit a nearby sensitive habitat (Sauder et al., 2017, Automation Rover for Extreme Environments : Section 6.2).
In the same year, 2017, Wilcox et al. designed a Europa probe that would be heated to 500°C on its journey out to Europa for complete sterilization (Wilcox et al., 2017. A deep subsurface ice probe for Europa)
These pioneering ideas for a 100% sterile probe have become much easier to implement as a result of advances in technology for HOTTech in just the last 5 years. We now have the capability to build a fully functioning probe that can survive for months on the Venus surface at 500°C with the components needed for a science mission ((Kremic et al., 2021, Long-lived in-Situ solar system explorer (LLISSE) Potential Contributions to solar system Exploration).
A 100% sterile Marscopter to explore sensitive local features like RSLs needs it to resist only brief heating at 300°C after deployment
I suggest we start with sterile Marscopters which would let our rovers send the marscopters to nearby features that they are not sufficiently sterilized to explore themselves and work up to a future where all missions to Mars are 100% sterile. This builds on a suggestion from the Venus lander team from 2018 which originally was almost fully mechanical. - with modern technology it becomes far easier already to have 100% sterile probes. See above:
With
- so much technology development in the last five years and with
- much lower specs, just to survive for a few minutes at 300°C
At 250 °C the half life of the RNA bases under hydrolysis is between 1 and 35 minutes, and
at 350 °C the half-lives are between 2 and 15 seconds (Levy et al., 1998. The stability of the RNA
bases: implications for the origin of life : 7935)
- Eight of the 20 amino acids have been proven to not just evaporate or liquefy but to decompose at temperatures between 185°C for Q (Glutamine) to 280°C for H (Histamine) (Weiss et al., 2018. Thermal decomposition of the amino acids glycine, cysteine, aspartic acid, asparagine, glutamic acid, glutamine, arginine and histidine)
- There would be other more recalcitrant organics remaining but a few minutes at 300°C would already be enough to eliminate viable terrestrial life and may be enough to eliminate the most problematic chemicals for giving “new ideas” to prebiotic chemistry.
Perhaps we could already build a Marscopter that could be sterilized to 300°C as it has relatively few components compared to Perseverance. Most of them seem to exist already in the NASA HOTTech program (NASA, n.d., HOTTech) with many of the other components such as video cameras already exist in commercial use, for instance to look inside ovens. These may not be able to survive for months at 500°C but many could easily resist a brief heating for a few minutes or an hour or so at 300°C.
How to sterilize: inside biobarrier or using onboard heater on the way to Mars?
A spacecraft using HOTTECH technology to achieve 100% sterilization doesn't need to be heated up to 300°C on Mars or just before re-entry to Mars. It could be heated here and enclosed in a bioshield opened before re-entry.
Viking used a fabric bioshield in two halves that separated to reveal the aeroshell. This image shows the orbiter (which didn't need a bioshield) and the lander in its shield. The bioshield had a vent that opened after launch to vent the atmosphere inside to prevent the bioshield bursting. The two halves separated using pyrotechnics to cut cables, when it was separated from the orbiter.
Viking image from (NASA, n.d. Viking 1 spacecraft) and overall summary of how it works (NASA, 1976, Viking Mission to Mars)
Details of how the lander is integrated into the bioshield (NASA, 1980, Viking '75 Spacecraft Design and Test Summary Volume II - Orbiter Design : 21-3)
The arm of the Phoenix lander was enclosed in a foil barrier. attached with latches which were released on Mars shortly after landing again pyroactivated.
Biobarrier for the Phoenix arm Bonitz et al., 2008, NASA Mars 2007 Phoenix Lander Robotic Arm and Icy Soil Acquisition Device : Figure 6)
Similarly a presterilized spacecraft can be enclosed in a bioshield, of fabric, or foil, ultra high vacuum (UHV) aluminium foil.
The alternative of an onboard heater is a proposal for Wilcox's 2017 design for a 100% sterile cryobot for Europa's ocean which heats itself up during the mission to Europa (Wilcox et al., 2017. A deep subsurface ice probe for Europa).
The advantage of the onboard heater is there is no biobarrier to release on the way to Mars or on Mars which eliminates a possible failure point. On the other hand without a biobarrier there is more risk of recontamination on Earth.
If sterility is the only aim a small amount of terrestrial contamination doesn't matter, because any contamination would be sterilized at 300 C. However the aim may be to remove all organics so as to avoid giving life on Mars new ideas with terrestrial biochemicals, or to avoid confusing life detection experiments with terrestrial organics. In that case it may be better to use a biobarrier since the sterilizing may be easier to do on Earth with that level of thoroughness.
There are three approaches to biobarriers which are listed in this early study from 2005 of possible ways to keep the spacecraft clean:
4.2.2.2 Biobarrier architectures
For MSR, a number of mission design options are under study. Key enclosure models include:
- Total spacecraft, in which the bioshield is released in orbit before the lander de-orbits.
This approach was used successfully by the Viking mission.- “Garage,” in which the rover is held in a protected region and surrounded by a biobarrier,
while the rest of lander is not as thoroughly protected.- “Arm,” in which a sampling tool and associated arm are protected in a biobarrier. The
lander or rover then delivers the arm to the sampling area, where it is unsheathed.((Barengoltz et al., 2005, Planetary Protection and Contamination Control Technologies for Future Space Science Missions : 27)
The bonus samples for the ESF lander would likely be enclosed in a biobarrier as for the "garage" approach. It is easy to sterilize a small thing like extra sample tubes / air compressor and enclose it. It adds a possible failure point on Mars but the risk of failure is low for such a simple form of containment. It is also only going to affect the bonus samples, and the entire point in them is to collect materials in clean containers.
The garage approach also may be appropriate for a sterile marscopter that can explore more sensitive regions deployed from a less sterilized rover, or perhaps it could use an onboard heater to self sterilize itself, or it could use the sterilized “arm” approach, with a sterilized grab or container it uses to pick up the pebble from an excavated crater and the bonus sample of clean salts.
With Perseverance, the engineers decided not to use biobarriers to protect the sample tubes or the sample collection apparatus.
The engineer’s perspective here is understandable. Based on the data they had, they made the best decision they could, given NASA’s constrained budget for robotic exploration, balancing cost with risk.
Engineers worried this risks jeopardizing the mission, if Perseverance got to Mars and then they found that the bag couldn’t be opened
The sample collection tubes, can be baked in a hot oven. As temperatures reach 500°Celsius (930°Fahrenheit), any organic materials are baked away and/or oxidized into carbon dioxide. As long as the materials can withstand those temperatures, it comes out of the oven completely clean. Contamination from air—specifically, air on Earth—is harder to avoid.
…
“Air is full or organic compounds—particles such as dust, volatiles in the form of gas,” Sessions said. “At the level of parts per billion, they’re everywhere.”
…
On the surface, the answer appears simple—put the sample collection tools inside an air-tight bag and transport it to Mars, keeping the material from ever coming in contact with Earth's atmosphere. But such a solution comes with its own problems.
"The engineers were very worried about this," Sessions said. "Imagine getting to Mars, and you can't get the bags open."
(Redd, 2015, How Much Contamination is Okay on Mars 2020 Rover?)
So, instead, after baking and sterilization, the sample tubes were exposed to the atmosphere in a clean room. They also had to be handled by technicians when they were placed in the rover. This decision made 100% sterilization impossible. So NASA went for less strict requirements.
However sadly this understandable engineering decision is what led to the mission being of almost no interest to astrobiology without bonus samples.
For a whole spacecraft the barrier idea is a major potential failure point. However with careful design, this method can work as Viking showed. In this case, for 100% sterility, we can use the same approach also for Viking. Clean the spacecraft as much as possible to eliminate as much as possible by way of organics. Then enclose the entire spacecraft in the biobarrier and heat the entire assembly to 300°C briefly, already enclosed in the biobarrier before launch. Then release the biobarrier once it is in space.
The biobarrier can be very low mass, for instance a foil barrier. It needs to be broken before the first course adjustments by the spacecraft to land on Mars, as the rocket motors need to be inside for the landing sequence.
Some things can't be heat sterilized to 300°C.
- propellant for the rocket engines for the landing sequence might not be able to withstand heating to 300°C. The rocket propellant would be burnt but there might be some unused propellant and the container for the propellant is another source for contamination with terrestrial life
- supply of labeled amino acids to feed to microbes for labeled release experiments which may be very sensitive for detecting life once the Viking results are understood and the anomalous chemistry allowed for (the temperature 300°C is intended to decompose amino acids
- other heat sensitive reagents and other consumables for experiments, and later, materials we might want to deliver to Mars for manufacturing processes on the surface,
- heat sensitive instruments that can't be heated to 300 C even briefly
That's not a problem in itself as there are many other ways to sterilize materials. So for instance the amino acids can be sterilized using ionizing radiation, or a mix of some heat combined with ionizing radiation.
The issue is how to integrate materials or instruments that have to be sterilized at low temperatures into the whole spacecraft if the spacecraft as a whole is going to be heat sterilized. Somehow the heat sensitive components need to be kept cool while the spacecraft is heated.
There are two main ways to do this, active cooling where heat is pumped out by various methods as for a fridge, or passive cooling. Since the spacecraft only needs to be heated to 300°C for a few minutes, or possibly a few hours to sterilize all life, passive cooling may be sufficient. It might be that all that's needed is heat insulation.
One approach would be to use vacuum to insulate sensitive components and materials, as for a thermos flask.
However we now have another alternative which is probably better than a vacuum chamber, heat resistant ceramic aerogels. They have very low thermal conductivity and are also ultra low mass. Silica aerogels are 1000 times less dense than glass (NASA, n.d. Aerogel).
Figure from (NASA, n.d. Aerogel)
The suggestion is to contain the amino acids in a heat resistant aerogel (which is also low mass) and sterilize the container and contents with ionizing radiation. The exterior of the container would be recontaminated but that is okay because the exterior is going to be heated up to 300°C. Then place the container inside the spacecraft still in the aerogel. Then when the spacecraft is heated briefly, the aerogel would keep its contents cold and undamaged.
So the suggestion is:
- put any easily heat damaged components or ingredients inside silica aerogel containers.
- Presterilize these with ionizing radiation and put hem in the whole spacecraft
- make sure the rest of the spacecraft is conductive enough to heat to heat up enough to be sterilized
Then we use the two possibilities already mentioned to sterilize the entire spacecraft
- wrap it in foil or fabric bioshield as for Viking and heat for a few minutes or hours at 300 C on Earth
- or else use an internal heater as for the Europa cryobot but only for a few minutes or hours at 300 C
Anything in the aerogel containers will be unaffected by the heating because the heat doesn't reach far enough into the aerogel to harm it in that time and the aerogel will only absorb a small amount of heat.
Chris McKay's view that human missions to Mars can be biologically reversible and any terrestrial contamination should be reversed if we find a second genesis on Mars
Chris McKay is of the view that any contamination after a human mission can still be biologically reversed. He says our goal is to enhance the richness and biodiversity of life in the universe. He says firstly, we need to search for and support other life in the universe, and secondly, to expand life from Earth.
Video: Biologically Reversible Exploration
Applying these principles to Mars, Chris McKay writes that we need to explore Mars in a biologically reversible way, and gives as his expert opinion that we can reverse the effects of a human landing if needed :
The discovery of a second genesis of life besides the one on Earth, this time on Mars, would have profound scientific and philosophical implications.
To protect a second genesis as we search for it, the robotic and human exploration of Mars should be done in a way that is biologically reversible, i.e., we must be able to undo our contamination of Mars if we discover a second genesis of life there. It is important to note that human exploration can be done in a way that is biologically reversible.
(McKay, 2018,. The Search on Mars for a Second Genesis of Life in the Solar System and the Need for Biologically Reversible Exploration : abstract)
It is important to note that human exploration can be done in a way that is biologically reversible.
(McKay, 2018,. The Search on Mars for a Second Genesis of Life in the Solar System and the Need for Biologically Reversible Exploration : abstract)
Then in the main body of the paper he explains that we need strict control of introduction of Earth life to Mars and ensure that if we find a second genesis on Mars, that all Earth life can be removed from Mars:
While control of contamination is desired even if there is no life or Earth-like life on Mars, the potential implications of contamination are more profound if there is or was a second genesis in the solar system on Mars, besides the one on Earth. In this latter case, in particular, strict control of the introduction of Earth life to Mars is indicated
The complete and effective sterilization of every spacecraft going to Mars is probably not an achievable option and would be pointless anyway since several spacecraft have already been sent to Mars with known bioloads. Instead, an adequate and achievable approach to controlling biological contamination on Mars is to require it to be reversible (McKay 2007, 2009b). This would imply, for example, that if at some time in the future a second genesis of life were discovered on Mars, then all Earth contamination could be removed.
(McKay, 2018,. The Search on Mars for a Second Genesis of Life in the Solar System and the Need for Biologically Reversible Exploration : 105)
He sees great value in a second genesis if that is what we find on Mars and wants to restore Mars to a previously more habitable state, writing
The discovery of a second genesis of life in our solar system has profound scientific as well as philosophical and ethical importance. Philosophically, the discovery would directly address the question of life in the universe, and would suggest that the phenomenon of life is distributed throughout the universe. Scientifically, having another example of life expands the scope of biology from one to two. There may well be significant advances in medicine, agriculture, pest control, and so on, from having a second type of life to study. If that second life is allowed to form a second biosphere, the practical benefits of the study of a second example would extend to the human management of Earth’s global biosphere as well.
The concept of assigning intrinsic value to life- forms is perhaps most clearly stated in the first two tenets of deep ecology (Naess 1984; Devall and Sessions 1985):
- The well-being of nonhuman life on Earth has value in itself. This value is independent of any instrumental usefulness for limited human purposes.
- Richness and diversity in life forms contribute to this value and are of further value in themselves
When applied to Mars (McKay 1990) these principles argue that a biologically rich Mars is of more value than the fascinating but largely dead world we see today. Furthermore, a Martian biosphere populated by a second type of life would contribute to more diversity than one populated by trans- plants from Earth. We know from observations of Mars that it does not have a rich global biosphere. Thus if there is Martian life it is either dormant or surviving within some limited refugia.
(McKay, 2018,. The Search on Mars for a Second Genesis of Life in the Solar System and the Need for Biologically Reversible Exploration : 105)
He say that though it is possible that alien life and terrestrial life might be able to co-exist, we would expect one to dominate in the competition for biological resources and drive the other to extinction.
We do not know what would be the fate of that life if Earth organisms were either to colonize these existing refugia or were to be present as Mars was warmed in the first step of terraforming. It might be that cohabitation of Earth life and an alien type of Martian life is possible. However, the facts of biological competition for resources would seem to indicate that one form would dominate and drive the other to extinction. Thus, it would seem that the notion of the intrinsic worth of life and diversity of life would dictate that we not allow contact between Martian life and Earth life until the implications of such contact are fully understood
(McKay, 2018,. The Search on Mars for a Second Genesis of Life in the Solar System and the Need for Biologically Reversible Exploration : 108)
The scenario of terrestrial life dominating martian life is the main concern in the forwards direction but for backwards contamination, domination at the microscopic level could go in either direction. One way martian life could win this competition is if it has an intrinsic advantage.
We look at this for our likely low probability but example worst case scenario of mirror life. If Mars has mirror life, it may be likely to be adapted to be able to use both symmetries of organics because most organics on Mars, even if there is indigenous life there, likely comes from infall from space. If so, it would be able to convert terrestrial organics to mirror life. With this advantage, it would win the competition for resources in microbiomes on Mars every time until terrestrial microbes develop the necessary isomerases to flip the symmetry of enough martian organics to metabolize them. See below:
- Mirror life as a potential astrobiological surprise like the unexpected carbon dioxide geysers for geology
- Scenario of a mirror life algae from Mars pre-adapted to normal organics brought to Mars by infall from space
- Great Oxygenation Event used to show a single species could in principle cause large scale biosphere changes and mass extinction as for the worst case mirror life scenarios
He also is of the view that it is important to preserve even lifeless landscapes on Mars and the Moon, places where life is absent or cannot grow (McKay, 2019,. Preservation of Static Lifeless Landscapes in the Antarctic Dry Valleys and the Atacama Desert and Applications to the Moon and Mars).
More on the value of such deserts here:
In his views on the need for biological reversibility Chris McKay goes beyond the views of those who think we should resign ourselves to an inevitable anthropocene on Mars. He is of a clear view that we should not permit the start of an anthropocene if that is possible. However his expert opinion is that our exploration can continue to be biologically reversible even after a human landing.
It is important to note that human exploration can be done in a way that is biologically reversible.
(McKay, 2018,. The Search on Mars for a Second Genesis of Life in the Solar System and the Need for Biologically Reversible Exploration : abstract)
This is a minority expert opinion in the literature which has had little attention. There are only 10 cites of his paper in Google scholar. This is a significant view that doesn't seem to have been discussed in the papers by other participants in the debate about the potential for an irreversible anthropocene.
As we saw above,NASA's first two planetary protection officers have as their expert opinion that human missions introduce terrestrial life to Mars irreversibly, though it can likely be contained to the vicinity of the areas of human exploration through careful procedures at least in the near term. Some other astrobiologists who have published on the topic are give as their expert opinion that it's likely that after the high bioload of a human landing, introduced life would start the martian anthropocene and irreversibly contaminate Mars globally.
For Chris McKay's ideas to work of biologically reversible exploration after a human landing on Mars, we need the knowledge gaps identified in the SSB review to be filled in the direction of finding that there are no microhabitats for life on the surface or connected to the surface that could be contaminated irreversibly, or that they are so isolated that life can't spread far enough to be irreversibly introduced to Mars. See above:
We need to consider how to reverse forward contamination after crashes of human occupied spacecraft on Mars.
Also any machines used to biologically reverse terrestrial contamination will need to be 100% clean themselves. See:
Whatever the situation after a human landing, restoration ecology to restore Mars to a more habitable state does need the contamination we already introduced to Mars to be biologically reversible. There seems general agreement that we probably haven't contaminated Mars irreversibly yet, though as we saw this is on the basis of probability, because of our use of only partially sterilized rovers. The aim of the current planetary protection guidelines, if one traces them back, is to achieve less than 1 chance in 1000 of irreversible forward contamination per mission. They most likely do better than that because of the very cold dry, UV radiated martian landscape.
So it seems plausible the terrestrial contamination we introduced so far could be reversed. If so, how might we do it if it is necessary? We will look at this in more detail in the next section:
How can we resolve this with more data from Mars? Once we have 100% sterilize landers and rovers, the Phoenix lander site seems an ideal location to visit since the lander got crushed by the advancing dry ice sheet from the northern polar ice cap as winter approached (as expected). It is also in an uncertain region with some potential for microhabitats for terrestrial life, and so seems a perfect spot to test how effective our planetary protection has been so far, and how easily it can be biologically reversed (hopefully).
As one of the most challenging of all the sites we'd need to decontaminate if we need to reverse introduction of life to Mars, if we can do biological reversal from the Phoenix lander site there is a good chance we can do it for the other robotic missions we sent so far. However Chris McKay goes further and outlines a way to reverse the contamination from a human landing on Mars. So let's look at that.
Is terrestrial contamination of Mars biologically reversible for human missions to sites like Jezero crater?
In the last section, we looked at Chris McKay's idea of biologically reversible exploration of Mars, Chris McKay goes on to describe in detail how to reverse the terrestrial contamination already there. All the spacecraft and pieces of metal would need to be removed. Then the surface dust needs to be blown up in to the atmosphere and kept suspended for several hours of Martian daylight until it has been exposed to UV for long enough for all microbes present to be sterilized. For the Mars polar lander it would be a case of digging up the crashed spacecraft from the crater it formed as it impacted into Mars and removing those pieces. Chris McKay is of the view that this process would reverse all the current terrestrial contamination and restore a planet free of terrestrial life.
He is of the view that the only form of contamination that couldn't be biologically reversed is deep drilling, if it reaches subsurface aquifers and these are globally connected. So any deep drilling should be with fully sterilized equipment until we know what is there.
If we do find a second genesis of life on Mars, Chris McKay is of the view that we should still terraform Mars to make it more habitable, but remove all terrestrial life first, which is why he requires all exploration to be biologically reversible. He calls this ecopoesis, ore restoration ecology, restoring conditions that life had on Mars early on.
The Martian life would be studied in detail to determine the environmental conditions that support its growth. The assumption would be that Martian life would thrive best in warm and wet conditions because the early history of Mars was warm and wet, and thus any life that originated on early Mars is likely to be similar to Earth life in requiring liquid water conditions.
Once all Earth life were removed, “terraforming” would proceed to allow that life to spread globally. Any indigenous life on Mars is likely to be microbial, and while it may spread globally on a Mars that has been altered to be warm and wet, it will not create the diverse and powerful biosphere that could be created with Earth life (especially trees). This is as it should be because the goal of terraforming in the case of indigenous life should not be focused on creating an environment suitable for humans but on enhancing the richness and diversity of life in the universe (McKay 2013; Randolph and McKay 2014).
(McKay, 2018,. The Search on Mars for a Second Genesis of Life in the Solar System and the Need for Biologically Reversible Exploration : 108)
He thinks that even after terraforming to make Mars more habitable for its indigenous martian microbes, if any, that Mars and Earth can be kept biologically isolated through to the indefinite future by deflecting any material that gets to Earth after larger impacts on Mars.
As discussed above, there are good reasons for supposing that impacts and meteorites could result in the natural exchange of biological material between Earth and Mars. Therefore, it may be argued that even if Mars has indigenous life, if that planet is made habitable and life flourishes there then cross contamination between the planets is inevitable, as Mars life is carried to Earth and vice versa. This would be true if human activities with respect to large impacts are not considered. It is almost a certainty that humans will develop the technology to shield planets from impacts: Spaceguard, the idea that NASA and other space agencies will set up systems for tracking and intercepting objects on a collision course with Earth.
This technology will not be deployed specifically to prevent biological cross contamination of the planets, but to protect human life on Earth and in settlements on Mars. Thus future biological isolation between the two planets can be maintained.
(McKay, 2018,. The Search on Mars for a Second Genesis of Life in the Solar System and the Need for Biologically Reversible Exploration : 108)
He presents a similar view still in 2019
If the adequate amounts of carbon dioxide and nitrogen are not present on Mars then terraforming is relegated to the distant future when human space technology is many orders of magnitude more advanced. If a terraformed Mars thus become impossible for all intents and purposes then this may call in question the very motivation for human exploration and plans for long term settlement. It also couples to the ethical question of human speciation on a Mars that cannot be terraformed to suit human life.
Finally, the question of terraforming Mars connects to environmental ethics and to the search for a second genesis of life on Mars. As has been suggested22 the ethical path in the event of such a discovery on Mars is continue with terraforming but with a view to enhancing the richness and diversity of that indigenous Martian life all the while maintaining strict separation between Earth’s biosphere and the emerging Martian biosphere.
(McKay, 2019,. Prerequisites to Human Activity on Mars: Scientific and Ethical Aspects : 321)
He also is of the view that it is important to preserve even lifeless landscapes on Mars and the Moon, places where life is absent or cannot grow (McKay, 2019. Preservation of Static Lifeless Landscapes in the Antarctic Dry Valleys and the Atacama Desert and Applications to the Moon and Mars).
More on the value of such deserts here:
In his views on the need for biological reversibility Chris McKay goes beyond the views of those who think we should resign ourselves to an inevitable anthropocene on Mars. He is of a clear view that we should not permit the start of an anthropocene if that is possible. However he is of the view that our exploration can continue to be biologically reversible even after a human landing.
Chris McKay introduced the new idea of biologically reversible exploration in 2009, and suggested that COSPAR should set a policy that all exploration, human or robotic, is biologically reversible:
We should not do anything now that would close off options for the future. I propose that COSPAR, in its upcoming discussions, set a policy that all Mars exploration be biologically reversible and that this policy extend to human exploration as well.
(McKay, 2009. Biologically reversible exploration)
COSPAR never made that change in policy. However with the technology of the day it could only be based on probabilities. It would be a major challenge to reverse terrestrial contamination after a human landing, and based on our knowledge so far there is a small but not zero chance that any mission we send to Mars might introduce terrestrial life irreversibly. We could have introduced some life to Mars irreversibly already though most expert opinion would say this hasn’t happened yet. We certainly sent viable spores to Mars but most was sterilized by the harsh conditions there and what remains is confined to our rovers, landers and the spacecraft that crashed on Mars, and the tracks they drove over on Mars and close to them.
It is too late to explore Mars in a way that guarantees biological reversibility, but we do have that capability for other places in the solar system that we haven’t sent rovers or landers to yet. With Mars, we likely haven’t introduced terrestrial life irreversibly yet and we have a choice as a civilization to explore it from now on in a way that prevents any future forward contamination.
If we have introduced life to Mars irreversibly already, it is likely to be limited to very few species. For instance, suppose we have accidentally introduced a single hardy species of microbe able to live in high concentrations of salt solutions on Mars. This may be able to co-exist with other native indigenous life without making it extinct or harming it in any way. It might even take part in a native biofilm in a way that is beneficial to the rest of the microbial community of native martian life. It wouldn’t follow that all terrestrial life can co-exist with martian life in a similarly harmless way and we would still have a motivation to keep all future exploration biologically reversible.
Chris McKay says that if we do find life on Mars after a human landing we should remove every spacecraft, every piece of metal from Mars and should sterilize the landing site for the human visitors and all the landing sites of all the spacecraft and then terraform Mars, but terraform it for the native martian indigenous life not humans.
For Chris McKay's ideas to work we need the knowledge gaps identified in the SSB review to be filled in the direction of finding that there are no microhabitats for life on the surface or connected to the surface that could be contaminated irreversibly, or that they are so isolated that life can't spread far enough to be irreversibly introduced to Mars. See above:
A few remarks to give another respective on his ideas of biological reversibility. This idea of removing all terrestrial contamination from Mars seems easier to achieve with the partially sterilized rovers and landers we already sent to Mars. Any microbes would likely be solitary spores or dormant states.
It would be far harder after a human landing. As an example, a ten day mission to Mars by 6 people, might contaminate the surface with sixty billion microbes by analogy with Antarctic microbial research camps in the McMurdo dry valleys. The researchers stay within a fixed area around the camp in order to limit their impact, in a "corral" system. They give as a a typical example, a corral that's 50 meters square. After a ten day camp with 6 people restricted to those 50 square meters, they will leave an estimated sixty billion microbes in the soil.
Assuming those sixty billion microbes are evenly mixed into the top one cm of the site, then that makes it around a hundred thousand microbes in each cubic centimeter, which is between 0.1% and 10% of the natural population of microbes in those sites. Calculations from (Cowan et al., 2011. Non-indigenous microorganisms in the Antarctic: Assessing the risks : box 2).
This calculation is based on one person shedding a billion microbes a day. With a human mission to Mars, many of those would remain in the habitats or in the spacesuits or any rovers, and astronauts could implement a far tighter corral system than in Antarctica for that reason. However, some would get onto the surface when airlocks are opened, or on the surface of the spacesuits, or on instruments they place on the surface, or on the exterior of their spaceship, and in other ways.
If we need to reverse this contamination later on, the biofilm fragments from a human landing would be harder to sterilize than the individual microbes from partially sterilized robotic rovers and landers. It would also be harder to sterilize the skin flakes, loose hairs, etc., that likely get out of the habitats to the surface on occasion when air locks are opened. Then the larger bioloads from a human landing would lead to a higher chance of microbes that end up in microcaves, or micropores or rock fractures and possibly even subsurface caves with entrances connected to the surface.
The suggestion to levitate the debris above the surface could lead to suspending fragments of biofilms which might then travel far from the landing site in the surface winds and still be viable depending on how viable biofilms are in the atmosphere of Mars. We looked at Billi et al's experiment in:
It would be especially hard to achieve complete biological reversal if Mars astronauts crash on the surface with a debris field as for the space shuttle Columbia crash. The landings on Mars are likely to be exceptionally challenging because of the thin and variable atmosphere, and if there are many human missions there we are likely to have a crash at some point. I cover this below:
Also any machines used to biologically reverse terrestrial contamination will need to be 100% clean themselves or they may add to the problem at the same time as solving it. The proposal here to develop 100% sterile landers and rovers and send them to Mars would help with the process of biological reversibility of existing viable terrestrial life on Mars. See:
If we are able to transition fully to 100% sterile landers and rovers, it would also mean we no longer introduce any more terrestrial life in the future from our robotic missions. We then only need to reverse the landings and crashes on Mars so far.
Even with our robotic landers, it might be a challenge to achieve reasonable confidence of 100% reversal of the effects.
Perhaps harder to remove life could be removed using other methods, for instance we could send a high intensity gamma ray source such as cobalt 60 in the future that would slowly retrace all the tracks of our current rovers to sterilize the surface of terrestrial life. This would be a priority if we work to make Mars more habitable for martian indigenous life, especially a second genesis. The 100% sterile rovers would also need to sterilize any fractures, microcaves, and search for hidden entrances to caves they might have missed.
However if we have reached a point where we need to decide between terraforming for humans or a program of restoration ecology to make Mars more habitable for martian life after discovering a second genesis there, we can assume a very high level of technology available. With that level of technology, it may not be so hard to erase all traces of terrestrial life as it may seem today with only present day capabilities.
Whatever way the knowledge gaps are resolved outlined in the SSB Review, there seems a good chance that our exploration so far is biologically reversible. Once we have 100% sterile landers then we can make a start on removing some of the contamination from Mars and exploring the previous landing sites to see if there is any forward contamination and to what extent it's present.
It would be far more challenging to do biological reversal after a human landing. If the surface of Mars is truly as inhospitable as Chris McKay sees it, as his expert opinion, that too may be possible. But if humans are exploring from orbit anyway first, we won’t land until we now whether or not there is a second genesis on Mars, and whether we need to explore in a biologically reversible way. We can then make decisions based on knowledge of what we have on Mars.
The Phoenix lander site as a top priority for testing the potential for biological reversibility of terrestrial life introduced to Mars and effectiveness of planetary protection to prevent forward contamination
Ideas about biological reversibility don't need to be based on theory. Once we have 100% sterile landers we can send them to the uncertain regions that may be special regions where our landers are more likely to encounter potential habitats for terrestrial life.
The Phoenix lander site would be a top priority. Where it landed is now classified as an uncertain region that may turn out to be a special region, likely to have microhabitats for life, as a result of the lander's own discovery of droplets on its legs. For the planetary protection classification system for Mars see above:
Also the lander was crushed by dry ice sheets with the advancing winter as expected.
Phoenix lander crushed by frost - layers of dry ice forming on the solar panels in winter snapped one of them off. It was not expected to last the winter. The right hand image shows it in 2010, two years after the image on the left which shows it soon after landing, in 2008. This shows a shadow from the western solar panel but no shadow from the eastern solar panel. It been snapped off.
(NASA, 2010. Phoenix Mars Lander Does Not Phone Home, New Image Shows Damage)
So it is a damaged crushed lander. It also spends each winter beneath a combination of dry ice and water ice, which might lead to microhabitats such as subglacial melt or cryoconite holes. For these suggested microhabitats on Mars see below:
- Potential for distant microbial oases as sources of ultra low concentrations of viable spores in dust storms
- Potential for distant Mars habitat common in Antarctica: Fresh water drilled by sun warmed dust and prevented from evaporation by an ice lid
If Phoenix has started to contaminate Mars we might find a small enclave of life around the lander. This would help to test to see how effective our planetary protection measures are. Phoenix is also a good candidate to study because we know exactly where it is, and have a lot of ground data from it from before it got crushed by the advancing dry ice.
A mission like that could be dual purpose, first to search for life habitats, past and present life signs and so on , in what is now thought of as a "uncertain region" that could have a high potential for present day martian life. So it would land some distance away from Phoenix, equipped with in situ life detection instruments. Then it would travel up to the crashed lander, photograph it, and analyse the remains and test for liquid water droplets and for signs of life. It could also examine the spacecraft itself for viable life there to test how sterilizing the Mars conditions actually are and whether they do correspond to the dry sterilization stage of Viking.
A similar mission could study the Mars polar lander crash site to find out what remains of it and whether it created a temporary habitat by melting the ice when it crashed into the ground. It would give valuable ground truth on how effective planetary protection is for landers that crashed. In both cases, if life is found that's actually growing the same mission or a follow up mission could then work to sterilize it to prevent the forwards contamination from spreading further as far as is practical. There the very cold dry martian conditions would help, as if there are only small levels of terrestrial life it likely takes a long time for it to grow slowly and then spread to a new microhabitat on Mars.
We can then use studies of how far it has spread combined with modelling of martian surface conditions to work out how challenging or easy it is to reverse the biological contamination of Mars which has certainly happened with the Phoenix mission, even if it is just a few hardy still viable spores attached to the rover itself.
If we can reverse terrestrial contamination, the next step if we wish to do restoration ecology is warming Mars up. We cover this in the Supplementary information under the discussion of the Swansong Gaia after first looking at how Mars might have got into its current barely habitable state. This affects how we can do the restoration ecology so the restoration ecology section is left to the end. See: Supplementary information under:
- Could there be alternating episodes of runaway warming and runaway atmospheric collapse in early Mars - and could we trigger a runaway warming today?
- Potential for a stronger biological pump during runaway warming if the early Mars atmosphere frequently collapsed and then recovered in a runaway warming again.
-
Estimated 100 millibars of dry ice absorbed in the regolith - is this involved in any feedback?
-
Feasibility of terraforming Mars or ecopoesis (making Mars more habitable for native martian life)
Humans in orbit around Mars exploring with immersive VR using 100% sterile surface avatars and what they could do
This future was explored in a 2012 Exploration Telerobotics Symposium organized by NASA (NASA, 2012. Space Exploration via Telepresence: A New Paradigm for Human-Robotic Cooperation )
Using NASA’s remarkable Venus HOTTECH technology, all these surface assets can be built to withstand months at 500°C, and so are easily sterilized with a few minutes of heating on the journey out.
Main image: “Safely tucked inside orbiting habitat, space explorers use telepresence to operate machinery on Mars, even lobbing a sample of the Red Planet to the outpost for detailed study." (Valinia et al. 2012, Telerobotics Could Help Humanity Explore Space)
As attendees at the 2012 symposium put it in a review paper after the symposium
Although landing humans on the Martian surface may remain our ultimate goal [2], extending human cognition/dexterity to local surface sites with high science potential in this way can be achieved on a shorter timescale and at a far more modest cost, and may ultimately serve the needs for humans on these surfaces.
…
It is entirely feasible that surface exploration of so-called Special Regions (on Mars) will require higher fidelity and more adaptable science activities that all but precludes human access at any time, and hence low-latency telerobotics may be a formal requirement to achieve science goals in such important locales. At such sites, LLT methods could include human orbiting or human operators on the surface at a safe standoff distance to allow appropriately prepared telerobots for access and exploration.
(Valinia et al., 2012. Low-latency telerobotics from Mars orbit: the case for synergy between science and human exploration)
Space.om highlighted some of the take home messages from the Symposium, that humans and robots work together and the budgets for robotics and for human space exploration can support each other:
Colleen Hartman, director for science, operations and programs at NASA's Goddard Space Flight Center
"Let's stop pitting science against exploration. The battle between humans and robots is a false one and a waste of time."
Robert Cabana, director of NASA's Kennedy Space Center
"The human-robotic interface is going to be key to our success now and in our future. you've seen the robotic devices we use to support astronauts in space. It's absolutely phenomenal."
Stephen Altemus, director of engineering at NASA's Johnson Space Center (summary of what he said by Space.com)
Even as robots take over routine and risky jobs (such as spacewalks), humans could potentially do much more meaningful science with their robot buddies. Future missions may have astronauts controlling robots on planetary surfaces from orbit, riding on robotic rovers, and even wearing robotic gloves or full-body exoskeletons to boost their natural strength and mobility.
(Hsu, 2012, NASA Calls for Ceasefire in Human-Robot Space Budget Wars)
Here is Nic Radford, Robonaut's Deputy Project Manager talking about how robonaut helps to substitute for routine activities that take up much of the time of ISS crew members, freeing them for the science activities only they can do.
Video: Nic Radford Interview
The ISS already uses robonauts for this purpose doing routine tasks that take up time of the crew members.
Text on graphics: First handshake in the ISS of Robnaut with astronaut, humans and robots working together in space.
Video description: Robonaut extends its hand for the first "man-machine" handshake in space with Commander Dan Burbank.
Video: Robonaut shakes hands
For more information and videos: (NASA, n.d., Robonaut)
Since many of our tools are designed for humans to use, we can deploy assets to the surface that are dual purpose, that can be operated by astronauts or robonauts, and design some of the surface avatars based on human shapes.
For instance we could send robonauts to the surface in place of astronauts, and then later if the surface proves to be biologically safe for humans, they can use the same tools and equipment the robonauts used, as for the ISS. Then if we find it is never biologically safe for humans to land on Mars, the robonaut avatars on the surface are easy for humans in orbit to operate as they are the same shape as the human operators.
Meanwhile, we can speed the Mars exploration so much that using the idea of "artificial real time" from computer games, we can control our rovers almost as easily as a rover on the Moon (say), which NASA will be able to do once you have broadband optical laser communication with Mars later this decade.
It works by simulating the position of your rover as it will be when the commands get to Mars, so you can drive in a virtual landscape in real time with no latency, a technique used in online multiple player games. In this video Dr Jeff Norris talks about using way points - so you tell the rover where you want it to go, and so long as the waypoints are ahead of where it has got to it doesn’t slow down.
Video: Telexploration: How video game technologies can take NASA to the next level
This was later developed into OnSight using Microsoft’s “hololens” software.
Video: Walking on Mars with HoloLens [OnSight]
For more about using Hololens to explore the Mars surface virtually (Norris, 2016, Immersed on Mars — Dr. Jeff Norris)
It will help also to have more autonomous and more rugged rovers. This is not about speeding at 30 miles per hour. At present 100 meters a day is fast. As of 2023, the off-world speed record of any rover is 18 km / hour for the Apollo 17 lunar rover (Guinness World Records, n.d. Lunar Speed Record). This video shows the astronauts "speeding" with the Apollo 16 lunar rover.
Video: Apollo 16 Lunar Rover "Grand Prix"
Traveling even a tenth of that speed would be revolutionary. The fastest unmanned rovers would be the Lunakhods. Lunakhod 2 - traveled about 39 km on the Moon in about 4 months driven from Earth by the Russians, using the updated distance measurements (LROC, n.d., Trundling Across the Moon). We may be able to improve on that with modern technology and software even as far away as Mars.
We already have gigapixel images of the surface of Mars built up from large numbers of individual photographs by the Curiosity mast cam. (Bodrov, 2021. First 4.5-billion-pixel of Mars by NASA's Perseverance Rover)
By the 2030s with fast bandwidth we can have gigapixel video cameras on Mars. We'll have scenes at far higher resolution than this, streaming in real time from Mars eventually, building up a 3D virtual world in microscopic detail as the rovers traverse Mars.
With gigapixel video cameras on the rovers, streaming back broadband to Earth, scientists and enthusiasts will be able to look at the landscape on Mars with a virtual hand lens, examining any rock the rovers ever passed by in microscopic detail. Their discoveries could then lead to the rover turning around and looking at the rock again - but as rovers get faster, able to move tens of kilometers a day as for the lunar rovers or even faster - and not limited like the lunar rovers were, to remain within walking distance of a base (Williams, 2016. The Apollo Lunar Roving Vehicle). We need far faster and more robust rovers for human exploration if we do get humans on the surface. We can send them there first, for robotic exploration.
Several authors have suggested bootstrapping space exploration with seed factories that makes more equipment on the surface of the Moon or other destinations, and even a copy of itself (Freitas et al., 1981, A self-replicating, growing lunar factory) (Kalil, 2014, Bootstrapping a Solar System Civilization) (Metzger et al., 2013. Affordable, rapid bootstrapping of the space industry and solar system civilization)
We could start with 3D printers that can large parts of rovers, marscopters, etc., which are assembled in situ to minimize the mass we need to send to Mars.
This would also help with keeping the equipment on Mars sterile of terrestrial life, if this is needed. So long as the original seed factory or 3D printer is sterile, any equipment made on Mars made by the small seed factory of 100% sterile manufacturing robots and any other 3D printers it makes would itself also be sterile. This could be a way to accelerate exploration or commercial use of Mars while keeping Mars 100% free of terrestrial life and this then is scaleable to large scale facilities and factories on Mars made largely with components constructed from raw materials sourced in situ.
In this way we would have many assets already in place when the astronauts get there, and data links working and tested, and capable teams on Earth to do most of the heavy work leaving it for the astronauts in orbit to be used to their maximum for the executive capabilities. For them it might be not unlike playing a game of “civilization” stepping in from time to time to help one of the robots that is stuck on some task or needs to be operated directly for some time sensitive experiment.
So we can do a huge amount of exploration on Mars from Earth in the very near future, and all this with those near future 100% sterile rovers that can explore anywhere with no planetary protection restrictions. All of this builds up assets on Mars and knowledge about Mars that will be very useful when humans get there, whether in orbit or on the surface.
The Mars colonization enthusiasts are so sure that the Martian biosphere will be safe for Earth. If they are right we will get a “pass” as a result of this exploration. It will hardly interrupt their plans at all if their confidence is well placed. Not only that they would get much broader backing because their confidence will be based on scientific knowledge about Mars rather than optimistic hunches and vivid metaphors.
While if they have to stay in orbit, and colonize the moons of Mars, they can use these assets to exploit Mars with robotic exports to their colony, even grow plants on the surface.
We can sterilize seeds without any risk of contaminating Mars with Earth micro-organisms.
Text on graphics: We could grow plants on Mars even if it has mirror life that can never be brought back or Earth has microbes can never be sent to Mars.
Seeds can be sterilized and grown in sterile aquaponics
(NASA, 2016. The Real Martian Technologies: Our Little Green Friends)
In this way we could have greenhouses on the surface of Mars and these could grow food for the colonists in orbit, high value items especially like medicines, or if Mars has valuable but hazardous native life it may be easier to grow it on the surface of Mars via telepresence.
We can in principle grow many terrestrial crops on the surface of Mars with no risk of contamination in either direction, on most scenarios. We could export those crops to the orbiting colonies, for instance on Phobos or further afield.
For details see:
. We can also do mining for minerals on Mars, and prospect for assets that may be worth selling to Earth. With automation and supervision from orbit, we can do everything the colonization enthusiasts want to do except humans on the surface.
A civilization’s choice: protecting Mars and Earth 100% now keeps all future options open until we fill knowledge gaps
I conclude we have technology already to achieve 100% protection of Earth, and we have technology in development which could be accelerated to protect the rest of the solar system from terrestrial contamination, enabling 100% planetary protection in both directions, and we can do this with no loss of science return. Indeed by opening up the most sensitive areas of Mars, Europa’s ocean and so on to 100% sterile robotic explorers, this new technology has potential lead to a huge advances in science.
It is our choice as a civilization whether we do this. We can work together to find a way forward to a stimulating future. We don’t know which of these scenarios we face, a future where it is:
- ALEADY SAFE for humans to explore the surface of Mars in person with no precautions needed in either direction
(such as no life or mutually benign life on Mars)
- CAN BE MADE SAFE WITH LIMITED QUARANTINE and other measures for travel between the planets to keep out certain invasive species that would harm Earth’s biosphere, humans, or agriculture.
- NEVER SAFE for humans to explore Mars in person - or at least, never safe for Earth’s biosphere to return to Earth after doing so
(such as life based on mirror organics on Mars)
The reason we need planetary protection is because we don't know for a certainty which of those scenarios we will face on Mars.
So we need an open mind here, to look at this with clear eyes and develop a Mars exploration program that is inspiring and stimulating for both robotic and human missions no matter which scenario we face, without risk to Earth's biosphere, or the future science value of Mars and without the risk of multi-billion dollar stranded assets on Mars.
If we drop planetary protection and send humans to the Martian surface, many astrobiologists are in agreement that this is likely to set in morton the anthropocene on Mars which can't be reversed. It also initializes the anthropocene on Mars with an accidentally selected particular initial mix of microbes, consisting of the first accidental microbial colonists from early human colonization attempts.
[TO EXPAND WITH CITES]
A more resilient way to achieve NASA's end goal of humans throughout the solar system with an intermediate goal to explore Mars from orbit, with In Situ Resource Utilization (ISRU) on its moons
NASA's current plan is goal based of sustained human presence throughout the solar system.
Text on graphic: NASA's rightmost goal can also be achieved with humans in orbit around Mars exploring a mirror life planet with telepresence avatars and similarly orbiting Venus, landing on Mercury, Callisto, perhaps Titan and beyond and on the Moon, asteroids and Near Earth Objects
Background image on the slide: (NASA, 2007, Titan beyond the rings)
Slide from here in the video: (NASA, 2022, NASA seeks input on Moon to Mars Objectives: 13:09)
Titan, shown as the background image in Jim Fries' presentation is a possible objective for human exploration as we'll see. Conditions there are so very cold that even if it does have native life we are likely to find that Titan life can't survive on Earth and terrestrial life can't survive on Titan though this would need to be verified before landing humans there.
This doesn't require humans on Mars. However they have an intermediate goal to land humans on Mars. This is not necessary for their final goal and is inflexible.

Text on graphic:
Mirror life Mars?
Mars, unlike the Moon, could have life based on mirror organics or exotic biology.This would greatly increase human interest in space exploration
However, in some scenarios it may never be safe for human astronauts to land there.
Background graphic from: (NASA, 2020, NASA Updates Planetary Protection Policies for Robotic and Human Missions to Earth’s Moon and Future Human Missions to Mars)
Slide from here in the video: (NASA, 2022, NASA seeks input on Moon to Mars Objectives : 11:29)
On the basis of the issues identified in this book we can't assume a biologically safe Mars at our current level of understanding of the planet.
Text on graphic:
RECOMMENDATIONS
This plan must be able to accommodate a mirror life Mars
Can't assume a biologically safe MarsNeeds rapid exhaustive biological exploration before human landing decision.
Slide from here in the video: (NASA, 2022, NASA seeks input on Moon to Mars Objectives : : 12:26)
Background graphic on the left from (NASA, 2021, HEOMD Strategic Campaign Operations Plan for Exploration : figure 1)
NASA talk about a need of constancy of purpose. But we can't have constancy of purpose if our goal is based on an assumption based on a knowledge gap about whether it is biologically safe for humans to land on Mars.
NASA's updated planetary protection objectives for humans to Mars reads:
1.3.2. If there is a gap between current knowledge/capability and the desired outcome as described in 1.3.1, NASA will undertake a program of activities to close the knowledge/capability gap.
(NASA, 2020, Biological Planetary Protection for Human Missions to Mars)
We have found that the only way to close the knowledge gap about whether the biosphere on Mars is safe for humans is with Sagan's exhaustive unmanned biological exploration of Mars. It is not possible to do this by using samples returned from Jezero crater.
We can achieve that constancy of purpose by retaining NASA's rightmost goal but changing the intermediate objective to a rapid astrobiological exploration of Mars from Earth and from orbit. We don't need to do the in situ resource utilization and placing of human habitats on the surface of Mars. We can do in situ resource utilization for creating fuel to drive on the surface as this is dual purpose and can be used for both humans and robots.
To avoid the risk of stranded assets on Mars we need to make sure everything we send to the surface is dual purpose for both human and robotic missions until we close the knowledge gap about whether what we have on Mars is biologically safe for human exploration or is biologically unsafe, but perhaps scientifically far more interesting such as mirror life.
We can still aim for a sustainable presence, but in Mars orbit and on the Martian moons. If we have this worked out for the Moon already we should be able to do it in orbit around Mars.
Text on graphic:
Long duration Regenerative ECLSS (Environment Control and Life Support Systems) on the Moon at polar peaks of light and in lunar caves (as in BIOS-3)Need to change to Mars orbit and martian moons Regenerative ECLSS
Graphic from (Bowersox, 2015, Human Exploration and Operations Overview: slide 8)
ADDCITES for BIOS-3 etc.
This approach is far more flexible in the future. Lessons we learn from in situ utilization on the Martian surface will need to be adapted to conditions of the Martian dust and corrosive perchlorates. It will use the carbon dioxide for in situ resource utilization and will be adapted to conditions of a near vacuum but not completely vacuum atmosphere and highly oxidising surface conditions. These are conditions only found on Mars in our solar system.
This is their current plan (NASA, 2021, HEOMD Strategic Campaign Operations Plan for Exploration : 7):
- develop a sustainable presence on the lunar surface to explore, conduct science and serve as an analog testbed for Mars
- prioritize reduced Mars mission duration
- pursue the minimum possible Mars mission architecture for humanity’s first expedition to the surface of Mars
- scientific discovery and exploration.
But Mars isn't the rightmost goal in their objective. It seems to put too much weight on Mars to have the Moon as a testbed for Mars, especially since we can't know yet if it is biologically safe to land humans on Mars.
For a more flexible mission plan that still is goal orientated, and objective based, we can replace this by:
- develop a sustainable presence on the lunar surface to explore, conduct science and serve as an analog testbed for human exploration throughout the solar system
- prioritize reduced Mars mission duration
- pursue the minimum possible Mars mission architecture for humanity’s first expedition to Mars orbit operating telerobotic avatars on the surface for a rapid astrobiological exploration of Mars and ensure all assets deployed to the purpose are dual purpose and will be useful if Mars turns out to be biologically unsafe for a human landing
- scientific discovery and exploration.
The suggestion here is to change objective 3 and modify objective 1. The rest can remain as they are.
Lessons we learn from in situ resource utilization on the Moon and the moons of Mars can be applied almost anywhere in the solar system including Mercury, the Moon, Near Earth Objects, the main belt asteroids and Callisto. So they are of far wider applicability for NASA's rightmost goal.
Text on graphic:
RECOMMENDATION:
Replace by dual purpose surface assets for a rapid astrobiological exploration of Mars in situ and do the in situ resource utilization on the Martian moons initially
Humans can be far more useful in orbit, HERRO found that one team in orbit with telepresence does as much science as three teams on the surface
Replace by inflatable habitats in orbit doing rapid exploration of Mars with telepresence avatars
ISRU in orbit and on the Martian moons is of far wider applicability for NASA's rightmost goal of humans throughout the solar system.
Crew set up base on Phobos
Crew explore Mars via telepresence from Phobos
Crew land / live on Phobos
[Similar sentences for landing / living on Mars in the graphic crossed out]
Graphic from (Bowersox, 2015, Human Exploration and Operations Overview: slide 8)
The parallels between the Moon and Mars aren't that close. There are closer parallels with asteroids, the Martian moons and Callisto. Mars has issues that the Moon doesn't have no other destination in the solar system has, such as the perchlorates in the dust, and the Martian dust storms. It has an asset, the CO2 in the atmosphere not found anywhere else.
There are benefits of the vacuum conditions on the Moon. We can do direct deposition of solar panels on the lunar surface and manufacture computer chips in situ with better vacuum conditions than anywhere on Earth.
The Moon has assets not found on Mars such as the potentially vast lunar caves up to kilometers in diameter and we know they are tens of meters in diameter, the peaks of sunlight at the poles that get solar power 24/7 for the entire year except for a few days of interrupted sunlight in winter, and the cold traps at the poles at below liquid nitrogen temperatures, which is an asset for fuel storage. The poles have near constant conditions for heat rejection too which is a major issue for explorers.
Text on graphic:
Parallels not that close, closer with asteroids, Martian moons and Callisto
Moon has assets not on Mars
- vacuum
- peaks of sunlight
- vast caves
- ultracold at poles
Mars has unique problems and
- assets not found anywhere else
- Perchlorates
- Dust storms which turn night to day for weeks on end
- CO2 as an asset
Graphic from (Bowersox, 2015, Human Exploration and Operations Overview: slide 8)
Most of the slides I commented on are from this presentation:
Video: NASA seeks input on Moon to Mars Objectives
See also: (NASA, 2023, NASA Details Strategy Behind Blueprint for Moon to Mars Exploration)
If humans could be sterilized like seeds we could land on Mars with no risk to ourselves or to native life, no matter what there is there. However, we are symbiotic with so much microbial life which can't be removed. As a result, some of the most interesting scenarios for life on Mars also make martian microbes unsafe for humans or our biosphere. In other interesting scenarios, terrestrial microbes can be potentially devastating for native martian life.
It may help to say a bit about my own personal perspective on this as the author of this book. Since a child,. I was very keen for humans to the surface of Mars, also the Moon, Mercury, Jupiter's moons, the asteroids, everywhere in the solar system, just like anyone else keen on space exploration. Based on the reasoning that led to this book, I still find a future with humans on Mars inspiring, as for a future with humans on the Moon. However, I now see a future where humans can't land on Mars as even more inspiring. I find that future even more inspiring, not because humans can't land on Mars, but because the reasons they can't land would make this a far more interesting and more valuable solar system for our civilization.
Readers may have different views about which of those they think would be a better scenario for our solar system. In any case our wishes and hopes can't change the reality of whatever there is on Mars including:
- as sterile as the Moon so that there will be no planetary protection issues involved in landing humans there,
- a novel, rich, diverse microbial biosphere. and possibly primitive multicellular life that perhaps can never be safely mixed with Earth's biosphere,
- prebiotic chemistry or early life of great interest to us that can't harm us but would quickly be erased by terrestrial life
- a novel biosphere of great interest that can mix harmlessly with our biosphere and with humans on the surface as in some of the science fiction stories.
Until we know what we have there we need to be prepared for anything we might find on Mars.
Reality check on timescale: when we have the capability to send humans to Mars safely we will also be able to run a human base on the Moon that needs resupply only every few years
NASA want to build a permanent base on the Moon. If we had the capability to send humans to Mars, we could send, a half dozen astronauts to the Moon with all the supplies they need for 3 years, and then leave them exploring and never need to send more supplies, replacement parts, etc. This would make it very easy to establish a permanent base on the Moon. It would likely cost far less than the ISS. But we are nowhere near that level of technological readiness.
It is natural for NASA to be optimistic and forward looking. However, we have never done anything resembling this. The issue is to maintain a sustained presence on the Moon. If we can't do that we are not likely to be able to do it in Mars orbit or on Mars. Although there have been many attempts, so far there have never been any long term successes for the easier task of a sustained presence of humans living beneath the shallow seas such as the Mediterranean, or in floating cities or farms on the sea. We are close to the technology to achieve such settlements but it is still not easy. To set up an outpost on the Moon is far harder.
see the Supplementary information section under:
We couldn’t send an ISS clone to Mars, not safely. It will be a major challenge to do an ISS clone on the Moon. ISS needs resupply every few months and they frequently need replacement parts from Earth when components fail.
NASA plans depend on a rapid transition from a life support system that has a mean time to failure of some life support systems of less than six months to one with a mean time to failure of over 30 months by the 2030s and that depends on returning materials back to Earth for monitoring (NASA, n.d., Environmental Control & Life Support/Fire Safety Systems Maturation Team Status : 11-14). We can do this but will it be ready as soon as the 2030s?
The life support system ECLSS is complex (NASA, 2000, Breathing Easy on the Space Station).
- CO2 and methane produced in the intestines.
- Ammonia from breakdown of urea in sweat
- acetone, methyl alcohol and carbon monoxide, in urine and breath (byproducts of metabolism).
This diagram is from 2001 but not much has changed. We have yet to test a new system designed from scratch with modern technology for long duration spaceflight.
Caption: The ISS Environmental Control and Life Support Systems (ECLSS)
(NASA, 2000, Breathing Easy on the Space Station)
This shows some of the capabilities that we need to develop for deep space missions such as to Mars. For instance the mean time to failure of some life support components of the ECLSS on the ISS is less than six months, they often need replacement from Earth. This needs to be increased to over 30 months for deep space missions.
Caption: The ISS Environmental Control and Life Support Systems (ECLSS)
(NASA, n.d., Environmental Control & Life Support/Fire Safety Systems Maturation Team Status)
An exploration class ECLSS for travel to Mars needs to be more self contained.
Caption: The ISS Environmental Control and Life Support Systems (ECLSS)
(NASA, n.d., Environmental Control & Life Support/Fire Safety Systems Maturation Team Status : 10)
Some of the challenges are summarized here: (NASA, n.d., Environmental Control & Life Support/Fire Safety Systems Maturation Team Status : 11-14)
The main risks identified for the ISS, by way of example, are:
• MMOD [Micrometeoroid and orbital debris damage] penetration of the ISS pressure wall or other critical hardware
• a catastrophic collision with the ISS by a visiting vehicle or robotic arm
• an on-board fire that results in loss of crew life or the ISS vehicle
• a toxic spill in the crew-habitable volume
• a catastrophic system failure
• a hardware or software design flaw that results in crew or vehicle loss
• a crew member becoming separated from the Station during an EVA or MMOD
penetration of an EVA crew member’s spacesuit
• a deliberate attack using ground assets to issue Station commands leading to a
catastrophic condition
• an inadvertent critical command from the Mission Control Center (MCC)(NASA, 2007, Final report of the International Space Station Independent Task Force)
A lunar surface colony will have many challenges not faced in the ISS. For instance, the astronauts in the ISS do about 10 to 20 space walks a year (NASA, n.d., Spacewalks).. Astronauts in a lunar settlement is likely to do many more.
EVAs on the Moon will involve walking and driving in a very dusty environment, very different conditions from the ISS microgravity space-walks. The Apollo spacesuits were so damaged by the lunar dust that after about three days they were close to unusable. How well will modern spacesuits function on the lunar surface? The lunar dust is likely to lead to many challenges, and we have solutions such as the suitport but these can't yet be tested on the Moon. Some of these issues are touched on in the Supplementary information section under:
Then we have no experience in running a life support system with no resupply from Earth for years at a time. But we will need this for a permanent base on the Moon especially if the plan is for a blueprint for more distant settlements in the solar system.
Then if the Apollo astronaut experiences are a good guide, we can expect the first lunar astronauts to face emergencies nobody foresaw and to need to respond rapidly o on many occasions. The Apollo astronauts would all have died on several occasions, if they hadn't been highly trained test pilots able to respond without panic to extreme emergencies. Everything will be new for the first missions to the Moon, and as they explore the surface, lunar caves, mountains and craters. They need to be ready in case spacesuits may fail, rovers break down, habitats lose integrity, there may be chemical releases, fires and explosions. We have had emergencies on the ISS with the most dangerous perhaps the near drowning of the Italian astronaut Luca Parmitano in his spacesuit after a leak of water from the plumbing of his spacesuit. (NASA, n.d., Investigation of EVA 23 Resonates at PK Expo).
Video: Luca Parmitano and Chris Cassidy explain what happened during EVA 23
Luca Parmitano ends his account of his near drowning saying we are explorers in space not colonizers:
By now, the upper part of the helmet is full of water and I can’t even be sure that the next time I breathe I will fill my lungs with air and not liquid. To make matters worse, I realise that I can’t even understand which direction I should head in to get back to the airlock. I can’t see more than a few centimetres in front of me, not even enough to make out the handles we use to move around the Station.
…
“Space is a harsh, inhospitable frontier and we are explorers, not colonizers. The skills of our engineers and the technology surrounding us make things appear simple when they are not, and perhaps we forget this sometimes. Better not to forget.”
(ESA, 2013. EVA 34: Exploring the Frontier)
At our current level of technology and knowledge, as explorers for now not yet settlers, the conditions on the Moon are far more challenging than for the ISS.
The ISS crew have lifeboat spacecraft attached to the station. Though it’s never been needed, they can get back to Earth in a few hours if they have a fire, chemical release, explosion, or a micrometeorite that damages the ISS to the extent they can’t repair it and need to evacuate right away. When we explore the Moon it will be a two day MEDVAC back to Earth. For missions to Mars it’s nearly two years MEDVAC if there is an accident just as the crew are leaving Earth on their way to Mars.
Then there is the issue of maintaining public support. The Apollo program stopped as a result of the public losing interest. Eventually private space may be able to pay for missions to the Moon based on commercial enterprises such as tourist hotels on the Moon but this seems to be a little way into the future yet. Before it is realistic to send humans as far as Mars we need sustained public support for a lunar mission for long enough to develop and test the necessary technology.
What can help here are many discoveries since Apollo which have shown the Moon to be far more interesting than we realized at the time of Apollo. However if we pay attention to our most experienced astronauts, it seems that we should be cautious about setting a date for when we expect to be ready to send humans as far as Mars.
Chris Hadfield, former ISS commander says Mars is for the next generation of astronauts
The Apollo astronauts made it look easy - but that was because they were experienced test pilots who could make quick decisions in an emergency without even a moment of panic - like when the Apollo 10 astronauts got within a few tens of seconds of crashing into the Moon (Teitel, When Apollo 10 Nearly Crashed Into the Moon) or when Buzz Aldrin found a way to take off from the lunar surface using a felt tip pen (Kennedy Space Center, 2019, Tell Me A Story: Buzz Aldrin's Felt Tip Pen Saves Day)
The retired Canadian astronaut Chris Hadfield, former commander of the ISS, interviewed by New Scientist, put it like this:
"I think ultimately we’ll be living on the moon for a generation before we get to Mars. If the world and the moon were threatened and the only way to preserve our species was to launch from Earth, we could go to Mars with yesterday’s technology, but we would probably kill just about everybody on the way."
(Klein, 2017, (Chris Hadfield: We should live on the moon before a trip to Mars)
It’s as if you and I were in Paris, paddling around in the Seine in little canoes saying, “We’ve got boats, we’ve got paddles, let’s go to Australia!” Australia? We can barely cross the English Channel.
Frame from 28 seconds into this ESA video: Moon Village
NASA's design reference architecture for Mars goes into this in technical detail. The Key Challenges section runs from page 64 to 75. This is followed by Risk Mitigation Strategies which runs from pages 75 to 77 focused on robotic missions to Mars and missions to the Moon to field test and use the hardware and systems that would later be used on Mars if we do land humans there (Drake et al., 2010, Human exploration of Mars, design reference architecture 5.0).
The Moon is a place where we can make our first steps in sustainable living in space, within easy access of Earth for repairs, supplies, and emergency MEDVAC back to Earth in only two days. It's far more interesting than we realized in the 1960s and 1970s.
The lunar caves are truly vast far larger than lava tube caves on Earth. Some may be up to kilometers wide. Some of the lunar caves probably have an internal steady temperature of around -20 °C, potentially useful as a constant heat sink for a settlement (Daga et al., 2009, Lunar and martian lava tube exploration as part of an overall scientific survey). The challenge of providing energy during the lunar night is a similar challenge to providing energy during martian dust storms. Then there are the peaks of almost eternal light at the poles with solar power 24/7 nearly year round (Foing, 2005, Peaks of Eternal Light), the polar ice and so on (NASA, 2019, Moon’s South Pole in NASA’s Landing Sites).
The Moon is also far easier to develop commercially with current technology, for instance it is within easy reach from Earth for tourism, and export from the Moon to Earth is potentially far easier. Depending on whether that potential is realized in the near future, with tourism, mining and so on, we may have hundreds to a few thousand permanent or temporary residents on the Moon by the time we are ready to make that big and difficult step onwards to Mars. If the Moon is developed commercially by then, that wealth of experience in living in space conditions on the Moon will make it far easier to travel to Mars in a reasonably safe way, either to orbit around Mars or to land on the surface.
The ice at the poles of the Moon could be the "Record keepers of the early solar system" as Greg Delory put it (Thomspon, 2019. 'Significant Amount' of Water Found on Moon). They will have meteorites from Mars and indeed likely from early Venus too that have been preserved frozen at liquid nitrogen temperatures for millions and even for billions of years.Then there is more ice offset from the north and south pole. This may be ancient deposits from over three billion years ago before the volcanic activity, which changed the polar axis slightly by shifting material (Hand, 2016. Update: Lopsided ice on the moon points to past shift in poles).
This gives the potential for many exciting discoveries on the Moon relevant to astrobiology.
- Possibility of an ancient regolith layer buried beneath later lava preserving actual organics from the first few hundred million years of Earth evolution (Spudis, 2015. Ancient Life on the Moon — From Earth).
- Suggestion that the lunar ice at the poles could preserve ancient biogenic organics (Schulze-Makuch, 2012. Organic Molecules in Lunar Ice: A Window to the Early Evolution of Life on Earth)
- Suggestion that effects of cosmic radiation on the ices and other chemicals at the poles of the Moon may be creating complex organics - not quite life probably but the ingredients for life (Brownell, 2014. Seeds of life can sprout in moon's icy pockets).
- Possibility of meteorites from Earth on the Moon - which again, if they landed in frozen areas of the Moon, may preserve organics from millions or even billions of years. They could also preserve fossils as fossil diatoms are still recognizable, and the smallest ones intact after a simulated impact on the Moon (Burchell et al., 2017. Survival of fossilised diatoms and forams in hypervelocity impacts with peak shock pressures in the 1–19 GPa range)
- It may also have early rocks from Mars, Venus and small bodies in the early solar system, including possibly biological evidence from those objects also (Crawford et al., 2012. Back to the Moon: The Scientific Rationale for Resuming Lunar Surface Exploration : 3.1.1)
- It may also record the composition of the solar wind, galactic environment, and volatiles from comets in the early solar system. See again (Crawford et al., 2012. Back to the Moon: The Scientific Rationale for Resuming Lunar Surface Exploration : 3.1.1)
- Must have organics from meteorite and comet impacts at least and probably from the solar wind too which brings not just hydrogen but also heavier elements to the Moon.
So for those who consider it important to have a colonization frontier in space, the Moon is an exciting and interesting first step to explore, and many of the capabilities we develop on the Moon can be developed further in space settlements on Phobos without the inconveniences of the surface of Mars with its dust storms and perchlorates and the physical dangers of a mission landing on the martian surface.
Dust as the great inhibitor on Mars and the Moon
Lunar dust isn’t laced with perchlorates like the Martian dust, but it was still a major issue for lunar astronauts. They all reported difficulties with the dust (Stubbs et al, 2007).
Astronaut Eugene Cernan, the last man to walk on the Moon to date (NASA, 2017, Remembering Gene Cernan), described the lunar dust as one of the greatest inhibitors to a nominal operation on the Moon (Levine, 2020. Lunar Dust and Its Impact on Human Exploration: Identifying the Problems)
“I think dust is probably one of the greatest inhibitors to a nominal operation on the Moon. I think we can overcome other physiological or physical or mechanical problems except dust.
One of the most aggravating, restricting facets of lunar surface exploration is the dust and its adherence to everything no matter what kind of material, whether it be skin, suit material, metal, no matter what it be and its restrictive friction-like action to everything it gets on., metal, no matter what it be and its restrictive friction-like action to everything it gets on,”
Caption: Gene Cernan inside the lunar module (NASA, n.d., Eugene Cernan in Lunar Module). The dust got everywhere. He was particularly dusty because of an incident with a broken fender on the lunar rover (Phillips, 2008, Moondust and Duct Tape)
The dirt got into the knees and the seat of the suit, and the upper arms too, perhaps when the astronauts supported themselves when they fell over on the lunar surface. This is something that needs to be considered in future lunar and Mars spacesuit designs (Christoffersen et al., 2009. Lunar dust effects on spacesuit systems: insights from the Apollo spacesuits)
Caption: Concentrations of titanium (in units of 100 ppm) in the suit fabric for Jack Schmitt’s suit for Apollo 17. This seems to be a good tracer of the lunar dust. The lunar dust got embedded deep in the woven fabric of the suits causing significant damage after just three days EVA on the surface.
Mars has worse dust problems than the Moon. For instance the Moon doesn’t have dust storms. Several times a decade dust storms on Mars block out the sunlight for weeks making surface conditions as dark as night.
Our rovers on Mars haven't yet traveled fast enough to create their own dust clouds but fast moving rovers or even people walking would throw the dust up into the air, as fine as cigarette ash, similarly to the arcs of dust thrown up by rovers on the moon.
Caption: Clouds of lunar dust thrown up by the rover, during Apollo 16. On Mars the dust is as fine as this, but it would linger in the air and not fall back in ballistic arcs as it did on the Moon (NASA, 1972, Apollo 16 lunar rover "Grand Prix"(stabilized)). Frame is from 1:11
Lunar dust falls back in ballistic arcs, in an atmosphere so thin it is classified as an exosphere rather than an atmosphere.
Lunar dust is more easily managed than Martian dust and likely similar to dust found on larger asteroids and perhaps the regolith of Phobos too
The vacuum conditions on the Moon are common for small solar system bodies and the lunar dust isn't confined to the Moon either. Smaller asteroids like Bennu are rough and dust free in photos because electrostatic forces levitate the fine dust which is lost to space.
But larger asteroids like Eros are smooth and likely covered in dust like the Moon (Hsu et al., 2022, Fine-grained regolith loss on sub-km asteroids) Press release: (Strain, 2022, Hopping space dust may influence the way asteroids look and move). So dust mitigation for a lunar base helps prepare for exploration, and perhaps settlement and operations on the larger asteroids
So a lunar base helps prepare for exploration, and perhaps settlement and operations on the larger asteroids. They also help prepare for ISRU on Mars's moons Phobos and Deimos, with
Phobos is intermediate in size between Bennu and Eros and it's surface also seems intermediate in smoothness, with a mix of smooth and sharp features. This shows Phobos at a resolution of 4.4 meters per pixel, though the image will be autoresized to fit the page. (ESA, 2010, Phobos flyby images). Original width of this image 1258 pixels which would correspond to about 5.5 kilometers in width.
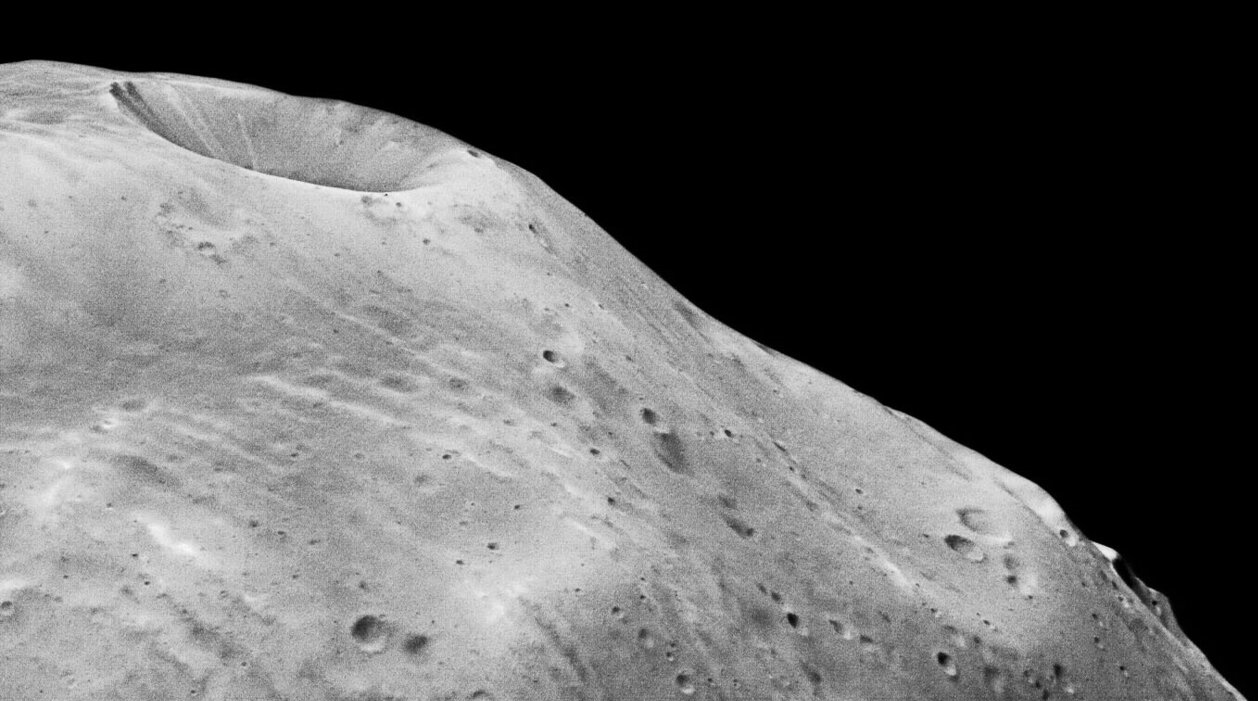
However the dust on Mars itself behaves differently. Mars has atmospheric phenomena such as the dust devils. It has the global dust storms. The finer dust can remain suspended for a long time if kicked up. The dust storms can block out sun and turn day to night. There are light winds blowing nearly all the time which at some times of the day carries bouncing dust grains with them too.
It's also different in composition, laced with perchlorates which aren't found on the Moon and which can be turned by the UV into chlorates and chlorites all of which have human health implications unique to Mars.
First, on Mars, some of the finest dust kicked up by astronauts or rovers would linger in the air for some time even in calm weather without winds. For large particles with little atmospheric drag, the height of the ballistic arcs on Mars and the Moon will be the same if g/V2 is the same where g is the acceleration due to gravity and V is the initial velocity with which the dust is kicked off the ground by equation 5 of (Hsu et al., 2012. Ballistic motion of dust particles in the Lunar Roving Vehicle dust trails : 455). So the arcs will be the same shape and height if the dust was kicked up one and a half times faster on Mars than on the Moon (calculated as sqrt(3.72/1.62) where 3.72 m/s² and 1.62 m/s² are for Mars gravity and the lunar gravity respectively).
The Apollo astronauts drove the lunar rover at around 10 km / hour (Hsu et al., 2012. Ballistic motion of dust particles in the Lunar Roving Vehicle dust trails) so as a rough estimate, a Mars rover driving at around 15 km / hour in similar conditions would produce similar height ballistic arcs to the lunar rover for large particles. To get the same height arcs on Earth we need to travel at sqrt(9.801/1.62) or about 2.46 times faster or about 24.6 km / hour
Dust of 1 mm diameter settles at a speed of about 5 meters per second sp wouldn’t linger in the atmosphere for long in calm weather. However, a one micron particle will take half an hour to fall a meter in Martian conditions (Fuerstenau, 2006. Solar heating of suspended particles and the dynamics of Martian dust devils : Figure 1), Fuerstenau et al. were looking at solar heating and dust devils so it's a different situation from dust thrown up by wheels or walking, The wheels wouldn't throw the finer dust up as high as on the Moon because of the higher gravity and air resistance but it would linger in the air for longer.
Then there's another wind driven factor, the bouncing dust grains. Quite large grains of dust can bounce across the dunes at half a millimeter in diameter. They can keep going at wind speeds far less than the speeds needed to kick them off the surface unlike the situation on Earth where the wind speed needed to start the grains bouncing is only a little over the wind speeds to keep them bouncing (Vasaveda, 2020. Mission Overview and Scientific Contributions from the Mars Science Laboratory Curiosity Rover After Eight Years of Surface Operations : 4.2). This means that if astronauts while driving around or even walking kick dust into the air the larger dust grains may start bouncing and keep bouncing for hours in the light winds.
We covered this in A way for microbes travel great distances on Mars in bouncing sand grains, up to half a millimeter in diameter
In short, as soon as we start having rovers on Mars traveling as fast as the lunar rovers or faster we can expect to get a fair bit of dust thrown up. The larger particles will follow paths similarly to those on the Moon though needing a slightly faster speed for the same height. The smaller ones may not be thrown up so far but would linger longer. Meanwhile if there is some wind blowing, some of the faster large dust grains thrown up may continue bouncing for a long time until the wind falls again. Our rovers on Mars so far travel far too slowly to observe these effects of their own motions.
Then the perchlorates in Martian dust, although useful as a resource for colonists to make fuel and oxygen, would also be potentially harmful. Perchlorates interfere with regulation of the thyroid gland (by impairing uptake of iodine). (Davila et al., 2013. Perchlorate on Mars: a chemical hazard and a resource for humans).
- Inhaling a few milligrams of Martian dust could exceed the recommended maximum daily dose for perchlorates (Reference dose or RfD)
- When the perchlorates are activated by ionizing radiation they may change to the more deadly chlorates and chlorites with some potential for more serious and immediate effects such as respiratory difficulties, headaches, skin burns, loss of consciousness and vomiting.
The challenges to keep the dust out impact on most aspects of a mission to the surface (Rucker, 2017. Dust storm impacts on human Mars mission equipment and operations). There are solutions.
This doesn't make Mars colonization impossible. There are methods for dealing with this, used for dust suppression when mining uranium, lead or other heavy metal contaminated areas. But it adds to the complexity of Mars colonization (Davila et al., 2013. Perchlorate on Mars: a chemical hazard and a resource for humans).
The suitport may be a solution for the problem of dust inside habitats and this would be useful on the Moon too and more widely on any dusty asteroids or moons (Boyle et al., 2013. Suitport feasibility-human pressurized space suit donning tests with the marman clamp and pneumatic flipper suitport concepts).
Caption: Suitport illustration from (Gernhardt et al., 2008. Health and safety benefits of small pressurized suitport rovers as EVA surface support vehicles) reduces loss of air when exiting or entering the rover and greatly reduces the dust problem.
We can test the effectiveness of suitports for keeping out dust on the Moon. But it's not an exact analogue for the situation on Mars.
These are issues unique to Mars and much of what we learn is only applicable to Mars. We won't face perchlorates or saltation or dust storms anywhere else in the solar system except on Mars and Earth. Possibly surface operations might lead to suspended dust in the atmosphere of Saturn's moon Titan but the atmosphere there is denser than Earth's and it has almost no wind at ground level and very different conditions given the cold.
It is easier to deal with the dust on the Moon because it doesn't have dust storms. Also the dust is different.
Larry Taylor was lucky enough to get hold of enough of the genuine lunar dust from Apollo to experiment with and he found it was as easy to make the dust into glass as it is to boil a cup of tea with a microwave. This doesn't work with the regolith simulants. The genuine lunar dust is mingled with iron intimately as a result of space weathering by micrometeorites and that's why it is so easy to microwave (NASA, 2005, Lunar Lawn Mower). This is a beneficial side effect of all the micrometeorite impacts on the Moon (which you don't get so much on Mars with its thin atmosphere, just enough to filter out micrometeorites). The Moon's "soil" or regolith contains large quantities of glass, created during the impacts. It also has free iron, at half of one percent of the soil, in tiny micro beads of iron (nanophase iron) which concentrate the microwave energy. Mars doesn't have these microbeads of pure iron, only iron oxides.
Larry Taylor found that it took only 30 seconds to melt a small lunar sample at 250 watts (typical of a domestic microwave). He found that we can melt the soil to glass as easily as we can boil water using microwaves. This only works with genuine lunar soil and not the simulants. We have nothing analogous to lunar soil on Earth,. He says the microstructure of the genuine lunar regolith, with nanophase iron beads scattered throughout, would be almost impossible to simulate (Taylor et al., 2005, Microwave Sintering of Lunar Soil: Properties, Theory, and Practice).
- Large areas of the surface round a lunar base can be sintered as can any
landing pad and possibly simultaneously turned into solar panels adding to the power for the base, and the same could likely be done on Phobos.
- humans on Mars could also similarly construct flattened areas around their bases and landing pads to reduce the dust problems, the Martian dust devils and dust storms will continue to deposit dust on and around the base. Also they won't be able to use this microwave sintering method.
- Looking further into the future, it would also be possible to construct long distance railways and
highways on the Moon which would remain dust free for millennia after they are constructed.
- Any long distance transport routes on Mars would need to be cleared of dust regularly to reduce the dust clouds that would be produced by fast land based transport on Mars. These also are unique problems to Mars not found anywhere else in the solar system.
There would be many such issues., We can expect them to be solvable problems but unique to Mars, and so not particularly relevant to facilitating NASA's end goal of humans throughout the solar system,
None of this makes Mars settlement with humans impossible, but Mars does not seem to be an optimal place to colonize for its own sake. If we find we can't attempt to colonize Mars it may not be such a drawback as one might at first expect.
We can grow food in greenhouses on Phobos in low gravity as easily as on Mars and if Mars has hazardous indigenous life, we can grow it on Mars by telepresence
CO2 is a waste gas to be scrubbed in human habitats. In a closed system the CO2 exhaled by humans plus a small amount from processing excreta exactly balances the CO2 taken up by crops as they grow. if any food needs to be imported astronauts exhale more CO2 than is needed to grow their crops.
Text on graphics: Greenhouses would work as well on PHobos as on Mars.
Crops grow well in microgravity in all the tests so far in space.
Combines photo of Phobos rotated 180 degrees (NASA, 2008, Phobos) with a photograph of the Arizona prototype lunar greenhouse (Story, D., n.d., Prototype Lunar Greenhouse) and Hubble photo of Mars (Hubble, 2003, Photograph of Mars taken by the Hubble Space Telescope during opposition in 2003)
The external Martian atmospheric pressure at less than 1% of the terrestrial atmospheric pressure has little effect on the engineering to contain even a low pressure greenhouse for plants only (not breathable) at 10% of terrestrial atmospheric pressure (Bucklin et al., 2002, Low Pressure Greenhouse Concepts for Mars: Atmospheric Composition : figure 6).
We have already designed almost self-sustaining space habitats like the early Russian BIOS-3 based on plants grown for food, and oxygen. The plants in turn take up carbon dioxide and water from humans, and our metabolisms use precisely the amount of oxygen that is generated by the plants we eat as they grow, making for a self sustaining closed system. This should work in space, a more challenging situation than an enclosed habitat in a world dominated by mirror life (Salisbury et al., 1997. Bios-3: Siberian experiments in bioregenerative life support) (Johansson, M., 2006. Living in Space: A Comparative Study of one Conventional Life Support System and two Biological Systems).
The BIOS-3 experiments were able to supply nearly all the food needed from only 30 square meters growing area per person. This suggests a space colony could be largely self-sufficient in food at an early stage. If astronauts produce enough crops for their food, the crops produce the same amount of oxygen as the astronauts consume eating the food. The water can be recycled too. So in principle a space colony anywhere in the solar system can be largely self sufficient in agriculture and life support so long as it has a sufficient supply of energy for the lighting, for instance from sunlight. It doesn't have to be on a terrestrial planet to do that.
We now have the results of many experiments in growing crops in microgravity in the ISS. It used to be thought that we needed artificial gravity to grow crops but it's become clear we don't. NASA's VEGGIE system was the first designed to test production of food crops in microgravity rather than plant experiments and was used to produce small amounts of food for the ISS crew (Zabel et al., 2016. Review and analysis of over 40 years of space plant growth systems : 8).
These crops have all been tested and grown successfully in microgravity in small scale experiments (Zabel et al., 2016. Review and analysis of over 40 years of space plant growth systems : 14, table 6). :
- Bean
- Beet
- Cabbage
- Carrot
- Chinese lettuce
- Different herbs
- Dill
- Kale
- Lettuce
- Nut sedge
- Onion
- Pea,
- Pepper
- Radish
- Rice
- Salad species
- Soybean
- Spinach / New Zealand spinach
- Strawberry
- Swiss chard
- Tomato
- Turnip
- Wheat
However many candidate crops recommended by researchers haven't yet been tested in space (Zabel et al., 2016. Review and analysis of over 40 years of space plant growth systems : 14, table 6). :
- Broccoli
- Bean
- Beet
- Canola
- Chickpea
- Chili pepper
- Collards
- Dandelion
- Endive
- Lentil
- Nut sedge
- Peanut
- Potatoes
- Sweet potatoes
- Tambala
- Taro
More importantly none of these crops have been tested in growing areas large enough to test large-scale food production This is a summary of the situation in space agriculture as of 2016:
In the past 40 years more than 20 plant growth systems were utilized to grow over 40 different plant species in space. More than 50 different plant experiments were conducted in space.
However, to grow enough plants for supplying a crew with a substantial amount of fresh food more research and development is necessary. Especially the available grow area in space has to be significantly increased to address research related to large- scale food production (e.g. procedures, automation).
As shown in this paper, there is a large discrepancy between the plants recommended for space greenhouses and the plant species actually grown in space. Plant experiments in space have to be focused on food crops rather than model plants. Until there is enough cultivation area available in space, researcher and engineers can rely on space analogue field campaigns to test integrated systems capable of cultivating food crops in a controlled closed environment.
(Zabel et al., 2016. Review and analysis of over 40 years of space plant growth systems : 14).
Other In Situ Resource Utilization (ISRU) tasks are similar and can be done just as easily on Phobos such as finding radiation shielding from the martian moons. Constructing habitats in microgravity has advantages as well as disadvantages. It is easier to move things in microgravity.
The gravity of Phobos is enough to prevent materials being lost accidentally into space, though it would be easy to throw things fast enough to leave the surface with an escape velocity of 11 meters per second, 41 km / hour or 25 mph (NASA, n.d., Phobos: Facts & Figures). At that speed, in principle Usain Bolt at his fastest speed of 44.72 km/hour(Nag, 2023, Usain Bolt’s records: best strikes from the Lightning Bolt) was fast enough to reach escape velocity except even he couldn't because there would be no way to sprint on Phobos.
There would need to be some care to make sure that humans don't accidentally contaminate Mars with debris from Phobos though small items would burn up in the martian atmosphere or heat up so much they are sterilized.
This ISRU on the martian moons is of far wider applicability in our solar system than ISRU on the martian surface. However, even if it is not biologically safe to land humans on Mars, later we can grow crops on the surface of Mars via telepresence. Seeds can be sterilized of microbial life (unlike humans).and can be grown in sterile aquaponics.
This might be economically viable if production of fuel on the surface is easy to do in an automated way.
Though we can’t sterilize humans, we may be able to sterilize seeds without any risk of contaminating Mars with Earth micro-organisms.
Text on graphics: We may be able to grow plants on Mars even if it has mirror life that can never be brought back, or Earth has microbes that can never be sent to Mars.
Seeds can be sterilized and grown in sterile hydroponics
In this way we could have greenhouses on the surface of Mars and these could grow food for the colonists in orbit.
Astronauts in orbit may have plenty to eat in their habitats anyway by then, but they might grow delicacies or medicinal plants and anything else of high value worth the extra work of export from the martian surface. Also they might grow large plants, and maybe trees in the Mars light gravity or whatever else grows best on the surface of Mars. Or if export from Mars is easy by then, simply grow crops on the surface of Mars for convenience to save space in orbiting habitats.
We can in principle grow many terrestrial crops on the surface of Mars with no risk of contamination in either direction, on most scenarios. We could export those crops to the orbiting colonies, on Phobos, or colonies in free orbit around Mars or further afield.
Chris McKay has suggested we do a proof of concept experiment to start with, sending a sterilized seed to Mars to grow a flower there.
The best design for a plant growth module for Mars would make use of the Martian soil, with nutrients added as necessary. Carbon dioxide and water would be obtained from the Martian atmosphere, and the natural sunlight on Mars would provide for photosynthesis. Because of the lower pressure on Mars, the plant would need to be in a small pressure vessel - its own little spacesuit.
The design of this miniature greenhouse would allow light to enter and, true to its name, provide greenhouse warmth during the day. At night the growth module may need to draw on heat generated by the main spacecraft to keep the plant warm. The plant's growth and flowering would be monitored using the lander camera.
Initial designs by groups at the University of Colorado and the Jet Propulsion Laboratory have shown that such a unit can be constructed. We could therefore send life to Mars on the next lander.
...
The planetary protection guidelines do not explicitly prevent the conrolled transport of biological materials to Mars or the use of biological materials in controlled experiments aboard spacecraft. A plant growth unit could be constructed in accordance with the bioload limits of the present planetary protection policy. In fact, to be sure it functions as intended, the system might well exceed these limits and even be treated to eliminate nearly all bacteria.
By developing ways to send life to Mars consistent with the goals of the planetary protection policy, a near-term plant experiment would pave the way for future research and study on the planet. Most important, the growth of a single flower on Mars would be a powerful symbol of the long-term goal of expanding life beyond the Earth, first to Mars and then elsewhere. It would rival the image of the Earth from space - the pale blue dot- as a symbol of our place and future in the universe.
(McKay, 2000. Flowers for Mars : 5)
With a Mars HOTTech, a plant-growth unit could be heated to 300°C as for the sterile landers for a few minutes to remove any possibility of viable life. Then we need to find a way to introduce a single seed which is also sterile of any other life. This may need close attention since the methods for sterilizing seeds for sterile hydroponics may not leave the seeds sterile enough for planetary protection standards especially if the aim is 100% confidence that no life is introduced to Mars. Perhaps we could do the experiment initially on the Moon and use seeds that are the results of several generations of sterile hydroponics on the Moon, sterilizing the seeds each time, and confirm that there is no DNA in the seed apart from the plant DNA before sending them to Mars?
Caption in original article: It will probably happen-humans will take the first steps to terraforming Mars. A good way to test the planet's biological friendliness is to export life there. An experiment as close as the next lander could grow a single flowering plant from seed in Martian soil. Illustrations: Douglas Shrock
See also slide from (McKay, 2010. Video: Biologically Reversible Exploration (video) : 5)
Then if we find novel biology on the surface of Mars, we can do work to make Mars more habitable for the martian microbes. See above:
It might also be useful for us to grow the Martian microbes on the martian surface. They may produce unique products that terrestrial biology can't make and that are complex or impossible to synthesize on Earth.
It may be both safer and more convenient to produce any unique products of martian biology on the surface of Mars in martian conditions in habitats optimized for the martian biology, and then the products exported to space. If the products are novel bioactive compounds and drugs they could be high value low mass exports that we could profitably grow on the surface of Mars rather than to grow them (or try to grow them) by replicating surface conditions in orbit.
What about the potential for viable life on Phobos? I don't find any suggestion for habitats on Phobos but it could have still life that got there from Mars in the last few million years, and remains dormant but still viable.
Any life in martian meteorites DOES get here better protected and faster than in samples returned from the top two centimeters of the MOONS of Mars: Phobos or Deimos
NASA's “better protected and faster” comes from the planetary protection reasoning for a sample from Phobos. There it is a valid argument, because the Phobos sample and the Martian meteorites have had a similar history since they were on Mars (SSB, 2019, Planetary protection classification of sample return missions from the martian moons : 38 ff)
The most recent opportunity for any life to get from Mars to Phobos OR to Earth was after the impact that formed the Zunil crater on Mars around a million years ago (direct crater count suggests 700,000 years ago) (Hartmann et al., 2020, Do young martian ray craters have ages consistent with the crater count system? : 626).
So any meteorites from Mars arriving at Earth today from the Zunil crater ejecta spent at least the last several hundreds of thousands of years in space, while any sample we return from Phobos take longer than the meteorites arriving today on Earth, because they left Mars at the same time and haven’t yet got here.
Then as for “better protected”, our martian meteorites last left Mars at least 700,000 years ago (ejection ages between 0.7 and 18.5 million years ago (Udry et al., 2020. What martian meteorites reveal about the interior and surface of Mars : table S4). So, t hey compared two chains of events, a Phobos sample return and transfer on a meteorite.
We don’t know how long it took exactly, but our best estimate is 700,000 years old for the Zunil crater, so let’s use that figure to help illustrate how they are an exact parallel of each other:
- Phobos sample return: Ejection from Mars → Shock of impact on Phobos → Remains in top 10 cm of the Phobos surface for 700,000 years → returned to Earth in the Phobos sample return
- Meteorites from Mars arriving today: Ejection from Mars → spends 700,000 years in space traveling from Mars to Earth → fireball of reentry into Earth’s atmosphere and delivered to Earth.
They found that the amount of sterilization is similar for ejection in both cases. Martian meteorites need a higher ejection velocity to reach Earth than to reach Phobos but a small percentage of the ejecta is only weakly shocked so the difference between the two cases is modest. (SSB, 2019, Planetary protection classification of sample return missions from the martian moons :59)
The amount of sterilization between ejection from Mars and arrival on Earth is also similar in both cases, as the samples get similar ionizing radiation, whether resting on the surface of Phobos or traveling to Earth in a meteorite.
There are two differences
- Phobos sample: Shock of impact on Phobos
- Mars meteorite: Fireball of re-entry into Earth’s atmosphere
They found that any microbes from Mars would be far more sterilized by the shock of an impact into Phobos than a reentry fireball to Earth because only the surface of the rock is heated (SSB, 2019, Planetary protection classification of sample return missions from the martian moons :40)
For this part of the calculation, the committee assumed a 10% survival of microbes (underestimating a likely 80 to 100% survival) (SSB, 2019, Planetary protection classification of sample return missions from the martian moons :40)
The Phobos sample return study estimates that about 100 kilograms of Martian meteorites arrives every year and that about 100,000 tons of material have been delivered to Earth from the Zunil impact in the last million years.
Compared to these figures the amount of material returned from the surface of Phobos will be small.
This is the backward contamination version of Greenberg’s “Natural contamination standard”
"As long as the probability of people infecting other planets with terrestrial microbes is substantially smaller than the probability that such contamination happens naturally, exploration activities would, in our view, be doing no harm. We call this concept the natural contamination standard."
(Greenberg,et al., 2001. Macroscope: Infecting Other Worlds)
The reasoning is that if Earth frequently encounters Martian life anyway, we have no need to protect Earth with special precautions,
The Phobos sample return analysis may have a slight omission here for photosynthetic life which lives near the surfaces of rocks and so is more vulnerable to the fireball of re-entry than most life. But if so, it seems a minor issue because the meteorites we have from Mars also likely come from over 1 meter below the surface in the very cold dry southern uplands and modelling suggests 50 to 100 meters depth. See the supplementary data:
There may be a slight omission in the Phobos sample return discussion of the fireball of re-entry as their 10% figure is based on life that inhabits the interior rather than just the surface of rocks. (SSB, 2019, Planetary protection classification of sample return missions from the martian moons : 40). The astrobiologist Charles Cockell tested the blue-green algae chroococcidiopsis. When he attached it at a typical growing depth on a re-entry aeroshell, he found that not only the algae, but all its associated organics were destroyed. He concluded
… Thus, the planetary exchange of photosynthesis might not be impossible, but quite specific physical situations and/or evolutionary innovations are required to create conditions where a photosynthetic organism happens to be buried deep within a rock during ejection to survive atmospheric transit.
(Cockell, 2008, The Interplanetary Exchange of Photosynthesis : 5)
The question here is, could there be viable photosynthetic life on the surface of Phobos, which can’t get to Earth in a meteorite.
I.e. life that is
- on Phobos in a surface layer of a rock
- living in a rock layer that would be destroyed during the re-entry fireball if it got to Earth on a meteorite
First, it helps that some photosynthetic life near the surface of the ejected rocks may be sterilized or destroyed already by the fireball of exit from Mars on its way to Phobos.
However the details here are uncertain because the total mass ejected by the Zunil impact was about 30% of the mass of the atmosphere above it, which could be enough to shield some of the ejecta from aerodynamic heating (SSB, 2019, Planetary protection classification of sample return missions from the martian moons : 27). This would seem to leave a possibility that some photosynthetic life could survive on the surface of a rock on Phobos.
However, second, all our martian meteorites come from locations where photosynthesis is unlikely.
They were
- thrown into space by glancing collisions into the high altitude southern uplands (Tornabene et al., 2006. Identification of large (2–10 km) rayed craters on Mars in THEMIS thermal infrared images : Implications for possible Martian meteorite source regions.) where the thin atmosphere makes ejection to Earth easier.
- from at least 3 meters below the surface by the low levels of radioisotopes produced by cosmic radiation(Eugster et al., 2002, Ejection ages from krypton‐81‐krypton‐83 dating and pre‐atmospheric sizes of martian meteorites : 1355). Impact modeling may suggest a depth of 50 to 100 meters below the surface
for a typical impactor half a kilometer in diameter that makes a crater 10 kilometers in diameter (Nyquist et al., 2001, Ages and geologic histories of martian meteorites : 152). There’s other confirmatory evidence that they come from at least 1 meter below the surface (Elliott et al., 2022, The role of target strength on the ejection of martian meteorites : 3) because they don’t show any sign of ionizing radiation from the sky on one side of the rock.
Third, there is yet another twist here to look at if we want to be as thorough as possible.
- The remarkable blue-green algae chroococcidiopsis can use alternative metabolic pathways to grow underground without any light (Li et al., 2020. Recycling and metabolic flexibility dictate life in the lower oceanic crust) (Puente-Sánchez et al., 2018. Viable cyanobacteria in the deep continental subsurface)
- So, martian life capable of photosynthesis is not impossible deep underground, but even then it would be using other metabolic pathways with no reason to live near the surface of a rock, in complete darkness.
Fourth, there is another possible exception, life can use thermal radiation from a deep sea hydrothermal vent for photosynthesis (Beatty et al., 2005. An obligately photosynthetic bacterial anaerobe from a deep-sea hydrothermal vent). The most recent possibility for a hydrothermal system in the southern uplands on Mars might be the rootless cones (volcanic cones without a magma chamber below them) which may have had hydrothermal systems above 0°C for up to 1,300 years ((Hamilton et al., 2010. Explosive lava ‐water interactions in Elysium Planitia, Mars: Geologic and thermodynamic constraints on the formation of the Tartarus Colles cone groups)) possibly active as recently as less than 20 million years ago (Stacey, 2019. Interactions between Athabasca Valles Flood Lavas and the Medusae Fossae Formation (Mars): Implications for Lava Emplacement Mechanisms and the Triggering of Steam Explosions).
However, these are very different conditions from a deep sea hydrothermal vent. For this exception we need:
- photosynthetic life using thermal radiation for photosynthesis could be found in the rootless cones in the last 20 million years
- be ejected to Earth,
- with the photosynthetic life only found near the surface of the ejected rocks,
That is what is needed for it to be sterilized in Earth’s atmosphere but potentially survive impact on Phobos. This may need expert review but on the face of it, it seems an unlikely scenario to the point of not realistic.
Then we don’t need to go further back than 20 million years, because any life from earlier impacts that might get into the surface samples from Phobos has had over 22.5% of many of its amino acids destroyed – this is calculated in the discussion of sterilization dose in:
- 500 million years of ionizing radiation would reduce a gram of amino acids to a milligram
So, the Phobos sample return analysis seems correct with this minor tweak to account for photosynthetic life.
The more eyes that look at these studies the better given how important it is to protect Earth’s biosphere.
If these arguments are correct, it may also be safe to send astronauts to Phobos so long as they sterilize any materials before contact from deep below the surface.
The issue for astronauts with deep subsurface samples is that there may be potential for viable life on Phobos buried deep after ancient larger impacts on Mars, which have been shielded ever since and are still viable, but can’t get to Earth currently, and perhaps potentially could have ejected photosynthetic life from the surface of Mars which got to Phobos but couldn’t survive the fireball of re-entry to Earth’s atmosphere.
The JAXA study found that the samples Japan plan to return from the surface of Phobos are biologically safe, but that's just the top few centimeters. Any life there has had a similar history of ionizing radiation to the Mars meteorites we receive from Mars today and has had an extra sterilization step of impact on Phobos which is if anything more sterilizing than the fireball of re-entry into Earth's atmosphere (SSB, 2019, Planetary protection classification of sample return missions from the martian moons).
ADDCITE / EXPAND
There is a remote possibility of viable life from Mars that survived the double sterilization processes of impact ejection form Mars, and impact into the surface of Phobos and penetrated deep into the surface of Phobos. The JAXA team's results only applied to surface layers. Any life that survived deep below the surface protected from ionizing radiation by the regolith could still viable though dormant (ADDCITE).
There might also be tracers of ancient life from Mars from hundreds of millions to billions of years ago. This of course also adds to the interest of Phobos. Astronauts can explore the moons of Phobos and Deimos for traces of past life.
A 2012 study found that a 100 gram sample could contain 0.2 micrograms in the 100 g sample deposited in the last 10 million years., Though a single large crater 60 km in diameter near the equator could send a similar amount of material to Phobos (Chappaz et al.,, 2013. Transfer of impact ejecta material from the surface of Mars to Phobos and Deimos) The team also say that a single 60 km diameter crater could send enough ejecta to Mars to equal their 0.2 micrograms.
[ADDCITE - check for more recent sources and the JAXA cite]
If there was life on Mars when a large meteorite hit Mars, it has not been exposed to chemical processes on the surface of Mars or alteration by water.
If some of it then got buried on Phobos, perhaps as a result of the impact of a nearby large meteorite on the surface there could be some potential for preserving material from early Mars on Phobos if it was reasonably abundant.
However for the older impacts it would need to be buried rapidly to a depth of several meters to protect the organics from ionizing radiation. We also have the risk of confusion with organic infall from space.See above:
Perhaps the rim of Stickney crater might have covered early organics deposited on Phobos just before the crater formed, and preserved it from the ionizing radiation?
Then if the past life had recognizable microfossils, those could survive the impact on Phobos enough to be still recognizable, for instance if it had analogues of the coccolithophores (NASA, n.d.,Marine Biota Exchange — The Biologic Pump). This book looks at the potential for fossils of microbial life on Mars below in the Swansong Gaia discussion:
Failure to detect life on Phobos doesn't mean that there was no life on Mars. But if life was abundant on Mars, in the past, then it might be possible to find it on Phobos, perhaps in some situations, more easily than on the surface of Mars itself, at least in the early stages of exploration.
However some of the more recent life might perhaps be viable if it has been buried deep below the surface for millions of years.
If this is still considered a possibility by then, the surface of Phobos around the base can be sterilized to a sufficient depth to make sure there is no life that could be accidentally released as a result of human activities. Similarly any parts of Phobos that could be exposed to human activities may need to be sterilized before humans access them and robotic moles used to explore deeper until it is better understood.
No concerns for Bennu or other Near Earth Objects because of natural contamination standard - and no other sample returns that might need protection until Ceres, Enceladus or Europa not likely until the 2040s
Text on graphic: The clean room is to protect the samples from Bennu from terrestrial organics.
There is no risk to Earth’s biosphere from samples from Bennu because of the natural contamination standard
We get a natural influx of several tons of unsterilized rocks similar to these every year.
Graphic from: NASA Solar System (@NASASolarSystem), 2023, The #OSIRISREx capsule is secure in the temporary clean room at the Utah Test and Training Range. Over the next few hours, the team will prepare the capsule for transport to @NASA_Johnson tomorrow. https://go.nasa.gov/46m1HNY, 6:28 PM · Sep 24, 2023. Tweet.
Experts have no concerns about sample returns from Bennu or other Near Earth Objects including Near Earth Comets. That's by Greenberg's natural contamination standard, the same reason as for Phobos sample returns” (Greenberg,et al., 2001. Macroscope: Infecting Other Worlds).
"As long as the probability of people infecting other planets with terrestrial microbes is substantially smaller than the probability that such contamination happens naturally, exploration activities would, in our view, be doing no harm. We call this concept the natural contamination standard."
(Greenberg,et al., 2001. Macroscope: Infecting Other Worlds)
We get tons of material from Near Earth Asteroids every year and much of it lands gently. Just the exterior heated up.
About 17,700 meteorites of 50 grams and larger fall every year.
QUOTE According to the research referred to above, there are approximately 17,000 a year.(Iberdrola, 2022, Meteorites on Earth: how many fall per year and why don't we see them?)
QUOTE Using the best-fit pairing estimate, we determined a global expected fall flux of 17,600 [12,200–25,400] yr-1 for masses >50
(Evatt, 2020, The spatial flux of Earth's meteorite falls found via Antarctic data)
We can also look at the fireballs. We get about 40 fireballs a year. Those would be for the larger meteorites (CNEOS, n.d., Fireballs)
We get over 1000 a year bright meteorites.(CNEOS, n.d., Bolides).
We get 5,200 tons from interplanetary dust every year though most of that would be so small it would be quickly sterilized by UV or ionizing radiation but some of that is dust from the breakup of fireballs (CNRS, 2021, More than 5,000 tons of extraterrestrial dust fall to Earth each year)
That doesn't work for Mars because any life on Mars has to be ejected into space through the atmosphere and with a sudden impact to eject it with enough speed to get into space against the martian gravity.
So, most life wouldn't be able to get into a meteorite to get to Earth.
But if there is life in asteroids (far harder place for life because any water or ice would immediately evaporate in the vacuum of space) then it already gets to Earth.
The main issue is for larger asteroids, the sample return from Ceres if they go ahead with that, would return samples around 2044, and it would return ice from Occata crater that couldn't get to Earth otherwise and could potentially have recently exposed ice from Ceres' mud ocean.
As for Bennu, it most likely is sterile, experts think small asteroids, even C and B-type asteroids have a very low chance of life, Bennu is B-type. But if it does have life, because of the natural influx it must be harmless. There's nothing special about Bennu there are many other asteroids like it and bits of them get into Earth's biosphere every year. So it is just replicating something that happens anyway.
So it has to be safe. This could be
- because they are all sterile, no life ever evolved or
- any life that evolved is harmless e.g. it may be very early life and easily extinguished in competition with terrestrial life.
Bennu counts as a "small body"
to the degree that sample return from missions to small bodies mimics even in a modest way the copious natural influx of interplanetary debris, it seems reasonable to assume that such samples are not potentially dangerous; this is the view that was adopted by the task group. For proposed missions to small solar system bodies that would return samples not introduced to Earth naturally, further analysis is needed before requirements for containment are considered.
(SSB, 1998, Evaluating the Biological Potential in Samples Returned from Planetary Satellites and Small Solar System Bodies: Framework for Decision Making : 21)
The chance of any life is extremely low, Bennu is B-type
Common asteroid types include undifferentiated, primitive types (C-, B-, and G-types); ... For undifferentiated, primitive (C-type) asteroids, the potential for a living entity to be contained in returned samples is extremely low, but the task group could not conclude that it is necessarily zero.
(SSB, 1998, Evaluating the Biological Potential in Samples Returned from Planetary Satellites and Small Solar System Bodies: Framework for Decision Making :3)
There may be hydrated minerals in the samples.
Data obtained from the spacecraft’s two spectrometers, the OSIRIS-REx Visible and Infrared Spectrometer (OVIRS) and the OSIRIS-REx Thermal Emission Spectrometer (OTES), reveal the presence of molecules that contain oxygen and hydrogen atoms bonded together, known as “hydroxyls.” The team suspects that these hydroxyl groups exist globally across the asteroid in water-bearing clay minerals, meaning that at some point, Bennu’s rocky material interacted with water. While Bennu itself is too small to have ever hosted liquid water, the finding does indicate that liquid water was present at some time on Bennu’s parent body, a much larger asteroid.
(NASA, 2018, NASA’s Newly Arrived OSIRIS-REx Spacecraft Discovers Water on Bennu)
It broke off a much larger body perhaps 700 million to 2 billion years ago:
Bennu likely was broken off from a much larger carbon-rich asteroid about 700 million to 2 billion years ago, which is relatively recent in geological time. It likely formed in the Main Asteroid Belt between Mars and Jupiter, and has drifted much closer to Earth since then.
Scientists think that a cataclysmic collision caused a carbon-rich asteroid 60 to 130 miles (100-200 kilometers) in diameter, roughly the size of Connecticut, to break apart, scattering pieces including Bennu. The asteroid has wandered into near-Earth space because of gravitational interactions with the giant planets and because of the long-term Yarkovsky effect – the small force on a spinning body due to its absorbing sunlight and re-emitting the heat as infrared radiation
(NASA, n.d., In Depth | 101955 Bennu – NASA Solar System Exploration)
From this description there seems to be no chance of liquid water on Bennu.
If so, the chance of life is likely totally zero because 700 million years is likely too long for a dormant microbe to remain viable even well below the surface and surely for surface samples.
It doesn’t actually matter if the risk is zero or extremely low because it is a NEO and we get a natural influx from asteroids like it anyway.
From the 1998 book again:
QUOTE Asteroids are the remnants of planetesimals—small primordial bodies from which the planets accumulated. Common asteroid types include undifferentiated, primitive types (C-, B-, and G-types); undifferentiated metamorphosed types (Q- and S-types [ordinary chondrites]); and differentiated types (M-, V-, J-, A-, S- [stony irons], and E-types). Other types of asteroids have been defined, including the common P- and D-types in the outer parts of the asteroid belt, but little is known about their composition and origin. Others are subdivisions of the types listed above, whereas still others are rare, new types, generally seen only among the population of very small asteroids.
(SSB, 1998, Evaluating the Biological Potential in Samples Returned from Planetary Satellites and Small Solar System Bodies: Framework for Decision Making :3)
I tried to find a planetary protection assessment for Bennu. But I don't find a detailed one like there was for previous sample returns.
I don't know if there is a new policy that they don't need that level of detail any more or if it exists and I haven't found it yet.
NASA's Planetary Protection Officer Nick Bernardini says: Bennu has zero chance of life.
“Due to this type of target body having only some of the key formational and evolutionary organic molecules necessary for life, there is no chance that the sample from Bennu could contain living organisms.”
(OSMA, 2023, OSIRIS REx Sample Return Doesn't Pose a Risk to Earth's Biosphere)
However he doesn't give any cites. So I don’t know the scientific basis of his reasoning.
This reasoning doesn’t seem very strong because we don’t know what are the required organics for evolution of life.
But it's not an issue for returns from NEOs because of the natural contamination standard.
If NASA return material from the asteroids from the outer edge of the asteroid belt with no natural influx to Earth that would need a planetary protection assessment for sure. The chance of life there is likely very low indeed but since we don't have a natural influx to Earth we'd need to consider the question more closely.
Then the larger asteroids need special attention. We have asteroids from Vesta which we can identify by their distinctive composition (American Museum of Natural History, n.d., Vesta Asteroid Fragments)
We probably have meteorites from Ceres but they would look like other carbonaceous chondrites (NASA, 2017, Dawn Discovers Evidence for Organic Material on Ceres – NASA Solar System Exploration)
Any mission to a larger asteroid that returns material better protected than it would be if knocked off the asteroid by a small asteroid impact, and sent on to Earth would need to be looked at carefully.
In the near future through to the 2030s, a Mars sample return seems the main concern. Also by the JAXA conclusions, if we return samples from deeper than 2 centimeters below the surface of Phobos we need a new planetary protection study for Phobos.
The satellite above GEO could later expand to a receiving station for samples throughout the solar system including Ceres, and eventually Europa and Enceladus
The new satellite would also be an investment for the future as it could be expanded to a receiving station for samples from everywhere in the solar system including the largest asteroid in the asteroid belt, Ceres, and Saturn’s moon Enceladus, both of which have potential for life, Enceladus could have life in its subsurface ocean, with plumes of water ejected into space (Neveu et al., 2020 . Returning samples from Enceladus for life detection) . Ceres could have life in a subsurface mud ocean because of evidence that it may still have internal heating and cryovolcanism (like a volcano but with liquid water instead of lava) and because of evidence of hydrated salt, hydrohalite (NaCl •2H2O) which must have been exposed recently (Castillo-Rogez et al., 2022, Ceres Exploration of Ceres' habitability)
However - if we do a mission to Ceres that samples an ice deposit, say, and returns it to Earth gently, protected from the vacuum of space, then that would be something not duplicated naturally. Given that Ceres is believed to have a mud ocean, there's a chance that recently exposed ice could have still viable life if life ever evolved in Ceres' mud ocean.
So that would need special care.
That's not for some time though, may return samples around 2044 according to the mission concept
QUOTE According to the concept a spacecraft would launch on a SpaceX Falcon Heavy rocket (or similar) in December 2030 and arrive at Ceres in July 2037. After 18 months in orbit for detailed reconnaissance it would send a lander to collect samples, “hop” to a second location elsewhere in Occator Crater to collect more samples, then launch back to Earth to arrive in 2044.
(Carter, 2022, Why this Ceres mission could change the search for alien life)
NASA may return samples from Ceres in the late 2030s with the mission already recommended in the Decadal review. This would return samples from the ice deposits in Occator crater which may also be one of the places where salty water from the subsurface muddy ocean is exposed to space. That's not for some time though, may return samples around 2044 according to the mission concept
According to the concept a spacecraft would launch on a SpaceX Falcon Heavy rocket (or similar) in December 2030 and arrive at Ceres in July 2037. After 18 months in orbit for detailed reconnaissance it would send a lander to collect samples, “hop” to a second location elsewhere in Occator Crater to collect more samples, then launch back to Earth to arrive in 2044 (Carter, 2022, Why this Ceres mission could change the search for alien life) (Castillo-Rogez et al., 2022, Ceres Exploration of Ceres' habitability).
Then perhaps further in the future, there are proposals to return samples from Enceladus captured by flying through the plumes. The return to Earth risks the samples getting further altered through reentry heating, landing shock and heating, and forward contamination from terrestrial life (Neveu et al., 2020. Returning samples from Enceladus for life detection).
From: (Neveu et al., 2020. Returning samples from Enceladus for life detection : Figure 4) ().
Neveu et al. comment
Organisms in Enceladus' ocean would be highly unlikely to survive ejection to space, sample capture, exposure to radiation during the back cruise, Earth reentry, and/or any exposure to the relatively oxidizing conditions of Earth's surface
(Neveu et al., 2020. Returning samples from Enceladus for life detection)
Return to a sample above GEO eliminates two of those four challenges, the Earth reentry, and the oxidizing conditions of Earth’s surface.
For returned samples they give four advantages over study in situ in orbit around Enceladus.
- More modern instruments (spacecraft can only have instruments available at the time of launch and usually selected long before launch)
- Return to above GEO also fulfils this
- Instruments that cannot be miniaturized
- Return to above GEO partially fulfils this – much larger instruments can be sent to above GEO
- Complex wet chemistry protocols or sample preparation steps
- May be some options for return to above GEO with artificial gravity and low latency telepresence
- A much more diverse suite of techniques than could be accommodated on any spacecraft.
- Return to above GEO also fulfils this.
The satellite above GEO meets two of those four challenges and partially fulfils the remaining two.
We can then apply the remaining techniques with pre-sterilized samples returned to Earth.
If the surface ice of Europa is dry, a Europa sample return might not need planetary protection since any surface ice samples would be sterilized already by the high levels of ionizing radiation.
However, there is some evidence suggests the chaos terrain in Thera Macula lies over a rising layer of liquid water heated from below (rather similar to the way that magma plumes form on Earth) (Schmidt et al., 2011 . Active formation of ‘chaos terrain’over shallow subsurface water on Europa). This liquid water can be shielded from thermal imaging by an insulating layer a few centimeters thick (Abramov et al., 2013 . Detectability of thermal signatures associated with active formation of ‘chaos terrain’on Europa).
Europa may also have water plumes as for Enceladus (Lesage at al., 2022 . Simulation of Freezing Cryomagma Reservoirs in Viscoelastic Ice Shells) which may bring water from the near surface to space. If so, they may have viable life in them as for Enceladus . NASA’s Europa Clipper will help resolve this question when it gets there in 2030 (NASA, 2022 , NASA Study Suggests Shallow Lakes in Europa's Icy Crust Could Erupt).
If Europa does have near surface liquid water, it could potentially have indigenous near surface life, as it would be partially shielded by the ice from the very high levels of surface ionizing radiation (NASA, 2011, Are Water Plumes Spraying From Europa? NASA's Europa Clipper Is on the Case).
If so, we may also need to protect Earth from samples from Europa’s near subsurface in the near future. These samples could be handled in similar ways to Ceres and Enceladus samples.
It would be easy to add simulation chambers that can be adjusted to any gravity and any temperature and light intensity. These could then handle samples returned from anywhere in the solar system.
Exploring Mars from orbit avoids the dust and risk of a Columbia type crash on Mars
[FIXCITES]
The methods used for robotic landing on Mars, of bouncing on expanding air bags, and skycranes, aren't easy to scale up to the multi-ton human spacecraft. Instead the main options considered are supersonic retropropulsion, or a very large parachute or ballute. Rob Manning gives an overview in his interview for the SpaceShow (podcast) (Manning, 2016, Broadcast 2673 Rob Manning : 14:00)
If the parachute is big enough, astronauts could do a conventional landing just as for Earth. The problem is deploying those parachutes and making sure they work. It's possible to work out some of the details with computer models, and test scale models of the parachutes in wind tunnels and so on. But at some point this work has to be tested with full scale parachutes in Mars analogue conditions. The parachutes used on Mars so far were tested by firing rockets in suborbital trajectories in Earth's upper atmosphere, because the Earth's upper atmosphere is similar in density to Mars. To make even larger supersonic parachutes requires a new set of these very expensive rocket tests. NASA did some work on this with their Low-Density Supersonic Decelerator (LDSD). (NASA, 2017, Low-Density Supersonic Decelerator (LDSD) Overview)
A large parachute might fail to open up, open up partially, open up at the wrong moment during the descent, or rip, amongst some of the failure modes.
NASA’s original plan was to use two very large parachutes to land humans on Mars. In their two tests in 2015, though the first parachute deployed successfully, Supersonic Inflatable Aerodynamic Decelerator (SIAD) deployed successfully and slowed it down from Mach 3.8 to Mach 2.5. However in both tests, the second chute, the Supersonic Disk Sail Parachute (SDSP) failed, in the second test it expanded most of the way but then tore apart before it was fully deployed (Koerner, 2018, The Supersonic Parachutes Carrying NASA's Martian Dreams). After this failure, the Human Architecture Team (HAT) decided by consensus at a supersonic parachute workshop that supersonic parachutes aren't applicable for payloads of over 10 tons such as are needed for human missions. (Bergin, 2015, LDSD test of Mars landing technology suffers chute failure). NASA was going to do a third test anyway, in 2016, but they cancelled it because of funding issues. So the second chute, which would be used to slow down to land on Mars remains untested (Koerner, 2018, The Supersonic Parachutes Carrying NASA's Martian Dreams).
The supersonic parachute technology was used for the Perseverance landing for the early stages before the skycrane which holds the record as the largest supersonic parachute used in a mission (Koerner, 2018, The Supersonic Parachutes Carrying NASA's Martian Dreams). But for larger payloads of ten tons or more,NASA seems to have decided on supersonic retropropulsion.
So this leaves supersonic retropropulsion which SpaceX uses successfully to return its rocket stages to Earth. The main issue with using the same approach on Mars is that the Martian atmosphere thins out rapidly and is only thick enough for this technique with landings that skim close to the surface in the lower altitudes of Mars. The atmospheric pressure also varies seasonally, between day and night and depending on the weather conditions at the time of the landing.
The main issue with supersonic retropropulsion on Mars is that the Martian atmosphere thins out rapidly and is only thick enough for this technique with landings that skim close to the surface in the lower altitudes of Mars. The atmospheric pressure also varies seasonally, between day and night and depending on the weather conditions at the time of the landing.
So the landing has to be flexible with the computer making decisions about what to do when depending on monitoring what happened and monitoring the atmosphere.
The most detail we have on this approach, not very detailed, is from Musk' 2017 talk on the topic SpaceX's plan avoids the need for a heat shield. The first stage is for the large ship to do the re-entry diving directly. Screenshots from: (Musk, 2017, Making Life Multiplanetary : 35:38)
In this approach the spacecraft first points towards the surface when it hits the atmosphere.
Then as it slows down it turns to tilt edge on to the atmosphere for maximum deceleration:
Once it is slow enough it turns to face away from the direction of travel for supersonic retropropulsion :
Then finally only a few hundred meters from the surface it gradually tilts back to a more vertical position for the landing.
The whole sequence takes 15 minutes, speeded up to a minute in the animation used by Elon Musk. The whole process is automated and there is much can go wrong here. With such a fast descent, humans couldn’t react fast enough to resolve the issues if something goes wrong during the descent, and couldn’t be relied on to make the right decisions at the right times to land safely.
Also unlike the case for the Apollo landings, the lander won't have enough fuel to return to orbit in an emergency, once it enters the Martian atmosphere it is committed to a landing. It needs to be refueled on the surface to return to Earth.
With this background we can see why Elon Musk said the first landings will be very dangerous.
"I think the first trips to Mars are going to be really, very dangerous. The risk of fatality will be high. There is just no way around it. It would basically be, 'Are you prepared to die?' Then if that's ok, then you are a candidate for going."
(Cooney, 2016, Elon Musk’s next great adventure: Colonizing Mars)
Their barge landings of the first stage used supersonic retropropulsion, in the very early stages when the first stage slowed down from 70 km down to 40 km, at just the right altitude to stand in for the tenuous Mars atmosphere. What’s more, they can achieve a pinpoint landing as well, as they have demonstrated several times - when it works. Perhaps that means pinpoint landings on Mars will be possible after all, once this technology matures. So it can certainly be done, but it is rather risky and tricky to do on Mars with the very thin atmosphere and its atmosphere far more variable in density than Earth’s (and full of dust in dust storms).
If the ship does crash, this spreads human remains, food, and other supplies over a large area of the surface of Mars, similarly to the Columbia disaster. That would likely lead to an accelerated start to the Martian anthropocene if there are any habitats on Mars that terrestrial microbes can inhabit.
(CAIB, 2003, Columbia Accident Investigation Board Report, Volume III, Appendix D.10, Debris recovery) - it spans about 250 miles (400 kilometers)
However astrobiologists generally seem agreed that if there are potential habitats for life on Mars, human missions to the surface are the start of the Martian anthropocene, when terrestrial life spreads on Mars irreversibly.
Astrobiologists debate how much they can do before humans irreversibly introduce terrestrial life to Mars
[Continues from earlier section: NASA's team seem unaware of the contemporary debate in astrobiology journals focused on how to ensure robotic spacecraft can study potential habitats before they are irreversibly contaminated by terrestrial life from human missions ]
The astrobiologist Alberto Fairén started this debate in 2017 with a paper published on 1st October (Fairén et al., 2017. Searching for life on Mars before it is too late). It has continued since then. Albertto Fairén and others advocate for doing as much astrobiology as possible with robotic landers and they advocate sending unsterilized robotic landers to sensitive regions on Mars. They know that this will mean they likely contaminate them with terrestrial biology but argue that robotic spacecraft will introduce less contamination than humans will and it is a chance to get some idea of what Mars was like before the anthropocene before it is too late to do such studies.
NASA's former planetary protection officers, Cassie Conley and John Rummel argue that we do have time to do astrobiology before humans get there. They also say that ethically we have to know it is safe to send humans to Mars before they go there, which means we have to do an astrobiological survey of Mars first. Their first reply was published simultaneously with Fairén et al's paper on 1st October 2017 (Rummel et al., 2017, Four Fallacies and an Oversight: Searching for Martian Life).
Both sides in this debate agree that after a human landing, we have introduced terrestrial life to Mars irreversibly. However the expert opinion of Alberto Fairén is it could occur relatively quickly after a human landing outpacing the ability of humans to explore the rest of Mars with rovers. He highlights the risk of extinction of native martian life after a human landing.
Text on graphic: Alberto Fairén - expert opinion at opposite end of the spectrum from NASA. Already certain of microbial oases for life throughout Mars globally.
What we already know is that the moment that astronauts set foot on Mars, microbial contamination will be inescapable and irreversible. Astronauts staying there for the long term will require some means of transporting and storing water and food, a continuous air supply, and the containment and management of secretions and human waste, among other requirements.
These activities create an unavoidable risk of microbial leaks from spacecraft, space suits, and waste disposal systems. The microbial leaks and species invasions could spread far enough to produce a global impact on Mars, eventually creating identifiable sediments.
… risk of extinction of any extant microbiota on Mars
…
Today’s reality is that our children or grandchildren will see astronaut footprints on the red sands of Mars. And when that happens, the Mars Anthropocene will begin.(Fairén, 2019, The Mars Anthropocene)
Those who argue for dropping sterilization reason that humans are likely to get to Mars in the near future. They argue that humans won't wait for the astrobiological survey to be complete. They agree that the science we do today with unsterilized rovers would be confused by terrestrial contamination carried by the rovers in a way that it would not be with sterilized rovers, that there is a good chance that we can disentangle the signal of native martian life from terrestrial life so long as we can get there with the rovers before the much more extensive terrestrial contamination brought by human astronauts reaches the site of interest.
Those that say we need to take care say we have time to do good science unconfused by terrestrial contamination before humans get to Mars. They argue that we have legal and ethical reasons that will ensure that we are not going to send humans to the surface until we know enough about surface conditions to know that humans won't encounter martian life and know that the missions will be safe for the astronauts and Earth's biosphere. They say that the extra funding for better sterilized rovers is significant, and adequate planetary protection might be 10% of the cost of a mission, but that this is an amount that can be found if it is prioritized as an ethical requirement. This book discusses the topic with a suggested solution below:
One of the main controversies here is about whether and to what extent it would be possible to distinguish terrestrial contamination brought by the rovers from native life on Mars, and whether even the low levels of initial contamination brought by unsterilized rovers could overwhelm martian life before we discover it. How quickly would life spread to the habitats from dirty rovers?
Both sides agree that sterilized robots are better. The differences in view are pragmatic, based on considerations of cost. The reason anyone recommends unsterilized rovers is to reduce cost and so increase the potential for robotic missions to be approved in time to do useful science before humans get there.
For the special regions astrobiologist want to study, with a high potential for habitats for present day life, the sterilization to Viking standard would add 10% to the cost. This may seem at first to be a small amount to be quibbling over with such high stakes, however it is a significant sum in a multi-billion dollar mission. With their natural focus on reducing costs as a top priority, NASA has to reduce costs as much as possible and so is not likely to approve a mission if it costs 10% extra. Instead they will wish to modify the remit of the mission to explore a region of Mars that doesn't require this extra level of sterilization.
As an example of how in NASA costs drive the mission planning, the planetary protection officers wanted Viking level sterilization for Perseverance, because it is a life detection mission. The aim for a life detection mission is to reduce the risk for forward contamination to confuse the results even if there isn't a risk of forward contamination. However, the engineers, administrators and so on at NASA have been given other priorities. They have to focus on reducing cost, and wherever possible, removing remove anything that might introduce potential extra failure points.
As we saw NASA paid less and less attention to planetary protection demands for a cleaner mission, and NASA eventually responded by closing down the planetary protection office. See:
We can understand from this why, given the choice between a 10% more expensive mission with a focus on present day life to a sensitive region and a lower cost mission with a focus on past life and geology which doesn't need this sterilization, NASA are going to adopt the past life and geology focused mission every time.
NASA is required to reduce costs of missions as a high priority. NASA divides its technologies into three categories, Critical, enhancing and transformational. 70% of the funding goes to critical technologies, 20% to enhancing and 10% to transformational technologies (NASA, 2017 .NASA Strategic Technology Investment Plan : 6). Planetary protection for robotic missions is not seen as critical but only enhancing for future human missions (NASA, 2017 .NASA Strategic Technology Investment Plan : 36).
This book argues that planetary protection for robotic missions should be seen as Critical Mid-Term for both robotic and human missions for as long as the mid-Term goal is to send humans to the martian surface and even if the mid-term goal is Phobos it is still of central importance to such a mission too, so should be seen as Critical Mid Term. See below:
This is why Fairén et al. advocate for immediately dropping planetary protection protocols for Mars to do rapid study of astrobiology in sensitive regions. Here "special regions" are regions where terrestrial life may be able to grow on Mars.: They ask for robotic missions to go to the special regions in the next 10 to 20 years before humans get to Mars as a matter of urgency for astrobiology. They believe it would be possible to use genetic sequencing to distinguish the contamination introduced by their unsterilized robots from any native martian life.
We advocate for allowing immediate access to the Special Regions for vehicles with the cleanliness level of Curiosity, Mars2020 or ExoMars. Special Regions could hold a sluggish extant biosphere able to produce biomarkers even under current Martian radiation, because viable microorganisms would repair cellular damage resulting from ionizing radiation; on the contrary, biomarkers of extinct life would simply degrade in several hundred millions of years in the top meter of Martian surface due to exposure to cosmic rays (Pavlov et al., 2012) and the oxidizing surface chemistry (Mancinelli, 2017). Therefore, focusing on the detection of evidence for extant life in subsurface and surface rocks and regolith in Special Regions may be more realistic than the hopes of detecting ancient and highly degraded organic biomarkers at or near the Martian surface on the long timescale.
… We urgently need to designate, describe and analyze a few special places on Mars that we can get to now (i.e., in the next 10–20 years) with rovers and landers, and try to do a better astrobiological job asking and addressing questions regarding whether there is present-day near-surface life on Mars or not, before the arrival of manned missions.(Fairén et al, 2019, Planetary Protection and the astrobiological exploration of Mars: Proactive steps in moving forward)
NASA's first two planetary protection officers, John Rummel and Cassie Conley agree we need to do a dedicated robotic search for life on Mars before humans get there.
We do genuinely agree with the position of Fairén et al. ‘‘that we need to resume a dedicated robotic search for life on Mars as soon as possible, before manned missions reach the planet and it becomes too late.’’
(Rummel et al., 2018, Inadvertently Finding Earth Contamination on Mars Should Not Be a Priority for Anyone : 113)
However, they are of the view that we still have time to do careful exploration and are not convinced that DNA sequencing can reliably distinguish native Martian life from terrestrial life and are also concerned that microbes introduced by dirty robots can contaminate resources that humans might want to use later on.
We find that there are shortcomings in their plans to look for evidence of life on Mars, that they do not support their contention that appropriate levels of spacecraft cleanliness are unaffordable, that there are major risks in assuming martian life could be identified by nucleic acid sequence comparison (especially if those sequences are obtained from a Special Region contaminated with Earth life), and that the authors do not justify their contention that exploration with dirty robots, now, is preferable to the possibility that later contamination will be spread by human exploration. We also note that the potential effects of contaminating resources and environments essential to future human occupants of Mars are both significant and not addressed by Fairén et al.
(Rummel et al., 2018, Inadvertently Finding Earth Contamination on Mars Should Not Be a Priority for Anyone : 114)
They don't share the vision of a near term global anthropocene, they are of the view that some degree of forward contamination from a human mission is inevitable but it can be minimized by operational and technological precautions.
Human missions, by design, will keep associated microbes alive as long as habitats are powered, and many will reproduce in great profusion. The humans, however, will need to contain and constrain microbial growth and reproduction, both to ensure proper operation of life support systems and because the humans and the microbes will be competing for water. It is true that "some degree of forward contamination associated with human astronaut explorers is inevitable" (Conley and Rummel, 2010), but for human exploration missions, the spread of those microbes into Mars Special Regions can be minimized, if not avoided completely, by appropriate operational and technological precautions.
(Rummel et al., 2018, Inadvertently Finding Earth Contamination on Mars Should Not Be a Priority for Anyone : 114)
The 2010 paper they refer to says that localized forward contamination of the regions around the landing site and human operations is inevitable but that spacesuits and and habitats would be designed and operated in such a way as to minimize it. [They don't go into details, but for instance, human waste wouldn't just be left on the surface as it was for the Apollo mission but would need to be disposed of internally as for the ISS]].
They say precautions must be taken to make sure it can't spread to special regions after a human landing or if humans crash on Mars.
The overall goal of planetary protection measures for human missions will be to prevent the contamination of "Special regions" on Mars (as defined by COSPAR policy), which are areas where Earth organisms might grow and thrive, or which might support indigenous martian life
... Prior planning will need to ... anticipate off nominal mission operations, as well, and avoid contamination of Special Regions even in the event of an accident
(Conley et al., 2010, Planetary protection for human exploration of Mars)
Accidents there would include a fire in the habitat forcing the astronauts to exit it without taking control measures to protect the surface from forward contamination, damage to a waste containment system, a rip in an EVA suit, or a spaceship with astronauts on it crashing on Mars. There would need to be measures in place to respond. They don't go into details, but presumably follow up missions to reverse the contamination. Human astronauts would also need precautions to prevent backwards contamination from Special Regions (which they might explore robotically from the landing site).
Rummel et al identified funding as the fundamental issue preventing adequate planetary protection to access the special regions in the forwards direction during the current phase of exploration of Mars. This will need to be addressed. They don't go into details but others have since then. This book covers issues of funding for planetary protection for robotic missions, and one proposal for how they could be resolved below, starting at:
Rummel et al. don't see planetary protection cleanliness as to blame for the delay in sending life-detection missions to Mars.
We dispute, however, their contention that planetary protection cleanliness requirements are somehow to blame for the delay in sending life-detection missions to Mars. We also disagree with the proposal that cleanliness requirements should be relaxed on near-term robotic missions—rather, improvements in our understanding of environments on Mars and the capabilities of Earth organisms highlight the continued need to ensure that planetary protection precautions are effective.
(Rummel et al., 2018, Inadvertently Finding Earth Contamination on Mars Should Not Be a Priority for Anyone : 114)
They drew attention to the knowledge gaps that need to be filled. They say it would be ethically incorrect to send a human mission to Mars without first understanding the potential risks to people on Mars of potentially exposing them to martian living organisms.
The short paper by Ehlmann et al. (2017) noted the need for ‘‘evidence that extant life is not widespread in martian surface materials’’ and the opportunity for the ‘‘continued characterization of martian surface materials to determine whether extant life is present’’ as important opportunities to address critical knowledge gaps about Mars. We contend that it would be ethically incorrect to send a human mission without understanding the resultant potential for exposing people (tourists?) to martian materials that may contain living organisms. Regulatory frameworks for licensing space missions exist, and treaty obligations apply even to countries that may not have implemented the appropriate national laws. It would be surprising if human missions to Mars were authorized to launch, prior to consideration of these sorts of concerns.
(Rummel et al., 2018, Inadvertently Finding Earth Contamination on Mars Should Not Be a Priority for Anyone : 114)
So they reject the proposal to send dirty rovers to Mars to cut costs. But they are of the expert opinion that Mars life-detection is essential to the health and safety of human missions and should be a priority. They encourage discussion of this in the Mars community and the broader community of the citizens who pay for the efforts and could be affected by the missions.
For multiple reasons, we reject the proposal by Fairén et al. that we should cut corners and attempt to fly robotic missions that could only equivocally resolve questions about martian life, before humans arrive on the planet.
Nonetheless, we believe that a Mars life-detection mission is essential to the health and safety of future humans on Mars and that this effort needs to be conducted in the most effective possible way, without sacrificing its essential credibility in the name of expediency. We strongly encourage continued discussion of these issues, both within the Mars exploration community and also in an expanded global community of citizens who both pay for and could be affected by such efforts.
(Rummel et al., 2018, Inadvertently Finding Earth Contamination on Mars Should Not Be a Priority for Anyone : 114)
The paper by Ehlmann et al that Rummel et al refer to identifies "evidence that extant life is not widespread " as the fourth of four knowledge gaps that need to be resolved in a way favourable for human safety before we can have human exploration of Mars.
Human exploration of Mars requires deeper understanding about the planet’s physical environment in addition to new technical capabilities. For instance, key knowledge gaps for both NASA- sponsored and commercial human exploration include
...d) evidence that extant life is not widespread in martian surface materials.
...
Critically, robotic sample return could facilitate uncontaminated return of samples from Mars special regions, where the chance for extant life is highest
(Ehlmann et al., 2017, Mars Exploration Science in 2050)
The COSPAR study of 2023 identifies a list of knowledge gaps that need to be filled before a human mission to Mars, with one of the most important, the gaps related to natural transport of viable terrestrial life on Mars, the same knowledge gap that SR-SAG2 singled out as of utmost importance.
Natural Transport of Contamination on Mars
3A. Measurements/models needed to determine atmospheric transport of contaminants
3B. Measurements/models for subsurface transport of contaminants
3C. Effect of biocidal factors on survival factors on growth and adaptation of microorganisms
3D. Determination of acceptable contamination rates & thresholds
3E. Protection mechanisms for organisms on Mars
3F. Degradation of landed materials by martian environment
3G. Induced environmental conditions around structures
3H. Sensitivity of non-culturable species to biocidal factors(COSPAR et al., 2023, Final Report of the COSPAR Meeting Series on Knowledge Gaps in Planetary Protection for Crewed Missions to Mars : III)
This is the same knowledge gap that SR-SAG2 singled out as especially worthy of consideration as if viable life can be transported in the dust “ the designation of Special Regions becomes more difficult, or even irrelevant.” (SSB, 2015. Review of the MEPAG report on Mars special regions :12)
To resolve this Ehlmann et al. set out a timetable of multiple small missions to Mars in the 2020s to do this mapping and astrobiological investigation leading to a sample return in the 2030s (Ehlmann et al., 2017, Mars Exploration Science in 2050) , however instead NASA has focused on a single large mission to return samples from one location on Mars which is not going to be able to resolve this question.
The 2020s could focus on an orbital mapping effort for localized exposures of current and past volatiles (and resources) as well as astrobiological investigations for extant life, which should be pursued vigorously before humans begin in situ exploration. This could be accomplished by a mid-size orbiter and multiple small, MER-class rovers with next generation instrumentation to meet science needs. The focus in the late-2020s and into the 2030s could shift toward return and analysis of samples from Mars, perhaps facilitated by humans on a Mars flyby mission, and emplacement of the weather/comm small sat. network.
(Ehlmann et al., 2017.Mars Exploration Science in 2050)
This also is the suggestion of this book. However based on modern engineering of the last few years, not available even in 2017, the suggestion is that we can have 100% sterile landers and because of miniaturization, many more of them. Also as we've seen, to have humans on Phobos rather than the surface as the intermediate goal, with the next steps depending on whether or not we discover it is biologically safe for humans to land on the surface of Mars.
2021 COSPAR recommendation to relax planetary protection if terrestrial life would only spread locally, but with buffer zones of hundreds of kilometers around cave entrances impossible to detect from orbit
COSPAR hasn't changed the planetary protection requirements for Special Regions, there is no prospect at present of "dirty rovers" with less than Viking level sterilization.
However the Council on Planetary Protection ruled in 2021 that planetary protection requirements CAN be reduced for missions to the surface based only on the presence or absence of nearby caves or ice if the contamination will be local to the region even if there are microhabitats for life in the soil. As summarized by Olsson-Francis et al:
Based on the sterilisation conditions at the surface of Mars, the CoPP focused on bioburden relaxation relating to potential access to sub-surface environments. It concluded that the bioburden requirements could be relaxed if the following criteria were met:
1a) Mission activities are restricted to the surface or
1b) For mission activities as deep as 1 m, the landing site must not show signs of ice in neutron or thermal data.
(Olsson-Francis et al., 2023.The COSPAR Planetary Protection Policy for robotic missions to Mars: A review of current scientific knowledge and future perspective : 32)
The recommendation of the CoPP in that study is that even if terrestrial life can spread locally on Mars the guidelines can be relaxed, so long as the habitat or microhabitat it colonizes is sufficiently biologically isolated from other potential habitats on Mars.
Regardless of the uncertainties, some of these environments are likely to have limited connectivity, and thus some contamination and growth could potentially be acceptable because it would be contained and thus would not constitute harmful contamination. Replication may be acceptable within a contained region, as long as proliferation outside that region does not occur:
...
To summarize the points discussed above, environments that permit microbial growth could be present in the top tens of centimeters of the shallow subsurface and below ~tens of meters in the deeper subsurface. However, such environments are likely discontinuous in
(1) the upper few (~5-20) centimeters of the surface anywhere, and
(2) the upper meter, in regions where no ice is detected from neutron or thermal observations.
As such, these regions could be suitable for relaxed bioburden requirements.
(Committee on Planetary Protection, 2021.Evaluation of Bioburden Requirements for Mars Missions :33 - 34)
They did however warn that contamination shouldn't be permitted if there are nearby cave entrances.
Finding 4: Microbial transport and proliferation are highly unlikely in disconnected subsurface environments. Thus, relaxed bioburden requirements could be appropriate for missions that do not access the subsurface, or for missions that access the subsurface (down to ~1 m12) where no evidence of ice exists.
Exceptions to this finding include buffer zones around subsurface access points and sites of astrobiological interest.
(Committee on Planetary Protection, 2021.Evaluation of Bioburden Requirements for Mars Missions : 34)
Their illustrative calculation for a buffer zone is 600 kilometers assuming a wind speed of 10 km / second.
At this velocity a suspended particle could travel approximately 540 km in 15 hours. Adding margin for higher wind speeds, the committee adopts a buffer radius of 600 km. This is considered a conservative value because it assumes unidirectional transport and does not include dust settling time. Landing during atmospheric conditions that promote higher wind speeds (e.g., dust storms) would necessitate a larger buffer zone; the committee’s calculation here assumes normal conditions.
(Committee on Planetary Protection, 2021.Evaluation of Bioburden Requirements for Mars Missions : 37)
[section starts: (Committee on Planetary Protection, 2021.Evaluation of Bioburden Requirements for Mars Missions : 36)
Many types of caves such as fracture and erosion caves are impossible to detect from orbit so this means that it's not possible to know if a site is suitable for these rules without landing first.
It would be a very major exercise involving dozens of surface missions to map out all fracture and erosion caves in a radius of 600 kilometers. Also there are occasional land slips which could expose caves that weren't previously detected.
Based on this calculation, if any caves are found the 600 kilometer diameter region around that cave needs to be excluded. For details about the potential for habitable caves on Mars and the difficulty of detecting them from orbit or even from the ground, see the section:
This seems a criterion that is of more theoretical than practical interest unless terrestrial life can be limited to spread considerably less than 600 kilometers as we find out more about the surface conditions on Mars.
Also - if a buffer zone of 600 kilometers is needed around every cave entrance, wouldn't a similar buffer zone be needed around any microhabitat in the top few centimeters of the sand dunes? Curiosity noticed a sudden drop in the water volume mixing ratio whenever it traveled over sand dunes which the team interpreted as evidence of water exchanging with the soil (Martín-Torres et al., 2015. Transient liquid water and water activity at Gale crater on Mars : fig 2b).
Earlier in this book we looked at how large sand dunes on Mars may seem static. However many of them are actively moving in the winds. Dunes ranging from a few meters to hundreds of meters in height are moving constantly. The average rate is about half a meter a year but the faster dunes move over 3 meters a year. This means the entire dune shifts, preserving its shape, about half a meter every year.(Chojnacki et al., 2019. Boundary condition controls on the high-sand-flux regions of Mars : Figure 1).
Over timescales of decades to centuries, these dunes move hundreds of meters. They are rich in perchlorate salts (which can form the brines Curiosity found, and also useful for life as an oxidant), nitrates (as a source for nitrogen, essential to life as we know it), are basaltic in composition and so have all the other trace elements life needs, and also have other chemical compounds of interest to life like oxygen, carbon monoxide and hydrogen (Stern, et al., 2018. Major Volatiles Evolved From Eolian Materials in Gale Crater : table 1), and they churn the surface and return material to the surface. If there are microhabitats anywhere in the dunes, this provides chemical redox gradients which life can use as a source of energy, for instance surface layers of sand dunes are superoxygenated while the slowly moving sand dunes constantly bring subsurface reducing layers to the surface (Fisk, et al., 2013. Habitability of Transgressing Mars Dunes).
The winds lift grains up to half a millimetre in diameter, and Curiosity observed grains as large as 3 mm in diameter moving on the surface of Mars in an active dune field called Bagnold sands (Vasaveda, 2020. Mission Overview and Scientific Contributions from the Mars Science Laboratory Curiosity Rover After Eight Years of Surface Operations : 4.2). We looked at how if there are microhabitats in any of these dunes, grains potentially carrying viable life from these microhabitats up to half a millimeter in diameter could be set bouncing and travel for hundreds of kilometers in the light martian winds.
For details of how this works see above:
There are many other issues with the study.
Olsson-Francis et al say there is a need to incorporate the latest scientific data into the COSPAR guidelines which requires broader community expert input and concensus. Amongst many other topics Olsson-Francis et al. discuss the subsurface metastable brines that Curiosity found and other possibilities including microbes able to extract water from salts such as gypsum, and conclude that the potential for habitability cannot be ruled out.
Although the conditions of these metastable brines have been deemed inhospitable to life (Rivera-Valentin et al., 2020), the potential for these brines to be habitable cannot be ruled out, as discussed previously (Carrier, 2020). On Earth, brines impact growth due to low water activity, high ionic strength, extreme chaotropicity, and/or multiple extremes (Hallsworth, 2021 and references within). Although this may hinder growth, there is no evidence that the conditions would kill/eradicate microbial life in certain brines, e.g., sulfate salts. Experimental work has recently shown that cells of Halomonas sp. str. HL12 survived and grew after several cycles of desiccation (Cesur et al., 2022).
This is prudent, as our understanding of water distribution on Mars is evolving rapidly. For example, new research about the upper meter of the regolith, based on the latest observations from the ExoMars Trace Gas Orbiter (TGO), has shown the existence of large patches of up to 40.3 weight % water equivalent hydrogen in the central part of the equatorial canyon Valles Marineris (Mitrofanov et al., 2022). This demonstrates the existence of vast deposits of ice or hydrated salts near the equator within the upper meter of the regolith that has remained hidden until now.
(Olsson-Francis et al., 2023.The COSPAR Planetary Protection Policy for robotic missions to Mars: A review of current scientific knowledge and future perspective : 32)
For details see:
Olsson et al. conclude that there are limitations to the approach of in-situ bioburden control recommended by COSPAR and suggest any future COSPAR guidelines would benefit from broader community expert input and consensus
In-situ bioburden control was considered as an alternative approach to pre-launch bioburden reduction methods. As mentioned in the paper, although this could be a more practical approach, there are also limitations to this approach, e.g., hidden surfaces, and verification of reduction. The report also outlined a flexible risk management approach that considers growing scientific knowledge and the specific type of mission to address the objectives of planetary protection, for example, taking into consideration the potential presence of extremophiles, the impact of post-landing bioburden reduction opportunities and the requirements of the specific mission that can impact the risk of probable harmful contamination. This approach would allow for the utilisation of existing and new models, tools and methodologies; and incorporate the latest scientific data. However, to date, these suggestions have not been incorporated into the COSPAR guidelines, and would require broader community expert input and concensus.
(Olsson-Francis et al., 2023.The COSPAR Planetary Protection Policy for robotic missions to Mars: A review of current scientific knowledge and future perspective : 30)
Suggestion to resolve this debate with more, rather than less ambition, making a case to Congress for extra funding for 100% sterile rovers, integrated closely with human missions with the aim to find out if it is safe for humans to land on Mars
Planetary protection is currently seen as relatively low priority in the technology needed for robotic and human missions. NASA is required to reduce costs of missions as a high priority.
NASA divides its technologies into three categories, Critical, enhancing and transformational. 70% of the funding goes to critical technologies, 20% to enhancing and 10% to transformational technologies (NASA, 2017 .NASA Strategic Technology Investment Plan : 6). Planetary protection for robotic missions is not seen as critical but only enhancing for future human missions (NASA, 2017 .NASA Strategic Technology Investment Plan : 36).
This is just a selection of the many technology categories and classifications (NASA, 2017 .NASA Strategic Technology Investment Plan : 36) :
Many of those technologies are seen as Critical. However, planetary protection at present is seen only as Enhancing. Only 20% of NASA’s technology development funding goes into Enhancing technologies.
The STIP advocates a 70/20/10 balance and targets investment levels of 70 percent for Critical, 20 percent for Enhancing, and 10 percent for TransformationaL
(NASA, 2017 .NASA Strategic Technology Investment Plan : 7) :
Enhancing technologies will comprise approximately 20 percent of the Agency’s technology development portfolio. Investments in Enhancing technologies are integral to supporting the strategy outlined by the STIP. Enhancing technologies represent enhancements to existing technologies or capabilities and offer increased performance, safety, reliability, which in turn can reduce system risk.
(NASA, 2017 .NASA Strategic Technology Investment Plan : 29) :
By the reasoning of this paper, investment in 100% planetary protection for unmanned rovers should be seen as a "Critical Mid Term" investment for human missions. This is because humans can't know whether or not it is safe to land on Mars without an astrobiological survey to find out what is there.
Also if we find humans can land on Mars or if we find that it is impossible to ever land on Mars we have two very different futures. If we do land on Mars, correct planetary protection may well remain critical mid-term for those missions, for instance protecting water supplies from biofouling or conversion to calcite.
So far the only solutions explored in the literature to the problem of how to find out what is there on Mars before humans get there are to keep the current planetary protection guidelines or to relax them. But perhaps with the improvements of modern technology this is an appropriate moment to consider a completely different approach, to enhance planetary protection to get the results of an astrobiological survey faster and in a far more reliable way.
The background to all the discussions so far has been the underlying assumption, going back to Sagan, that we can't reduce the risk of forwards contamination to zero. Even with robotic missions, after hopefully a very large number of missions, we would introduce terrestrial life to Mars.
This is an example paper from 2019. Lopez et al. who hypothesize that it is near impossible to explore new planets without introducing microbial travelers.
Based on modern microbiology, we propose a major revision in current space exploration philosophy and planetary protection policy, especially regarding microorganisms in space. Mainly, microbial introduction should not be considered accidental but inevitable. We hypothesize the near impossibility of exploring new planets without carrying and/or delivering any microbial travelers.
(Lopez et al., 2019, Inevitable future: space colonization beyond Earth with microbes first)
However the situation has changed quite rapidly even in the last four years since that paper. As we saw, modern high temperature electronics and the Venus HotTech now gives us this capability that we didn't have at the time of Sagan or indeed even as recently as 2019, of 100% sterile landers on Mars. See above:
So we have new options now we didn't have four years ago and perhaps this debate can be revisited. The martian anthropocene would be an irreversible change not just for us but for all future generations.
So this book is suggesting a third way here, a way that doesn't contaminate Mars at all until we know what is there. Because this can potentially add to cost and complexity, this is not a path that NASA is likely to adopt without a major change of direction.
However this book is suggesting that this is a debate we need to go to the public with rather than keep confined to the academic literature.
This book argues that this is a decision for our civilization as a whole. It is not a decision just for enthusiasts who wish to attempt to colonize Mars as quickly as possible and engineers who wish to reduce costs and complexity as top priority.
It also argues that the implications of human colonization before we know what's there on Mars should be explained to the public and discussed in a full and open way, that we risk introducing life to Mars irreversibly and that there is a risk of extinction of any extant martian life on Mars. It suggests that this debate should be opened out to input to those who have other points of view on the matter. We need to actively promote discussion and understanding, so we can debate it thoroughly and make any decisions with full understanding of what we are doing.
It is a decision we need to make as a civilization and with our eyes open, aware of what we are doing. Do we want to start an anthropocene on Mars before we know what is there? Or do we want to find out what is on Mars first and then decide if we want to start an anthropocene on Mars? Are we prepared to perhaps spend a little more on robotic exploration initially and add a little to the complexity of the missions in order to do this?
By combining this with the need to find out if it is safe for humans to land on Mars, with a rapid survey from orbit, this book suggests we can make the robotic exploration an essential part of the human exploration of space. Then we no longer have this polarization between the robotic and the human missions, and both would be working together. Those who support astronauts and those who support human colonization would want to know what is on Mars too.
With this perspective, the robotic searches become integrated more with the human exploration, to be controlled by humans in orbit around Mars. Instead of adding 10% from the very tight robotic exploration budget to partially sterilize the robotic spacecraft to Viking standards, we can have a fully funded program to develop 100% sterile landers which should attract more funding as they would then be seen as part of the human exploration program which attracts far higher levels of funding than robotic exploration.
The costs are high priority for human missions too, but 10% of the funding of a robotic mission is only a minute fraction of the funding for human exploration for the astronauts that would be using those robots for remote exploration once they get to Mars orbit and Phobos, and also for robotic missions that we do now, if they are seen as an integral part of human exploration.
The motivation for taking such care, even if it costs a little more, is covered in more detail in the Supplementary information section under:
In the backwards direction, public concern about large-scale effects is the likely driver for a change of policy. Also, we will find that the alternative of a robotic lab above GEO may be a way to achieve this even with a reduction in the total cost of the mission. See below:
- A small step for NASA, a giant leap for planetary protection: the case for a miniature telerobotic lab above GEO
- How a miniature life detection lab above GEO can overcome the technological uncertainties of a fully telerobotic biosafety lab on Earth, at significantly lower cost, with a significantly higher technological readiness level and more robust biocontainment
But in the forwards direction, it seems inevitable that planetary protection will require a little more funding. This means change can only happen with a major change of NASA funding policies. As we saw, one way to achieve it would be with a dedicated budget for planetary protection added to the estimated mission costs for unsterilized missions.
The robotic missions budget and the time constraints for the engineering for the missions are so tight for robotic missions we can't expect the NASA planners and engineers to adopt a change that increases cost and complexity from their side without direct support by way of extra funding.
In the USA this is likely a matter for Congress as we saw in:
- Need to avoid a trade-off between planetary protection and NASA's obligations to minimize costs
- An independent body to safeguard Earth and Mars: avoiding perverse incentives to reduce safety by ensuring planetary protection costs don't come from NASA's budget
It may be easier to get the support needed for extra funding to achieve 100% sterile missions if
- this is seen as essential for the human missions to Mars, both the colonization enthusiasts and the astrobiologists present the same case,
- it is also seen as an investment for the whole of our civilization to protect potentially valuable assets on Mars (the insights and potentially also commercial value of a second genesis on Mars)
The extra cost for sterilization per mission may well go down considerably once the designs and methods for 100% sterile landers are well established. It is mainly a matter of specifications for a 100% sterile lander, and then some way of heating it to 300°C after it leaves Earth, or else using a shroud of some sort later discarded. Once we now how to do it with one mission it wouldn't add so much mission cost after that and especially if we are also using far smaller landers by then as we saw in:
Interesting astrobiology but numerous worst case planetary protection issues both ways for the scenario of distant cousins of terrestrial life on Mars
We've already seen that even with related life, Mars could have soil pathogens that no terrestrial organism has ever encountered, leading potentially to major issues in the worst case. It could be the same in the other direction too, that terrestrial fungal pathogens of microbes such as the Chytrid fungi might have never got to Mars, and if so could have a devastating effect on the microbial populations there, and change how the microbial ecosystems function.
A scenario of related life could also be of great interest for panspermia. We'd find out by observation which species, genera, families, domains were able to spread between the two planets. We'd also be able to look at how particular genera, families, even domains evolved in the radically different conditions on Mars.
There are many possible scenarios here of great interest but also with potential for significant impacts on the two biospheres if they are permitted to mix in the worst case scenarios.
Here are some of them based on ideas explored in this book:
- never developed photosynthesis or has more efficient photosynthesis
- never developed nitrogen fixation or novel more efficient nitrogen fixation
- novel genus of fungal pathogens of humans or other terrestrial organisms on Mars, or novel fungal genus of microbes on Earth that has never reached Mars (e.g. the Chytrids)
- novel accidental toxins, metabolites, etc.
- novel capabilities developed on Mars such as very high levels of resistance to ionizing radiation, UV, or molds with capabilities to grow in freezers or to grow far faster in fridges, or to spoil cheeses, milk, or cakes
- Earth has a novel entire domain of life (e.g. eukaryotes) that never arose on Mars or Mars has an entire domain or multiple domains as deeply branched as the archaea that never got to Earth
- normal terrestrial life could co-exist with novel biology such as mirror life on Mars
[Link to sections about those topics in the book]
How introducing microbes in the wrong order in an accidental anthropocene could harm human prospects on Mars
There are many ways that microbes could be useful to Mars colonists. Charles Cockell lists: (Cockell, 2010, Geomicrobiology beyond Earth: microbe-mineral interactions in space exploration and settlement)
- Microbial fuel cells to generate electricity
- If introduced microbes can grow on Mars they might be able to bind the dust and stop the dust storms and air could also be passed over racks of biocrusts in a station as a form of filter.
- Releasing nutrients from the regolith
- Soil formation for greenhouses
- Extracting metals and other useful elements from the regolith.
However, introduced microbes could have unexpected effects. For instance they could turn underground aquifers into cement.
For example, introduced Earth microbes could prevent the use of existing resources on, or under, the surface of Mars. We don't have a good understanding of where, and in what form, those resources will be discovered; but if something like an underground aquifer is contaminated by Earth organisms, it is clear that it could quickly be made less useful by the Earth organisms released into it. In particular, certain Earth organisms introduced into an underground environment could behave like the self-calcifying crack-closing microbes discovered, and used as a concrete additive, by some clever researchers at the Delft University of Technology
(Rummel et al., 2017, Four Fallacies and an Oversight: Searching for Martian Life)
The cite is (Jonkers et al., 2010. Application of bacteria as self-healing agent for the development of sustainable concrete). There are many biological pathways that bacteria can use to form calcite (cement) with some of them used in self healing concrete (Dhami et al., 2013. Biomineralization of calcium carbonates and their engineered applications: a review). One of special interest to Mars is that a consortium of methane oxidising and sulfate reducing bacteria can convert underground aquifers to calcite through anaerobic oxidation of methane (Drake et al., 2015. Extreme 13 C depletion of carbonates formed during oxidation of biogenic methane in fractured granite)
Our only ever experiment in a new closed ecosystem, Biosphere 2, had an unexpected loss of oxygen which was eventually traced to carbon dioxide respired by the microbes reacting with the concrete used to make the habitat. (Severinhaus et al., 1994, Oxygen loss in biosphere 2).
In this case we are introducing life for the first time to a planet. It may be wise to try it out with smaller scale Mars simulation experiments first before introducing life to an entire planet.
One idea is to warm Mars up to make a CO2 rich atmosphere (if there is enough CO2) and then to use cyanobacteria to convert that CO2 to oxygen to make the air breathable, all the time using artificial greenhouse gases to keep Mars warm.
However in the other direction, if we accidentally introduce cyanobacteria and Mars doesn't yet have them, this might take CO2 out of the atmosphere making Mars less habitable.
Other microbes we introduce might fix carbon dioxide
Or at a later stage how well the cyanobacteria manage on Mars may depend on whether we have introduced fungal pathogens of cynaobacteria, or bacteriophages, or whether we have introduce microbes that graze on them.
We might want to introduce methanogens to use a methane boost to speed up warming of Mars as a natural warming process. But then methanotrophs may consume the methane.
Researchers find it hard to quantify the effect on Earth's climate of the melting permafrost and the microbes that grow in it.
Text on graphic: Wide variation in estimates of methane emissions. Permafrost may be a sink or a source
Figure shows estimates of the cumulative net carbon change by the year published of the estimates. The emissions may be methane or carbon dioxide. The date shows the year the estimates were made or the models done.
(IPCC, 2019, Special Report on the Ocean and Cryosphere in a Changing Climate : section 3.4.3.1.1, Figure 3.11)
IPCC 2019 Special report on the Ocean and Cryosphere Figure 3.11)
The earlier models all showed that significant carbon would be emitted as CO2 or methane. But from 2016 onwards more sophisticated models lead to much more uncertainty with some of them showing the melting permafrost as a net sink. The reason here isn't because of uncertainties in the physics, but of uncertainties in how the ecosystems will respond to the warming. The IPCC summarize the situation like this.
However, the latest model runs performed with either structural enhancements to better represent permafrost carbon dynamics, or common environmental input data (McGuire et al., 2016) show similar soil carbon losses, but also indicate the potential for stimulated plant growth (nutrients, temperature/growing season length, CO2 fertilization) to offset some (Kleinen and Brovkin, 2018) or all of these losses, at least during this century, by sequestering new carbon into plant biomass and increasing carbon inputs into the surface soil
(IPCC, 2019, Special Report on the Ocean and Cryosphere in a Changing Climate : section 3.4.3.1.1, page 252 of chapter 3)
In the case of Mars there are many unknowns including how terrestrial life would respond to local conditions, and whether there is any local martian life and if so, how it would respond. It may be just as complex or more so to predict effects of the biological responses to warming of the Martian permafrost after an accidentally started Mars Anthropocene.
Warming of the terrestrial permafrost is hardly a close analogue of the effect of introduced biology to a warming martian permafrost. But it's perhaps the closest analogue we have.
This figure shows some of the many complex processes involved in permafrost as it warms. The methane can be degraded by aerobic or anaerobic methanotrophs and some of it may reach the atmosphere. Some may be trapped as organics.
From: (Dean et al., 2017, Methane Feedbacks to the Global Climate System in a Warmer World : Figure 1) (note, license is non commercial no derivatives)
There would be many complex interactions and the effects would be likely to be unpredictable.
For instance introduced life may eat or compete with algae we attempt to use to convert CO2 to oxygen or methanogens that we may introduce or nitrogen fixing microbes, or nitrogen liberating microbes if we find large stocks of nitrates on Mars for the nitrogen which we'd otherwise have to source from comets.
This is just to touch on what is sure to be an immensely complex future topic if we do decide we want to terraform Mars. By then hopefully we have a far better understanding of the chemistry and physics AND any native biology of Mars. We might then need studies of Mars as complex and wide-ranging as the IPCC reports to use as a basis for our decisions and they are likely to better informed, more grounded if we do experimental tests of the effects of introducing terrestrial biology to simulated martian permafrost gradually warmed in Mars simulation habitats far larger and more sophisticated than our current Mars simulation chambers.
There may be many potential outcomes for Mars depending on the order in which we introduce microbes to the planet, and what is already there.
It's possible that even if Mars is uninhabited and has no interesting native biology or biochemistry and is not able to tell us anything about the early stages of the origins of life, even then we may well want to introduce life to Mars carefully. It might be that our choice of the first microbes to introduce to Mars could have a major effect on the outcome and that could be a reason to keep humans in orbit until we have established a biological succession of microbes on Mars and various biochemical cycles to our liking to support humans on Mars.
As one example that might be easy to miss, if we have the luxury of a "clean reset" without random contamination by humans, we can decide which microbial parasites they should have. We can introduce microbes with or without bacteriophages and select which bacteriophages we want them to have in the early stages of terraforming for Mars. We can also introduce microalgae with or without the dark matter fungi (Grossart et al., 2016, Discovery of dark matter fungi in aquatic ecosystems demands a reappraisal of the phylogeny and ecology of zoosporic fungi), recently discovered parasites of phytoplankton in the sea, originally discovered in fresh water. The marine dark matter fungi are described in the title of a 2022 paper as a mystery yet to unravel” (Ilicic et al., 2022, Basal parasitic fungi in marine food webs—a mystery yet to unravel)
Microalgae with parasites would be easier for other microbes to degrade [ADDCITE]. Without parasites they may be better for sequestering large amounts of carbon if that is what we wish to do.
Then any introduced cyanobacteria and pathogens of them would radiate to new strains and species and eventually new genera on Mars, possibly rapidly in the novel conditions.
Any introduced terrestrial pathogens radiating through a gradually Terraforming mars would encounter evolutionary pressures to:
- Be able to resist far more extreme temperature changes than on Earth from below -70 to above 15 C in one day and may well develop various cryoprotectants and chaotropic agents possibly including incorporating perchlorates into their intracellular fluids.
- Resistance to rapid changes on Mars may make some of them able to reproduce in freezers on Earth
- resilient to oxidants far more oxidising than on Earth
- Resilient to perchlorates
- Resilient specifically to ionizing radiation damage of their DNA rather than just as an accidental byproduct of desiccation resistance.
- develop the isomerases to be able to metabolize mirror organics from space as half the organics will be mirror organics.
- fungi with spores like Aspergillus would develop spores that spread better in even the very light winds and near vacuum conditions on Mars
- fungi and biofilms would develop extrapolymeric substances to protect against the very harsh conditions on Mars
- there would be a strong evolutionary pressure for ability to spread in dust storms which could include extra layers for spores, propagules incorporating iron oxides in some way.
- desert mosses on Mars would develop the ability to absorb water far faster and retain it through to midday.
- the fungal pathogens and the bacteriophages of terrestrial microbes introduced to Mars in the process of terraforming would also evolve independently of Earth in novel conditions.
All those changes would mean that after some period of time the microbes on Mars will be different strains, species and even genera. The rate of evolution is fast in microbes because of the rapid generation time, and depends on the timescale but also on the population. Given an entire planet to colonize this may happen quite quickly.
The timescale to fully terraform Mars to a breathable atmosphere even with megatechnology may be of the order of millennia to hundreds of thousands of years, see: Supplementary information under:
During this timescale most likely there are no impacts on Mars able to return life from Mars to Earth.
So there would seem to be potential for terraforming Mars to stimulate evolution of potentially invasive microbes. Issues could include, a fungal pathogen of cyanobacteria that terrestrial microalgae haven't encountered, a novel species of Aspergillus more easily spread to humans and more resilient to our natural immune system's antibiotics than Aspergillus fumigatus, a species that even if it radiated originally from Aspergillus but is so changed by evolution on Mars that it is no longer the same genus, microbes that are able to cope with the very low conditions in freezers to spoil food, and many other possibilities.
These are similar to the issues involved in returning distantly related but novel forms of terrestrial biology from Mars to Earth.
We could greatly reduce the risk of this depending on how we do the terraforming. For instance introducing only a select number of species and introduce the microbes without any pathogens, ether fungal or bacteriophages, then that would be a much more controlled situation so long as there is no native life on Mars able to complicate the picture. Then introduce more species once conditions on Mars are more similar to terrestrial conditions.
Even then the future evolution of that life may not be totally predictable or how those species might exploit / compete with each other on Mars or respond to the Martian conditions. And even a pathogen free species of chroococcidiopsis, that has evolved in Martian conditions in large numbers for a few years might not be safe to return.
In this scenario of a highly controlled terraforming then humans might not land until far later but by the time they do land on Mars the initial microbes might have evolved far enough so that the effects of returning them to Earth are no longer predictable.
Then there's the risk that life introduced to terraform Mars may have the opposite effect of rapidly unterraforming it. The atmosphere is in an equilibrium with the dry ice at the poles keeping it barely habitable.
McKay et al. found that the Martian atmosphere is unstable in the region between 6 ppm and a little over 30 ppm with any increase in CO2 condensing back as dry ice at the poles rapidly restoring it to 6 ppm. if Mars has enough dry ice for a thick atmosphere, to terraform it, we need to rapidly push it up from 6 ppm of CO2 to over 30 ppm before the CO2 we added sublimes back out as dry ice at the poles. They suggest using artificially generated greenhouse gases to achieve this with 500 power stations working for a century to produce the gases. Once we reach that point any remaining dry ice will quickly evaporate in a runaway warming effect.
However we now know that there is only enough dry ice at the poles for 12.5 millibars in a runaway greenhouse effect, because this runaway greenhouse actually happens at times when Mars' axis is tilted far enough for the south polar ice cap to evaporate (Bierson et al, 2016, Stratigraphy and evolution of the buried CO2 deposit in the Martian south polar cap : 4176). Also at present the dry ice is covered in water ice caps (sandwiched with the dry ice in three separate layers) that prevent it subliming, and the most recent runaway greenhouse which started 40,000 years ago has only liberated a tenth of a millibar instead of the usual 6 millibars. We seem to be in the middle of that phase at present, if so we may be able to liberate the remaining 6 millibars by breaking through the caps of water ice sufficiently to let the dry ice erupt from below as dry ice geysers. For this dry ice feedback cycle see: Supplementary information under:
This may be solvable, for instance Mars has the equivalent of several times Earth's atmosphere locked up in the dust and rocks as carbonates. This can be liberated relatively easily with acid and there are some lime boring microbes that can do that for us. But we would need to set up a biosphere artificially that uses those microbes to return the CO2 in the place of the plate tectonics which returns the carbonates via volcanoes on Earth.
That would need to be a fully functioning biosphere that can maintain itself, or some way to keep it in balance so that it does continuously return the CO2 as desired and not do the opposite and sequester more and more carbonates. However with the current thin atmosphere it will be hard to kick start that process. With not enough dry ice to push the atmosphere above 12.5 millibars , we are stuck at 6 millibars because of the dry ice feedback, together with any use of warming gases to create marginally more habitable conditions on Mars. So we have to find a way to liberate carbon dioxide from carbonates in a cold dry Mars not much warmer than today.
If we do manage to set up a thicker atmosphere by liberating the carbon dioxide from carbonates, and we g et things wrong, Mars may get into a "Swansong Gaia" state, or if it has native life, it may be in it already, where it rapidly reduces its habitability until it is barely habitable by sequestering carbon dioxide as carbonates. It is possible we may need a carefully constructed artificial biosphere to avoid this end state. I suggested above that if Mars does have life it might already be a Swansong biosphere.
I discuss terraforming and the proposal to use lime boring microbes to return the carbonates to the atmosphere as CO2towards the end here:
I discuss Chris McKay's terraforming and ecopoesis ideas and how they could be applied to present day Mars in the Supplementary information under:
These may all be solvable issues. However the issues seem complex with many potential pitfalls and they seem to require large-scale megatechnology.
If we do decide to go ahead with such a project, it might be wise to do tests and experiments in smaller habitats outside of Mars and a wide ranging program of study to try to anticipate the effects before deciding
- whether to terraform Mars and if so,
- how exactly, including how to liberate the carbon dioxide from the carbonates
- with which microbes (e.g. methanogens or cyanobacteria and lime boring ) and which grazers and microbial pathogens (e.g. fungal pathogens or bacteriophages) to regulate those microbes
- in which order.
Choices we make here may lead to different end states for terraformed Mars.
It is far easier to paraterraform (cover with habitats) either Mars or Phobos indeed, or to build habitats in space. I go into details here:
Valuing any martian uninhabited habitats: how discovery of prebiotic chemistry or the first steps of a second genesis of early life could revolutionize understanding of life's origins
[FIXCITES (HTML MARKUP]
[IS THIS BEST KEPT HERE, OR BEST TO MOVE SOME INTO THE SUPPLEMENTARY INFORMATION WITH SUMMARY HERE?]
If there is no harmful life for humans on Mars, it is still not yet equivalent to the Moon and we have other things to consider that we don't need to consider for the Moon.
For Mars to be like the Moon, with no issues for humans visiting there in the forward direction, we need Mars to have:
- no interesting early life prebiotic or abiotic chemistry - Mars is the only terrestrial analogue of Earth within light years - once we introduce terrestrial life no future generations on Earth will ever be able to study current chemical processes on present day Mars, just hints of how they worked in protected deposits.
- that it doesn’t matter what order terrestrial microbes are introduced to Mars - some terrestrial microbes may be able to turn subsurface aquifers on Mars to cement for instance.
We already looked at how the order we introduce microbes may matter, above in:
We've also looked at the potential for microbially diverse oases of extant life
In this section we'll look at issues that might arise if we find that Mars has interesting but harmless prebiotic or abiotic chemistry or early life.
We never find much by way of complex prebiotic or abiotic chemistry in natural conditions on Earth because even cooling lava from a volcano is only briefly uninhabited and is quickly colonized by terrestrial life. Even there we work to preserve lifeless deserts, biologically reversing our contamination as far as possible in the McMurdo dry valleys.
Chris McKay says that we need to consider the ethical value of lifeless deserts on other planets and may need to preserve static lifeless deserts.
Environmental ethics and policy have been largely developed around the concept of nature as a dynamic collection of living beings in direct interaction with humans. However, when we consider the Moon, Mars, and other worlds we encounter profoundly static and lifeless nature with essentially no history of human interaction. On what basis do we make decisions on the preservation or utilization of such lifeless landscapes? Here I suggest that static lifeless landscapes on other worlds have some parallels on Earth in the upper elevations of the Antarctic Dry Valleys and the central depression of the Atacama Desert in Chile and Peru. In the soil of both locations life is absent or cannot grow and the timescales of landscape evolution vastly exceed human timescales. For all intents and purposes, these are static and lifeless landscapes. Today, human activities in Antarctica are carefully regulated with a view toward preservation while in the Atacama Desert resource mining has extensively altered the landscape. Thus, these two sites provide alternate possible futures for the Moon and Mars.
Preservation of static lifeless landscapes could be based on two attributes. First, they provide a way to further define and understand the Earth and the limits of life—which is the current scientific motivation for biological and geological research activities there. Second, both on Earth and particularly on other worlds, lifeless landscapes may express states that are new to human experience akin to new forms of art or new branches of science. We don't understand these environments, their nature, or their relationship to us. In the case of Mars there may come to be a tension between preserving a lifeless landscape and introducing or enhancing life on that planet.
(McKay, 2019,. Preservation of Static Lifeless Landscapes in the Antarctic Dry Valleys and the Atacama Desert and Applications to the Moon and Mars)
However similar conditions on Mars could remain uncolonized by martian life for thousands or millions of years, and if Mars has never had life, perhaps they could have never had life even for billions of years. Mars could have many uninhabited habitats with no life in them (Cockell, 2014, Trajectories of martian habitability).
This then could be very interesting for the origins of life, because life is thought to have evolved from prebiotic chemistry slowly over millions of years of the chemical environment getting more an m ore complex in various ways until eventually the first precursors of life evolved, through chemical evolution, transition from less organized chemicals to more organized chemicals. There are many ideas for how that could have happened.
If we introduce terrestrial life to start the anthropocene on Mars, we may become the last generation with the opportunity to study what happens to a terrestrial planet like Mars with surface water, organics, infall of organics from space, and complex chemical reactions that provide the energy that life can use if it evolves. We could be the last generation with an opportunity to study prebiotic or abiotic chemistry on a terrestrial planet in our solar system.
Up to as recently as a decade ago we would have had no choice. Even with Viking levels of sterilization, we had no way to effectively sterilize a rover or lander on Mars sufficiently to explore a habitat suitable for terrestrial life to thrive without a significant risk of introducing terrestrial life to it, except possibly in ultra cold habitats that terrestrial life would colonize only very slowly, or extreme habitats beyond the capabilities of most or all terrestrial life to colonize.
We had the choice, to leave the most interesting but most vulnerable regions of Mars unstudied, for future generations, or to study them and risk introducing terrestrial life, as we saw above in:
It was the same choice for uninhabited habitats anywhere in our solar system - whether on Mars or Europa, Enceladus, Ceres, etc,
But today because of the advances of science, we no longer need to make that difficult choice whether to explore uninhabited habitats in our solar system, knowing that we run the risk that we erase much of their interest for ourselves and for future generations by starting an anthropocene on those bodies, or to leave them for future generations to study. Ours is the first generation to have the opportunity to explore uninhabited habitats with truly zero chance of introducing terrestrial life. We can do this if we if we decide to prioritize the development of 100% sterile rovers and landers, and to keep humans in orbit for now until we know what we might lose, as we saw above in the sections:
- How to do a rapid 100% heat sterilized unmanned biological exploration of Mars using a simpler version of NASA's Venus HOTTech
- Two technology pioneers for 100% sterile exploration: a proposal from 2017 to use the technology for a Venus surface rover to explore biologically sensitive habitats, and a proposed Europa cryobot from 2019
- A 100% sterile Marscopter to explore sensitive local features like RSLs needs it to resist only brief heating at 300°C after deployment
- Humans in orbit around Mars exploring with immersive VR using 100% sterile surface avatars and what they could do
- A civilization’s choice: protecting Mars and Earth 100% now keeps all future options open until we fill knowledge gaps
So, supposing we find we are in a scenario with no life or only early life on Mars. Does it matter if we introduce terrestrial life to uninhabited habitats with abiotic or prebiotic chemistry or early life in them, and how important is it?
My aim in this chapter is just to cover a few of the main strands of thought about what prebiotic and abiotic chemistry could involve. We are not able to make fully functional examples of any of these ideas. We can't make even the simplest ribocell or RNA world cell with just a few genes in it or even the far more primitive protocells that may have come before them.
Then if we can't make them, you might think we could create conditions for early life to evolve in our laboratories. But that is well beyond our capabilities too. We can’t simulate in our laboratories the effect of millions, or billions of years of prebiotic chemistry on another world. We can't even simulate 1000 years of chemical evolution of prebiotic chemistry in a small cave.
We can use directed chemical evolution to try to speed up some of the processes of prebiotic chemistry. The idea is to select some goal, perhaps complex sequences of RNA for the RNA world hypothesis, and then speed up evolution by selecting the products at each stage that best approximate a hypothesis for how early life evolved, but this depends on informed guesses about how prebiotic chemistry developed.
If we don't know yet how early life evolved, then on the timescales and spatial scales available for human experiments in laboratories, we can't directly simulate even a thousand years of chemical evolution from prebiotic chemistry in,a small sulfur cave warmed by hydrothermal processes - or any other environment we might consider to be an optimal habitat for the origins of life.
The scenario of no life on Mars may be a wonderful opportunity to give us clues about what may have happened on the path to the evolution of life on Earth and what may be happening right now on other terrestrial planets in our galaxy that it may be impossible for us to discovery in any other way realistically in the near future. The nearest terrestrial planets in the habitable zone of a star outside our solar system would be light yeas away and realistically we are not likely to be able to study them close up in less than centuries.
Potential of Mars for a variety of short lived and longer lived isolated habitable "oases" in caves, surface isolated oases and so on where prebiotic, early life or abiotic chemistry might explore different pathways
We saw that Mars might have microbial oases of life which might each have a different distribution of microbial species, similar to the Galapagos islands, with some microbes able to spread between them and others confined to within the oasis.
Prebiotic oases or early life oases could be even more varied because there may be no way for early life or prebiotic chemistry to spread between them. In this way an uninhabited Mars could have numerous isolated oases of habitability on the surface as well as caves that might explore different prebiotic and abiotic pathways towards life. They could show us different stages of that evolution too, with none of them ever reaching early life quite. Or in the most exciting scenario of all they might some of them have early forms of life making their first steps of evolution towards the complexity of modern life. Different microbial oases might explore different paths. In the best case for early life studies here we might have not just one genesis of early life on Mars but dozens of them.
Mars could have a large variety of prebiotic oases that do these experiments for us, habitable for time periods of thousands to millions or even billions of years.
There may be many short lived habitats of this sort. For instance, lakes formed 210 million years ago on one of the flanks of Arsia Mons as a result of volcanism, two of around 40 cubic kilometers of water each, and a third one of 20 cubic kilometers of water. They probably stayed liquid for hundreds to thousands of years, covered in ice and kept warm by a subsurface hydrothermal system (Scanlon, 2014, A habitable environment on Martin volcano?).
5.1. Englacial and basal lakes
The glaciovolcanic landforms in the Arsia Mons FSD are likely to have generated several englacial lakes with volumes on the order of tens of cubic kilometers and longevity of hundreds to thousands of years. The few terrestrial volcanically generated englacial lakes whose ecosystems have been surveyed contained microbial communities capable of fixing carbon and nitrogen, and including organisms related to known acetogens, sulfate reducers and iron reducers. These communities are supported by sediments and bottom waters rich in volcanic CO 2, H2, ferric iron, and sulfur compounds, as well as oxygen from the melted glacial ice
(Scanlon et al., 2015. Volcanism-induced, local wet-based glacial conditions recorded in the Late Amazonian Arsia Mons tropical mountain glacier deposits : 26)
6. Conclusion
...
Previous work has made a strong case for cold- based glaciation at the Tharsis Montes, but new, higher-resolution data show that landforms indicative of local wet-based conditions due to interaction between lava and glacial ice are also distributed throughout the deposit. These landforms, which record local increases in ice-flow velocity and polythermal conditions, include thrust-block moraines, ribbed moraines, highly arcuate drop moraines, streamlined knobs, and fluvial outflow channels.
Since these landforms all occur exclusively in association with glaciovolcanic features, we conclude that the Arsia Mons FSD was left by a glacier whose local wet-based conditions were induced by volcanic warming rather than by climate. The aqueous environments evidenced by the Arsia Mons FSD are exceptional in that they are comparable in size to similar Noachian-Hesperian environments, and potentially much longer- lived than other Amazonian environments. Furthermore, the Arsia Mons glaciovolcanic environments occurred recently enough that unambiguous molecular biomarkers have been retrieved from much older terrestrial environments.
(Scanlon et al., 2015. Volcanism-induced, local wet-based glacial conditions recorded in the Late Amazonian Arsia Mons tropical mountain glacier deposits : 29)
[ILLUSTRATION NEEDS PERMISSION]
Illustration showing wet based ice on both sides of the volcano and fluvial channels draining from one of the ares (Scanlon et al., 2015. Volcanism-induced, local wet-based glacial conditions recorded in the Late Amazonian Arsia Mons tropical mountain glacier deposits : Fig. 13)
We don’t know how quickly life can evolve - is that enough time? Could Mars have newly evolved early life local to Arsia Mons from 210 million years ago? ? Will we find traces of early life still there maybe in geothermally heated caves?
210 million years ago is relatively recent in geological time and there are other signs that Mars is still geologically active, so it could have hydrothermal processes deep below the surface even today that we haven't yet detected. For a short summary of this research see the introduction to:
Lava tube caves on Mars are expected to be 40 to 400 m high on Mars and wider than they are high, compared to 10 to 30 meters in diameter on Earth (Sauro et al., 2021, Lava tubes on Earth, Moon and Mars: A review on their size and morphology revealed by comparative planetology : 13).
Lava tubes could be of interest if they formed under a glacier or in the presence of ice and water. Other types of caves such as fracture caves or solutional caves (like our terrestrial limestone caves) might also be vast in the Martian gravity and may also be biologically isolated from each other, with life or prebiotic chemistry only able to spread from one to the other if it can survive transfer in the harsh surface conditions on Mars. Some may be kept warm by geothermal processes. These may provide temporary conditions similar to the hydrothermal vents proposed as one of the candidates for the origin of life.
So there may be subsurface hot spots today perhaps creating conditions for subsurface caves filled with water or sulfuric acid (Boston, 2010. Location, location, location! Lava caves on Mars for habitat, resources, and the search for life ).
Most would not be detectable from orbit.
Snottites in Ceva de Villa Luz, a sulfur cave in Mexico which may be an analogue of similar caves on Mars. With its abundance of sulfur Mars may have many sulfur caves.
Though we have not yet detected geothermal heating on Mars, it has been volcanically active within the last few tens of thousands of years. So it's not impossible some are geothermally heated and able to host life and may be open to the surface.
(NASA, 2006, Cave Slime)
For details about the potential for habitable caves on Mars see the section:
Depending how fast life evolves, perhaps life could evolve from scratch in one of these temporary habitats, then find its way to the surface, perhaps in the RSLs, and then be transported in the wind. Perhaps it may adapt to surface conditions and spread for a while in the brine layers found by Curiosity in localized oases on the surface above the temporary habitat. This is just one scenario, to motivate the possibility of newly evolved life on Mars.
There could be many isolated oases on the surface too, for prebiotic chemistry or early life similarly to the possible situation for microbial life, see above:
In the best case (from the point of view of the study of early life) Mars could have multiple unconnected potential habitats, uninhabited and inhabited, preserving different stages of evolution from complex chemistry to life.
This would give a fine grained understanding of stages in the processes of development of life from non life. We could use present day Mars like a time machine to take us back to various stages of the early stages of life and perhaps get some idea of what happened on our own planet right at the beginning before life evolved.
Many ideas for how early life could have started, which Mars might be exploring for us right now in uninhabited habitats
Perhaps some such habitats could have RNA and autopoetic cells (Stano et al., 2010. Chemical Approaches to Synthetic Biology-From Vesicles Self-Reproduction to Semi-Synthetic Minimal Cells) but no life. An autopoetic cell is one that is able to maintain itself by taking in materials and using energy from the environment. However it wouldn't count as life as such, it's prebiotic chemistry.
Text on graphic: Minimal self maintaining cell.
Autopoetic cell maintains its boundary using materials that it lets in and transforms.
Early or prebiotic life
Genetic and metabolic network [transforms ingredients and produces the cell wall lipids]
Based on (Stano et al., 2010, Chemical Approaches to Synthetic Biology: From Vesicles Self-Reproduction to Semi-Synthetic Minimal Cells : Figure 1)
The idea is that , inside the cell, there is a network that turns these precursors into the cell wall itself, as well as using them to regenerate itself, and expels waste products. The vesicle as it gets larger splits to replicate, or alternatively, it creates a daughter cell inside which then leaves the cell. In these primitive protocells, this is regulated, for instance, by the surface area to volume ratio.
This could happen without any DNA or RNA to regulate it. This process happens with some fatty acid vesicles for instance. Some researchers are working with Butschli droplets, a complex mixture of oils and other chemicals such as detergents, that behave rather like cells to explore such ideas. These are either droplets of oils in water or of water in oil. These are not likely precursors for us, e, but are examples that work like protocells which let us explore artificial life scenarios in a different medium.
Nobody has ever managed to demonstrate a living protocell that can assemble itself. But it is possible to provide droplets that are capable of self-organization and self-propulsion too, seeking out the chemicals they need to grow, but not through self-assembly as they are just simple combinations of chemicals.
With regard to synthesizing life using protocells simply from self-assembly of components, no one has successfully demonstrated a living protocell based on the principle of self-assembly despite more than 100 years of experimentation. This is one of the main motivations for producing a different type of protocell based on self-organizing self-propulsion.
(Hanczyc, 2014, Droplets: Unconventional Protocell Model with Life-Like Dynamics and Room to Grow : 1045)
He speculated that this might permit more open thinking about how non-living matter might self-organize into something resembling life, not dependent on the familiar biology of DNA and enzymes.
Immediately from the first analysis of self-movement and chemotaxis we were able to establish the link between environmental sensing and direction and mode of motion. This intimate link between the environment and protocell behavior may form the basis for higher order functionality in such simple nonliving protocell systems [38]. The idea of dynamic droplets as protocells promotes more open thinking about how non-living matter might self-organize into evolving matter that adapts over time to a changing environment. Perhaps such simple dynamics in simple physical systems will constitute in the future the first true embodiment of artificial life that is an orthologous departure from the one familiar type of biological life based on DNA and protein enzymes. This lifts the constraints of searching for liquid water-based life in the universe where such conditions are not feasible
(Hanczyc, 2014, Droplets: Unconventional Protocell Model with Life-Like Dynamics and Room to Grow : 1045)
Video: Martin Hanczyc: the line between life and non-life
Or perhaps life started with self-replicating chemicals. As an example of this approach, Domingo has suggested that viruses may have come first, if so we could find a world of viruses without life.
Life is characterized by four integrated features: replication, evolvability, metabolism, and compartmentalization. It is debated whether replication or metabolism was the dominant triggering factor.
At least two ancestral positive selection events might have contributed to the development of life: the selection of replicating over nonreplicating polymers, and selection of splitting-prone versus nonsplitting-prone membrane vesicles.
- Present-day viruses may be descendants from the primitive replicons that participated in early life prior to cellular organizations, or from structured cells by escape or reduction.
- Key features of many present-day viruses may be an inheritance of primitive replicons, notably error-prone replication, spread through membrane structures, and tendency to engage in genome integration and transfers.
- The geological record indicates multiple, brief, and drastic mass extinction events in Earth’s history. Such events anticipate mass extinction of the resident viruses. A dynamics of emergences, reemergences, and extinctions might have operated historically at a grand-scale, with mechanisms similar to those we observe with present-day viruses. Present virosphere dynamics suggests difficulties to reconstruct events that spanned millions of years.
(Domingo, 2020. Introduction to virus origins and their role in biological evolution
If Mars still has an RNA world somewhere perhaps amongst the underground caves, it could have viruses without cells, or cells without viruses, so giving some evidence of which came first. Again we can't make a self-replicating virus that can replicate without the cell machinery of a cell to infect.
Text on graphic: First viruses arise before cells
First cells arise before viruses
Virus lineages shown in dark red.
Annotations added to: (Domingo, 2020. Introduction to virus origins and their role in biological evolution : figure 1)
[Shared by Springer Verlag under the Elsevier's Novel Coronavirus Information Center license which gives permission to reuse the research in any form by any means with acknowledgement of the original source, for any research published in it before July 2023 (Elsevier, 2023, Novel Coronavirus Information Center)]
Or perhaps life started with both working together as in Domingo's second example, of RNA world cells. In this case there may be reproducers and replicators that co-evolve.
A simple model for early evolution of self replicating reproducers. In this diagram the black dots are resources in the environment that the cells take up. The yellow shaded cells are able to reproduce themselves but can't replicate their contents
The purple strands are able to replicate themselves, the green ones can't.
Both types of cell reproduce when the contents reach some value, in this example arbitrarily set to seven of the black dots.
Over time some proto cells may eventually emerge that can both reproduce and also replicate their internal chemistry in the new protocells.
Cells die when they no longer have any of the black dot resources.
Annotations added to: (Babajanyan et al., 2023, Coevolution of reproducers and replicators at the origin of life and the conditions for the origin of genomes : figure 1)
In terrestrial life, most of the genetic information is stored in DNA and has to be translated to messenger RNA which is then translated into proteins using the very large ribosomes which in turn are made up of proteins and fragments of RNA which also have to be made from the DNA and the ribosomes are used to make the enzymes which do the translation of DNA into messenger mRNA and catalyze many other processes. So how did all this get started?
The idea of the ribocell is a cell that has only RNA, no DNA, no proteins, and the enzymes are "ribozymes" made up of fragments of RNA without proteins. It is a field of very active research at present (Kun, 2021. Maintenance of Genetic Information in the First Ribocell).
The big challenge at present in the field of research into ribocells is to make a ribozyme that can assemble an RNA strand as complex as itself from simpler genetic material in the cell. The main tool we have for this is directed evolution in chemical solutions, selecting more complex fragments of RNA that are able to catalyze joining simpler fragments together.
. A fully functional example of this ribozyme is so far elusive.
...
a breakthrough came in 2011 when a ribozyme capable of synthesizing a strand of up to 98 nucleotides was selected. A ribozyme can extend a primer with more than 200 nucleotides [43], but requires a very specific template. Unfortunately, this specific template is not its own sequence or its complementary sequence. Thus, ribozymes are capable of synthesizing long strands based on a template, but their generality is still a question.
(Kun, 2021. Maintenance of Genetic Information in the First Ribocell).
Joyce lab (Joyce, n.d., Joyce lab) specialize in this directed evolution, which has got as far as a ribozyme that was able to synthesize its own ancestor in this process of directed chemical evolution of complex molecules able to act as enzymes to synthesize other simpler complex molecules. (Tjhung et al, 2020, An RNA polymerase ribozyme that synthesizes its own ancestor)
Text on graphic: Joyce lab created a ribozyme through directed evolution that made the three strands of this ancestor ribozyme which self assembled but couldn't make itself
Most copies also had mutations which prevented them functioning
Graphic from press release:
(Joyce, 2020. Ribozyme that synthesizes its own ancestor)
Steven Benner and Paul Davies are of the view that the very small structures in the meteorite ALH84001 just might be fossils of simpler RNA world cells from an earlier form of life without those large ribosomes and without the proteins
"Why should proteins be universally necessary components of life? Could it be that Martian life has no proteins?
... Life forms in the putative RNA world (by definition) survived without encoded proteins and the ribosomes needed to assemble them. ... If those structures represent a trace of an ancient RNA world on Mars, they would not need to be large enough to accommodate ribosomes. The shapes in meteorite ALH84001 just might be fossil organisms from a Martian "RNA world".
(Benner et al., 2012, Towards a Theory of Life : 37)
There has been enough interest in the possibility of nanobacteria with a simpler biochemistry to search for a terrestrial “shadow biosphere”. Phillipa Unwin observed nanobes only 20 nm in diameter, far too small for familiar life, but she found some disputed evidence that they could contain DNA (Cleland, 2019, The Quest for a Universal Theory of Life: Searching for Life as we don't know it : 213 - 214)
Or the precursors of life could consist of chemicals that replicate, with no metabolism or cell wall. One possibility, for the RNA world hypothesis, is some kind of a mineral substrate. That's the idea of "naked genes" which for instance the chemist Leslie Orgel favours:
"A scenario that I personally find attractive is one in which the very first replicators were 'naked genes' adsorbed on the surface of mineral particles, and in which impermeable membrane caps were 'invented' by the genetic system as it became metabolically competent. Escape from the mineral surface, enabled by the development of a closed spherical membrane would occur at a relatively late stage in evolution"
(Orgel, 2004, Prebiotic Chemistry and the Origin of the RNA World)
Different habitable regions could differ in the type and complexity of the prebiotic chemistry, again in ways we can never simulate or study once the regions are taken over by Earth life
To give a few examples, if the martian surface is at a very early stage of prebiotic chemistry even after 4.5 billion years, some habitats might have those autopoetic cells (Stano et al., 2010. Chemical Approaches to Synthetic Biology-From Vesicles Self-Reproduction to Semi-Synthetic Minimal Cells). Or in some places on Mars, we might find the ‘naked genes’ adsorbed on the surface of mineral particles, and perhaps with impermeable membrane caps ‘invented’ by the genetic system (Leslie, 2004. Prebiotic chemistry and the origin of the RNA world) or any of the other ideas for prebiotic chemistry including the ones we touched on in the last section.
Illustrative example, uninhabited habitats could explore various suggestions for the origins of chirality - the preferred symmetry for life
Depending on how biologically isolated they have been from each other, and for how long, some of these uninhabited habitats might have be normal and some mirror life. We might even be able to study a chemical network (chiral network) shifting from ordinary to mirror life according to one popular theory, punctuated chirality (Gleiser et all, 2008, Punctuated chirality). We covered this scenario above in :
Or perhaps following another idea for how life ended up all as normal rather than mirror life, is that it forms huge crystals of organics all of one symmetry. In a solution with a mix of crystals of various sizes, the smaller ones often dissolve and reaccrete onto the larger crystals are more energetically favourable. The organics can change symmetry as they do so flipping to the mirror image to accrete onto the large crystal (solution phase racemization)> Eventually this ends up with one big crystal. This is one theory for how modern life got its chirality - the preference for one form of a molecule over its mirror image. The process is known as "Ostwald ripening" and it could take all the amino acids of one chirality out of a solution into one big crystal, amplifying a tiny signal of a slight excess of one version of an amino acid over its mirror image (Blackmond, 2010. The origin of biological homochirality : figure 7).
There are two main types of chiral molecule, those that crystallize out as a compound, where both symmetries combine to make a a solid and those that crystallize out as a conglomerate, the molecules of a crystal all have to be the same symmetry, so it forms a mix of crystals, some of one symmetry and some of other symmetries. About 1 in 10 of chiral molecules form conglomerates.
In 2005 the chemist Viedma discovered that if you stir a solution that crystallizes out as a conglomerate, and you also include glass beads in the solution so they are moved around as it's stirred, then the crystals dissolve and reform over and over until all of them are of one symmetry. He found that this can happen quickly in days. Other researchers quickly reproduced his results and found that various refinements can reduce this to hours. There are four main ways this can happen, through turbulence, ultrasound, frequent cold to hot and back again rapid changes of temperature, or if the small crystals grind against each other as they are stirred.
There are two ways this can happen. Some crystals are asymmetrical in solid form but they are symmetrical when in solution, so then any molecule in solution can join onto a new crystal of either symmetry as it crystallizes out. The other way it can happen is if the molecules can change to the opposite symmetry in solution or as they attach themselves to the new crystal.
In 2005, Viedma reported that solid-to-solid deracemization of NaClO3 proceeded from its saturated solution by abrasive grinding with glass beads. Complete homochirality with bimodal distribution is reached after several hours or days. The process can also be triggered by replacing grinding with ultrasound, turbulent flow, or temperature variations.
(Sallembien et al., 2022. Possible chemical and physical scenarios towards biological homochirality)
This illustrates the idea
Viedma ripening, the solution is stirred using spinning magnet rotors in the flasks with glass beads which lead to the crystals as the form grinded to pieces then reforming
Over a period of days, or even hours, the original solution with a mix of crystals of both symmetries gradually turns into one of only one symmetry.
(Sögütoglu et al., 2015, Viedma ripening: a reliable crystallisation method to reach single chirality : Figure 1)
So some of these caves or isolated regions of prebiotic chemistry might have huge Ostwald crystals for us to discover. Or the processes of Viedma ripening may be going on right now on Mars.
There are many other suggestions for how life could have got its preference for molecules all of one symmetry. It could have happened in space before early organics were delivered to Earth, or on Earth before the origins of life, or at various stages in the process of evolution of prebiotic chemistry and early life.
An overview of ways that life could have got its preference for one of the two possible symmetries for its organics at various stages of prebiotic chemistry and evolution of early life. Some of these mechanisms assume a bias towards one symmetry over the other in the organics when they first form on Earth or the ones delivered from space which is then amplified.
LUCA = Last Universal Common Ancestor
From: (Sallembien et al., 2022. Possible chemical and physical scenarios towards biological homochirality : fig 19) which covers these topics in detail.
One of the more popular ideas is that the Earth was seeded by organics from space that already had an excess of one of the symmetries, which was then amplified by other processes on Earth. We do find complex organics in the gas clouds that collapse to form star systems, such as amino acids. There aren't many physical processes that can sort molecules in that way in space but one mechanism that does work is circularly polarized light. Astronomers detect this form of light in star forming nebulae like the Orion nebula though only in parts of the nebula not all of it (Fukue et al., 2010. Extended High Circular Polarization in the Orion Massive Star Forming Region: Implications for the Origin of Homochirality in the Solar System). More cites in the sections on circularly polarized light in: (Sallembien et al., 2022. Possible chemical and physical scenarios towards biological homochirality)
There are numerous other proposed explanations. Some physical, prebiotic. Some due to life processes themselves. This graphic summarizes the main categories of ideas.:
In this graphic, the abbreviations are
PVED: parity-violating energy difference. This is a small asymmetry in fundamental physics. It has few noticeable effects, but one example is that when cobalt 60 decays to nickel 60 it only emits electrons with a left handed spin because W+ and W- bosons can only couple with left handed electrons. Effects like this would likely only lead to a very weak excess that would need to be amplified. But if this is the reason, it is the only explanation that could lead us to d expect life universally in our universe to have the same mirror symmetry.
SMSB: spontaneous mirror symmetry breaking. The Soai reaction is a chemical reaction that rapidly amplifies a small chiral excess discovered by Soai in 1995 and is one example of SMBS. It could go either way but can turn a small excess into a large excess.
From: (Sallembien et al., 2022. Possible chemical and physical scenarios towards biological homochirality : figure 1) which covers these topics in detail.
If Mars has no life but has prebiotic chemistry we may still be able to learn more about whether and how this happens or rule out the possibility of a prebiotic selection if it turns out that we don't find any preference in habitable regions with prebiotic chemistry. We might be able to explore many of these proposed mechanisms, with different processes happening in different places on the surface of Mars or in caves.
Also, more speculatively, some locations might have chirality indifferent early life or prebiotic chemistry, with enzymes such as Joyce’s RNA enzyme which can replicate RNA of opposite chirality including its own mirror version (Joyce, 2007. A glimpse of biology's first enzyme) (Sczepanski et al., 2014. A cross-chiral RNA polymerase ribozyme) (Singer, 2014, New Twist Found in the Story of Life’s Start )
Opportunity to study prebiotic chemistry in action, on its first steps towards life - or if there is no interesting prebiotic chemistry, perhaps complex chemistry that is unsuitable to ever evolve into life - like a time machine to take us back to conditions on early Earth
We could study these processes actually in action, study naked genes as they are adsorbed on surfaces, growth of Ostwald crystals, or the activity of protocells or origins of chirality in the native environment in which they developed.
Or perhaps there is nothing on Mars anywhere of any real interest to prebiotic chemistry. Perhaps none of these hypothesized processes happen anywhere. That also would be a major discovery suggesting that it must be exceptionally difficult for chemistry to take even the first steps on the path to life. We know that we will find very complex chemistry and complex molecules. We find these even in the meteorites that fall on Earth, and those also fall on Mars, and then they get processed further. But they may not have any ordering or patterning to them that seems likely to be useful for the evolution of life.
If these habitats with or without prebiotic chemistry are habitable to Earth microbes, the organics produced by the prebiotic chemistry might be very attractive as a food source to terrestrial life. If so, how long would they remain in a state suitable for study by astrobiologists and geochemists after infection by even one microbe or dormant spore capable of replicating in them?
Perhaps even an intact microbe or microbial spore is not needed. Infection with fragments of RNA or enzymes from Earth microbes could be enough to give protobionts in these habitats at a late stage in chemical evolution the missing key to become a simple form of replicating life. The resulting life could be interesting in its own right, but this process could erase all traces of the pre-existing protobionts, so though in this case we would end up with an interesting form of life we could never create by ourselves, a simpler life-form that has incorporated some of the biochemistry of terrestrial life into it, we might never get to study it as a living cell in its original state.
Study of the Martian meteorite NWA 5790 has revealed small vesicles that may provide bioreactors for early life to evolve even in the more recent Amazonian period on Mars (Viennet et al., 2021. Martian Magmatic Clay Minerals Forming Vesicles: Perfect Niches for Emerging Life?) Suppose they are, but life has not yet evolved? What a wonderful opportunity to study this process? There may be no other planet within light years to gain such insights from.
Mars could have either prebiotic life or gone beyond those early steps at prebiotic chemistry to very early life, perhaps recently evolved in temporary surface habitats cut off from its deep hydrosphere. This earliest life could predate Darwinian evolution of cells based on Weiss’s idea of simple modifiable cells with no barriers to uptake of genetic material from other cells evolving through Lamarckian evolution with Darwinian evolution only of the genetic material itself:
Vulnerability of such a treasure as early pre-Darwinian life to terrestrial life
However such a planet would be exceptionally vulnerable. If life is still at an extremely early stage of evolution on Mars with early life with modifiable cells, or prebiotic with life not yet evolved, it might be only a matter of time after the first human boots on Mars before introduced terrestrial life reigns supreme in all the habitats on such a world, both previously uninhabited and habited.
If Mars does have early life, it might be completely harmless to Earth's biosphere., According to one idea, the earliest life, all the way through to the last universal common ancestor (LUCA), might have been simple “modifiable cells” capable of taking up “naked” genetic material which evolved through lateral transfer, by Lamarckian rather than Darwinian evolution. It is the community as a whole that evolves, the whole ecosystem of all the components. However individual cells in the community are somewhat different and over time this transitions to a more vertical Darwinian type evolution with the cells evolving in competition with each other.
… Aboriginal cell designs are taken to be simple and loosely organized enough that all cellular componentry can be altered and/or displaced through HGT [Horizontal Gene Transfer], making HGT the principal driving force in early cellular evolution. Primitive cells did not carry a stable organismal genealogical trace. Primitive cellular evolution is basically communal.
The high level of novelty required to evolve cell designs is a product of communal invention, of the universal HGT field, not intralineage variation. It is the community as a whole, the ecosystem, which evolves. The individual cell designs that evolved in this way are nevertheless fundamentally distinct, because the initial conditions in each case are somewhat different. As a cell design becomes more complex and interconnected a critical point is reached where a more integrated cellular organization emerges, and vertically generated novelty can and does assume greater importance.
(Woese, 2002. On the evolution of cells)
See also: (Woese, 1998. The universal ancestor) (Brown, 2003. Ancient horizontal gene transfer) (Jheeta 2013. Horizontal gene transfer and its part in the reorganisation of genetics during the LUCA epoch . Life (Basel))
If Mars has pre-Darwinian life, then, although cells themselves would not yet be in competition with each other, the genomes within them would be. These might evolve some degree of genomic protection, in small vesicles. As described by Koonin:
Conceivably, such primitive units of evolution could have been represented by small, virus-like replicons that populated abiogenic lipid vesicles or inorganic compartments and were subject to selection for replication efficiency.
In the course of evolution, such small replicons would accrete to form larger genomes, those that carried favorable combinations of genes attaining selective advantage. Once such growing replicons reached the level of complexity sufficient for the formation of cells, the major evolution transition to cellular life forms (reproducers sensu Maynard Smith and Szathmary) associated with viral parasites would occur - Woese's Darwinian threshold would be crossed.
( Koonin,, 2014. Carl Woese's vision of cellular evolution and the domains of life )
Exploring ways that Mars could still have early life or prebiotic chemistry
This first stage may have continued for several hundred million years of evolution, through to as late as 3.5 - 3.8 billion years ago (Doolittle, 2000, Uprooting the Tree of Life)
On this hypothesis for evolution of early life on Earth, the transition to Darwinian evolution of whole cells on Earth occurred at roughly the same time as the end of the Noachian period on Mars, right at the end of its period of most abundant water and seas.
If life on Mars evolved at a similar pace, the Noachian period on Mars might not have been long enough time for life to evolve beyond pre-LUCA replicons in vesicles in undifferentiated cells, sharing genetic material with each other readily. Then - perhaps that life could continue to the present, depending on whether the barely habitable conditions since then slow down or increase the pace of evolution.
The barely habitable conditions might favour small cells with simpler organization such as ribocells. We looked at this above in:
Though primitive, such life could still be adapted to the conditions it inhabits, with many specialist enzymes and other adaptations to help it to function in its extreme environment. Evolving through massively parallel Lamarckian evolution, the priority of all the cells would be easy and fast uptake of capabilities from its neighbours.
The evolution could just be very slow. Or maybe cells that can share capabilities with each other in such extreme environments would be favoured through to today, preventing it from taking the first steps towards Darwinian evolution that life did on Earth with more abundant resources.
Or it might be that evolution of life is rare, and not inevitable. Perhaps by chance it happened on Earth just once 4.5 billion years ago and we are all descended from that one happy accident that was never repeated which set it off on the long path to modern life. Other planets may have all the conditions for life but that chance event never happened. Or it might be that several low probability events have to happen before life can evolve and we were just lucky that they all happened early in the life of our planet
One argument in favour of a second genesis on Mars, is that we have evidence that terrestrial life started early on. It may even have evolved as early as a few hundred million years of the origins of our solar system.
Video: Diverse life forms may have evolved earlier than previously thought
Press release: (Greaves, 2022, Diverse life forms may have evolved earlier than previously thought)
Paper: (Papineau, 2022. Metabolically diverse primordial microbial communities in Earth's oldest seafloor-hydrothermal jasper)
Combining fossil and genomic information and using the concept of a molecular clock, that genomes acquire new mutations at a steady rate, the earliest life seems to date back to 4.5 billion years ago, again only a few hundred million years after the Earth itself formed. While the cells with a nucleus, Eukaryotes which most multicellular life is based on, has origins billions of years later.
Press release: University of Bristol, 2018, A timescale for the origin and evolution of all of life on Earth https://www.bristol.ac.uk/news/2018/august/history-of-life-on-earth.html
Paper: Bettset al., 2018.‘Integrated genomic and fossil evidence illuminates life’s early evolution and eukaryote origin’
It's a similar picture for the first evidence from zircons of carbon with isotope ratios that suggest life processes.
Bell et al., 2015, Potentially biogenic carbon preserved in a 4.1 billion-year-old zircon
Another researcher, Hedges commented on that result saying
“If life arose relatively quickly on Earth, then it could be common in the universe.”
(Borenstein, 2015, Hints of life on what was thought to be desolate early Earth)
If the origin of life is rare on a terrestrial planet, why would it happen so soon on Earth? It would be more likely that we would find we lived on a planet that was lifeless for billions of years before the chance events came together and the first living organisms appeared.
The eukaryotes, cells with a nucleus, did arise much later on Earth. Is that just through chance? Nick Lane uses this to argue that while simple life may be common, complex life with its ability to use energy much more efficiently in presence of oxygen may be a random rare event (Lane, 2012, Life: is it inevitable or just a fluke?).
On the other hand, it could be that the origin of life is rare, but both Mars and Earth were seeded from elsewhere .
In view of the complexity of the LCC [Last Common Community] relative to that resulting from all subsequent evolution, it is difficult to avoid the conclusion that at least as great a timespan preceded the LCC as that which followed it. If so, we must search beyond Earth for the cradle of life.
Line, 2002, The enigma of the origin of life and its timing
One possibility is life on Mars and Earth has a common origin, seeded from each other. Both could also be seeded from other stars in the birth nebula of our solar system which could exchange life readily when the stars were closer together (Valtonen et al., 2008. Natural transfer of viable microbes in space from planets in extra-solar systems to a planet in our solar system and vice versa) (Belbruno et al., 2012. Chaotic exchange of solid material between planetary systems: implications for lithopanspermia) . Life in our sun’s birth cluster could also originate in a star older than our sun, spread from cluster to cluster by life bearing stars (Adams et al., 2005. Lithopanspermia in star-forming clusters).
Sharov et al. graphed genetic complexity of non redundant nucleotides against the time of origin of an organism, and found that the complexity of the most complex organism increased at almost the same uniform rate of exponential increase of a 7.8 fold increase in complexity every billion years (log of complexity increased by 0.89 every billion years) (Sharov, 2006. Genome increase as a clock for the origin and evolution of life) .
To get this graph, Sharov et al. first had to deal with the issue of “junk DNA” as it is popularly called, DNA that doesn’t encode for proteins. For the prokaryotes, cells without a nucleus including the archaea and bacteria, there is a single genetic sequence in a closed loop and nearly all is functional. However for eukaryotes, cells with a separate nucleus enclosed in a membrane inside the cell, often most of the DNA is non coding, doesn’t make proteins. Some eukaryote microbes have more DNA than a human being ((Pray, 2008. Transposons, or jumping genes: Not junk DNA) (Elliott et al., 2015. What's in a genome? The C-value enigma and the evolution of eukaryotic genome content ). A lot of the gene sequences in eukaryotes seem to serve no function. This is the so called C Value Enigma (Nicolau et al., 2021 . The Evolutionary Volte-Face of Transposable Elements: From Harmful Jumping Genes to Major Drivers of Genetic Innovation). Measuring the DNA by functional non redundant nucleotides deals with that issue.
Projecting back, if evolution of genetic complexity continued at the same rate since the origins of life, Sharov finds that Earth life originated around ten billion years ago. If so, life on Earth could be billions of years older than our solar system (Sharov, 2006. Genome increase as a clock for the origin and evolution of life).
This diagram shows an estimate for the complexity of each type of organism when it first appears in the record. It uses the complexity of the DNA as measured using the number of functional non redundant nucleotides (Sharov, 2013. Life before earth) . This is a better measure of the genetic complexity than the total length of its DNA.
The graph is from (Sharov, 2013. Life before earth : Figure 1) with the section from the origins of Earth onwards also in (Sharov, 2006. Genome increase as a clock for the origin and evolution of life) , which also explains in detail how it was derived.
Notice that the prokaryotes; the simplest primitive cell structures we know; are well over half way in complexity between the potential earliest forms of life and ourselves.
The most complex archaea increased only 1.9 fold every billion years and the most complex Eubacteria increased only 2.5 fold every billion years (Sharov, 2006. Genome increase as a clock for the origin and evolution of life).
Both these arguments would seem to suggest we should find early life on Mars, and if so, there is a chance that it would still be there today.
However, these are just suggestive arguments and not proof. if life is rare, Mars could have prebiotic chemistry similarly to the state of Earth before life evolved, but no life, even billions of years later. Perhaps life never evolved there, just by chance because on most planets life never evolves.
It's also possible that Mars did have life but it became extinct or at least extinct on the surface if perhaps it still lives deep below the surface. If so, there might be uninhabited habitats there where the process of slow evolution of life from abiotic chemistry is once more underway. Or it may be that there is early life on Mars but it is not able to inhabit most of Mars. For instance if it lacks the ability to fix nitrogen or to do photosynthesis and has never developed hardy dormant states such as spores, it may still be present but most temporary habitats would be uninhabited. Or the very harsh surface conditions light just not make it possible even for highly developed life to spread to temporary habitats and it may only exist deep below the surface if it still survives.
So, it could be there is no life on Mars, or no life on or near the surface, or life never reached microenvironments or other habitable regions on the surface.
Value of knowing whether or not we have a treasure beyond compare of early life or prebiotic life on Mars before we make a decision as a civilization about whether and how to start the anthropocene
In all these scenarios, any martian abiotic chemistry in uninhabited habitats is clearly vulnerable to terrestrial life. Once terrestrial life grows there, the conditions will be too altered to explore the complex chemistry that was going on there before terrestrial life was introduced.
Early life might also be vulnerable on Mars, either recently evolved or that never evolved far. It also might have little by way of defences against modern Earth microbes. Simpler life must have existed on Earth in the past and no trace of it now remains. If it still exist on Mars it could go extinct rapidly when faced with the competition with modern life, due to the same processes that made it extinct on Earth, whatever they were.
We can use pre-Darwinian life as an example of how we might find harmless life on Mars which is nevertheless a treasure beyond compare for our civilization, while bearing in mind that simpler life might take many different forms.
If we find recently evolved pre-Darwinian life on Mars, or other forms of primitive early life in undifferentiated cells, we’d likely conclude early on that there is no risk to Earth’s biosphere. In some cases such as the mirror life nanobes example, it might be difficult to prove that even early life can't harm terrestrial life given that it could change how biospheres function, or produce toxins and so on. However, suppose we can show that it is completely destroyed by microbes found anywhere on Earth that it might encounter we might be confident that it is safe. We could try terrestrial microbe challenge experiments to confirm that the Martian life is completely destroyed by Earth life. If this happens we might conclude early on that it is of no risk to Earth.
We would need to make sure that there is ONLY pre-Darwinian life on Mars and it doesn't share some of the habitats with some other more advanced form of life that can spread on Earth. Supposing we are confident of all that.
In that situation we still have a decision as a civilization - is such early life of value to be preserved for the future? If so, do we need to protect the entire planet or are there other ways to preserve it?
Depending on our future plans, if the decision is made to colonize an early life or prebiotic Mars, at least we know in advance what we are doing. After making such a decision, our preliminary astrobiological survey would give us an opportunity to "rescue" early life and prebiotic chemistry on Mars, to attempt to reproduce it, perhaps in space habitats outside of Mars, or there may be things we can do on the planet to protect small microcosms of uncontaminated Mars, even if just in small-scale habitats a few meters across, before the processes are erased on the rest of the planet itself.
If we prioritize an astrobiological survey from orbit first, we may soon be able to make informed decisions about whether to send humans to the Mars surface. If the decision is then made to land astronauts on Mars, the astrobiological survey can help us to do it in a way that preserves the interest of native Martian complex chemistry, protocells, early life or complex life and is safe for our astronauts, and Earth itself.
While if we try to do this without an astrobiological survey we are flying blind. We don't know what is there or what we might do to it or it to us. If there are biological treasures there we may lose them before we know they exist.
Also, even if there is early or pre-biotic life on Mars, and we make the decision as a civilization to extinguish / destroy it at least on Mars itself, if we have this astrobiological survey first, the measurements made during this survey would remain as a record for future humanity, thousands of years into our future of what Mars was like before we initiate the anthropocene geological era on Mars with surface processes altered by terrestrial biology.
The main thing is that we would make our decisions in knowledge of what we do. We’d avoid the situation of landing there, destroying early Mars and then potentially having regret for what we destroyed, which we would know can never be restored. See Supplementary information section under:
Instead we'd be replacing pre-biotic or early life on Mars with terrestrial life in full knowledge of what we are doing and the consequences and preserving what we feel needs to be preserved for ourselves and for future generations.
This would seem to be a decision that needs a broad consensus in our civilization given the wide-ranging effects for future generations of our actions. Many may not know that there is no prospect with current technology of reversing extinction if we found a recently extinct microbial species on Mars. See Supplementary information section under:
There is no real prospect either of simulating complex chemistry that might only happen after millions of years in an uninhabited cave or surface oasis on Mars. So far none of our experiments in prebiotic chemistry in laboratories are anywhere close to reaching the point of creating a second genesis in the labs.
Also the potential value from biology or prebiotic chemistry may be far more wide-ranging than many in the public would realize. See Supplementary information section under:
In this book I suggest an extension or remastering of the precautionary principle for such potential discoveries that may be "superpositive" . Of incalculable future value to our civilization with a risk that we may lose them before we know they are there. See Supplementary information section under:
This book suggests that such a decision is something that we need to go into with eyes wide open.
We can be pretty sure there already are viable terrestrial; microbes on Mars. attached to our spacecraft and some have likely fallen into the dirt - but that's not the same as starting the anthropocene on Mars, because they also need to find habitats that they are pre-adapted to inhabit, which most likely hasn't happened yet.
Also if we find terrestrial life has started to spread irreversibly, this doesn't mean it makes no difference to introduce extra species. Cane toads have different effects on Australian wildlife from European rabbits. Suppose, say, Mars already has blue-green algae but nothing else from Earth, Some early life might be able to co-exist. Some uninhabited habitats would be underground and not accessible to photosynthetic life. We would still have a decision to consider, whether to act to stabilize the Mars anthropocene at whatever point it has reached so far or let other terrestrial microbes in to expand the anthropocene further.
The main thing here that seems important is that it should be an informed debate and an informed decision rather than a blind accidental destruction of something we might later decide was of great value before we lost it.
A mirror life planet humans can never land on in our backyard as Sagan's "treasure beyond assessing"
If we do find life on Mars based on an independent biology, it's a discovery to treasure, not a reason to despair.Carl Sagan called it a "treasure beyond assessing". Mirror life would fit this.
The existence of an independent biology on a nearby planet is a treasure beyond assessing, and the preservation of that life must, I think, supersede any other possible use of Mars.
(Allan, 2020, Happy Birthday Carl Sagan)
Space colonization enthusiasts might feel their dreams are shattered. But far from it. We can see this as an inspiration for space exploration. To have something so remarkable, in our own solar system, which we'd otherwise likely need to travel light years to find, especially independent life on a terrestrial planet (we might also find independent life in the oceans of the icy moons Europa, Enceladus, and the asteroid Ceres amongst others, but it would still be of unique interest to find independently evolved life in a terrestrial world like Earth).
We have places on Earth we can’t settle currently such as the deep ocean, even the shallow ocean is hard for us to inhabit, for now the atmosphere is hard to inhabit, we have only made a start on seasteading, and some places are well beyond current technology. We can’t explore down to the core of our planet as Jules Verne envisioned in his “Journey to the center of the Earth” (Verne, 1897, A Journey to the Center of the Earth). But we have never had a frontier due to biology.
A Mars with mirror life, perhaps, becomes a forever unattainable frontier, a world you can never actually land on, but can still explore with high fidelity telepresence. We have never had a frontier like that.
If we do find life on Mars that can never be returned safely, this may stimulate rather than discourage vigorous space exploration and settlement. The first astronauts to Mars might study the surface remotely in a spectacular orbit, a sun synchronous Molniya orbit as proposed by the Mars HERRO study. This orbit is tilted at 117 degrees and it is easy to get to as it needs less delta v than a landing on Earth’s moon, and is similar to the minimal delta v Mars capture orbit
A dual-purpose approach: whether we decide we can never land on Mars or the two biospheres are compatible, either way a base on Phobos is a natural and valuable first step
Many readers of this book are likely to be space colonization enthusiasts so it seems important to address their concerns. They have many reasons to want to send humans to Mars. Would they satisfy these objectives if we find that humans can never land on Mars?
First, as we saw, if space colonization enthusiasts are right that the two biospheres are compatible, by the time humans get to Mars they will have numerous assets on the surface of Mars already.
So the future is similar up to the point where we make the decision about whether or not it is safe to land humans on the martian surface.
The spectacular HERRO orbit skimming close to Mars twice a day at local midday for our first telerobotic explorers of Mars
Mars can be explored robotically, and then telerobotically, with humans in orbit and on its two moons. This would involve both humans and robots, each doing what it does best, in a valued partnership. The astronauts would be involved in the search for life, controlling robots directly through telepresence and haptic feedback whenever there is a need for on the spot decisions using human intelligence. The robots are our collective sense organs on other planets. Torrence V. Johnson, Galileo Chief Scientist, put it like this in the foreword to Meltzer’s “Mission to Jupiter”
“There is always a tension in the national debate about how much robotic exploration (such as Galileo) we should do versus so-called human exploration (such as Apollo). This misses the point! What we call robotic exploration is in fact human exploration. The crews sitting in the control room at Jet Propulsion Laboratory as well as everyone out there who can log on to the Internet can take a look at what’s going on. So, in effect, we are all standing on the bridge of Starship Enterprise”
(Meltzer, 2007, Mission to Jupiter: a history of the Galileo project)
Once we get humans to Mars orbit - maybe not until late 2030s or 2040s we have the spectacular HERRO orbit for them to use. The ISS orbiting Earth is very inspiring for astronauts photographing Earth from above, this would be too. It is a Mars capture orbit which is easy to get into from Earth with minimal delta v. It is tilted at 117 degrees and in that orbit under the influence of the gravity of Mars and the sun, it automatically precesses in a way that is synchronized to the sun. It comes in close by both poles twice a day and it gets closest to Mars over the equatorial regions, the sunny side of Mars on opposite sides twice a day (Oleson et al,, 2013, HERRO mission to Mars using telerobotic surface exploration from orbit) (Valinia, 2012, Low-Latency Telerobotics from Mars Orbit: The Case for Synergy Between Science and Human Exploration).
Video: One Orbit Flyby, Time 100x: Mars Molniya Orbit Telerobotic Exploration in HERRO Mission
Even when furthest from Mars, the time delay is only 147 ms or less than a sixth of a second. This lets astronauts control rovers on the surface in real time, at a reasonable speed at any position in the orbit. The close passes of Mars let the astronauts have control without any noticeable latency, for fine positioning of rovers, handling specimens, doing experiments, etc.
This video is intended to give an approximate idea of the sun precessing orbit.
Technical details for the video:
To set this up in the Orbiter simulator I used
Orbit reference MARS
Frame ref equator
Epoch Current
semimajor axis 12880
Eccentricity 0.726451
Inclination 116
LAN 70
LPe 70
eps 272
There the LAN, LPe and eps are guesses. Chosen so that it approaches on the sunny side but not sure if it approaches closest exactly at the point closest to the sun. As for the eccentricity - I adjusted that until the periapsis was the same as given in the paper. Inclination and semimajor axis just as given in the paper (Schmidt et al,, 2012, HERRO missions to Mars and Venus using telerobotic surface exploration from orbit)
Early astronaut explorers would operate surface marscopters, rovers and
other surface assets, similarly to avatars in a computer game.
They might well use immersive 3D VR headsets
with binocular vision and haptic feedback
as for the HERRO study
(Oleson et al,, 2013, HERRO mission to Mars using telerobotic surface exploration from orbit) (Valinia, 2012, Low-Latency Telerobotics from Mars Orbit: The Case for Synergy Between Science and Human Exploration) and other methods such as the omnidirectional treadmills now used to walk endlessly in any direction in computer games.
Text on graphic: 100% planetary protection both ways, even if Mars has vulnerable early life, prebiotic chemistry, or hazardous soil pathogens or mirror life.
Safe from:
- solar storms
- dust storms
- hazardous perchlorates laced dust
- leaking space-suits
- breakdown of rover
- hazards of landing and takeoffOrbital team does as much science as three teams on the surface according to HERRO analysis
All of these landers and rovers can be 100% sterile of terrestrial life using a Mars HOTTECH modeled after Venus HOTTECH using modern technology able to withstand heating for a few minutes to 300°C
First human expedition likely in Mars capture orbit, later on Phobos.
Can sterilize returned samples or return to a separate robotic life detection lab.
Main image: “Safely tucked inside orbiting habitat, space explorers use telepresence to operate machinery on Mars, even lobbing a sample of the Red Planet to the outpost for detailed study." (NASA, 2012, Telerobotics Could Help Humanity Explore Space)Inset image from (Mann, 2012. Almost Being There: Why the Future of Space Exploration Is Not What You Think
This is the basis for their reasoning that one team in orbit does as much as three teams on the surface from their powerpoint presentation (Shmidt et al., HERRO (Human Exploration using Real-time Robotic Operations): A robotically intense strategy for human exploration)
Also:
We could add to this that we could now make high resolution 3D graphics of all the sites they explore based on the video they send back to Earth, which in their plans would all be shot in binocular vision.
Also by the time humans are sent to Phobos or to the HERRO orbit, modern video would be multiple gigapixel resolution allowing geologists on Earth to use the equivalent of a hand lens to zoom in on any nearby rock feature of interest, so long as they establish the bandwidth to send all that video back to Earth.
With modern technology, especially developments in machine intelligence, the robotic avatars on the surface would also do a lot of automated exploration and in that way the teams could likely control rovers and landers on far more than three sites today, only intervening where the expertise and decision making capabilities of a human scientist is needed.
This is about the Centaur robot shown in the illustration, a humanoid torso on wheels (Mehling et al,, 2007, Centaur: NASA’s Mobile Humanoid Designed for Field Work), and a version that has jointed legs ending in wheels,
to combine the versatility of legs and wheels(Klamt et al,, 2019, Remote Mobile Manipulation with the Centauro Robot: Full-body Telepresence and Autonomous Operator Assistance).
This is what it might look like from inside the spacecraft
Text on graphic: Mirror life here (one scenario)
Can never land (at least never return) - but can explore via immersive VR experienced more clearly than if they are on the surface
Would stimulate high levels of interest in the public
In the 1960s the public quickly got bored of the Apollo missions after the flag and footsteps succeeded.
Composite of photo from the Cupola of the ISS (Coleman, 2011, Russian cosmonaut Dmitri Kondratyev (left), Expedition 27 commander; and Italian Space Agency/European Space Agency astronaut Paolo Nespoli in the Cupola, use still cameras to photograph the topography of points on Earth. Picture taken by 3rd crew member, Cady Coleman) and Hubble photo of Mars (Hubble, 2003, Photograph of Mars taken by the Hubble Space Telescope during opposition in 2003)
The HERRO study found that one team in orbit can do as much as three teams on the surface in this way (ADDCITE). Our capabilities for telerobotics are greatly increased since then so the ratio is likely greater. Astronauts in a surface habitat may not be able to travel that far from their base. Even with fast rovers, for safety reasons they may well be limited like the lunar astronauts to be close enough to walk back with the oxygen supply in a space-suit. They need to be able to walk back to the base in case of irreparable damage to the rover unless the rovers have an extraordinary level of reliability.
Then the lunar spacesuits reached end of life at 2-3 days because of the lunar dust.
Our astronauts can also explore Phobos which should have samples from throughout the history of Mars that are predicted to lie in its regolith as a result of impacts on early Mars. Deep below the surface of Phobos we are likely to find relatively unaltered traces of any life that might have existed there billions of years ago. This would complement our discoveries from astrobiological exploration of Mars itself.
According to physical modeling the surface of Phobos is likely to have accumulated 13 microns thickness of deposits from the impact that formed Gale crater and 5.5 millimeters thickness from the Hellas basin impact, with a mass of about 16.5 kilograms per square meter from Hellas basin and 13 grams per square meter from Gale crater. Zunil crater would only deposit 0.07 grams per square meter. (Ramsey et al,, 2013, Mars impact ejecta in the regolith of Phobos: Bulk concentration and distribution : Table 2). This is well beyond the 20 million years horizon of samples that left Mars in the martian meteorites that arrive on Earth today, which is important for astrobiology if there is a possibility that the samples might have contained viable life when ejected from Mars.
This depends also on how common or rare life was on Mars in the past or is at present. For instance if it never developed photosynthesis then it might be there but hard to find in any samples from impacts on Mars. See below:
There would be larger rock fragments also from the large craters, though broken up further by impact into Phobos, and some of it excavated by impacts on Phobos and then reimpacting later on.
There are two hypotheses for the origins of the martian moons (Kuramoto et al,, 2022, Martian moons exploration MMX: sample return mission to Phobos elucidating formation processes of habitable planets).
- Capture hypothesis: According to the spectroscopic classification of asteroids they would be classified as D or T-type asteroids which are volatile rich and originate near Jupiter's orbit. This suggests they could have been captured.
However it is hard for Mars to capture an asteroid except through atmospheric drag, which would normally end with the asteroid impacting on Mars, so to capture the moons it needs a thicker atmosphere which rapidly thins while it still has some captured asteroids orbiting within the outer reaches of its atmosphere that haven't yet hit Mars. .Also captured asteroids could approach at any angle to the Martian equator and it is hard to explain how the moons got into their near circular equatorial orbits as captured asteroids. - Giant impact hypothesis: It is easier to model the moons using a giant impact into Mars which then leads to a debris disk forming and then the two moons coalesce out of it similarly to the theory of the formation of Earth's moon. Phobos has a decaying orbit that will lead to it breaking up due to tidal forces, but this may be a repeating process. It might be that each time it breaks up, part of it gets slowed down by the Martian atmosphere and de-orbits and the part that is left coalesces again to form a new moon. So Phobos might have formed and re-formed multiple times in the past.
The main issue with the impact hypothesis is how to explain the spectra of the Martian moons, which so closely resembles D or T-type asteroids.
For details see: (Kuramoto et al,, 2022, Martian moons exploration MMX: sample return mission to Phobos elucidating formation processes of habitable planets).
We will know more about this when Japan returns its Phobos samples, which as we have seen CAN be done safely with no planetary protection issues for Earth's biosphere so long as they sample only the top couple of centimeters of Phobos, as they plan to do.
In both cases the moons would be of great astrobiological interest because of their value as "witness plates" for any life in the ejecta from asteroids impacting onto Mars for billions of years. If they are the result of a giant impact on Mars then they may tell us a lot about the conditions on Mars at the time of the impact. Perhaps they might have rocks that are essentially unaltered since those times except for impact fragmentation.
Our astrobiological understanding of Mars should expand rapidly in a few years once we can do this, both our understanding of past life if it existed and present day life too if there is any life on Mars today.
In this way humans and robots can work together to unravel the astrobiology of Mars in a way that avoids all possibility of such worst case consequences either for Earth, or for the astronauts themselves.
This approach also follows the recommendations for the first part of the Lockheed Martin “Stepping Stones” to Mars (Hopkins et al,, 2011, Comparison of Deimos and Phobos as destinations for human exploration, and identification of preferred landing sites) (Kwong et al., 2011, Stepping stones: exploring a series of increasingly challenging destinations on the way to Mars) and Mars Base Camp (Cichan et al,, 2017, Mars Base Camp: An Architecture for Sending Humans to Mars) studies as far as the human base camp on the moons of Mars exploring the surface via telepresence,
The astronauts in orbit might well also use two habitats tethered together slowly spinning to generate artificial gravity to simulate Mars surface gravity, or terrestrial gravity as desired. There has been almost no research on this to date but the little data we do have is promising.
Artificial gravity: promising data from Skylab and parabolic flight could be followed up by low cost small centrifuges and tether experiments in orbit with the potential to greatly expand options for space explorers and colonizers
Humans lose bone mass and get less and less healthy after months in space. It is possible to slow this down, with two hours of vigorous exercise a day. The twin study with the twin astronauts Scott and Mark Kelly found that Scott was able to retain reasonable health after 340 days in space, with two hours of exercise a day, coming to the conclusion that :(Francine et al., 2019, The NASA Twins Study: A multidimensional analysis of a year-long human spaceflight)
Given that the majority of the biological and human health variables remained stable, or returned to baseline, after a 340-day space mission, these data suggest that human health can be mostly sustained over this duration of spaceflight.
We don't yet have any data for multi-year expeditions in zero g. Also we don't know that Mars gravity would be more healthy for humans, though it seems likely with a third of the gravity of Earth.
However we might be able to counteract the harmful effects of microgravity using centrifuges or tether systems. Some people are very sensitive to spinning motions. However there is evidence that suggests this response gets switched off in zero g.
The only test of anything like artificial gravity in humans is the test of astronauts spinning in a chair on board Skylab. The astronauts showed no motion sickness symptoms up to 30 rpm which was the fastest the chair could spin in the experiment. It was a striking change.
"Under experimental conditions in the workshop the virtual failure to elicit symptoms of motion sickness in any of the five astronauts who were exposed to a stressful type of accelerative stimuli in a rotating chair (on or after mission day 8) implies that, under the stimulus conditions, susceptibility was lower aloft than on the ground, where symptoms were elicited preflight and postflight. The amount of this decrease in susceptibility could not be measured because the "ceiling" on the test (30 r/min) was so quickly reached."
(Johnston et al, 1977, Biomedical results from Skylab)
This for instance is the data for Skylab 4. The data is similar for Skylab 3.
Text on graphic: Skylab results for astronauts spinning in a chair at 30 rpm. No motion sickness in space
(Johnston et al., 1977, Biomedical results from Skylab : 85, Figure 11-1-7)
However these experiments were more to do with trying to understand space-sickness than artificial gravity. These encouraging results for the use of artificial gravity in space at least in the short term have not been followed up since then. But anecdotally astronauts find that they don't get sick when they spin fast in space, motions that would make them sick back on Earth.
Tim Peake shared a video showing how he felt no dizziness after spinning in zero g.
Video:Tim Peake's dizziness experiment
Tim Peake says his "vestibule system switches off" - but that's just an informal theory of an astronaut. We do have a more detailed hypothesis as well - the experimenters for the Skylab litter chair experiment hypothesized that the reason their subjects could tolerate back and forth spins and continuous spins without getting nauseous in space was because the otoliths aren't stimulated in the same way, because there is no gravity acting in the direction of the spin axis.
So first, background information. Our vestibular system is a system of canals in our ear that help us to tell whether we are spinning or stationary. For some reason spinning motions make many humans nauseous. Rats don't get nauseous when they spin so it is to do with human physiology.Mary Roach mentions in her book "Packing for Mars" that NASA Ames researcher Bill Toscano has a defective vestibular system. He only realised this when they put him on the spinning chair and he experienced no nauseous effects at all from the spinning (Roach, 2011, Packing for Mars, by Mars : 95). So, he at least, could spend 24/7 at 30 rpm for full gravity with no ill effects. The same is also true for some deaf people.
There are others who can tolerate high spin rates for a long period of time such as whirling dervishes (Semazen, 2007, Whirling Dervishes ceremony : 39:00 - 57:30) timed it at getting on for 40 rpm for over 18 minutes and 30 seconds., Then there are the figure skaters who can tolerate very fast spins for short periods of time in their routines, such as Natalia Kanounnikova with her world record spin of 308 rpm. (rpeters123, 2007, World Record Figure Skating Spin)
In the population as a whole, tolerance to motion sickness varies greatly James Lackner of the Ashton Graybiel Spatial Orientation Laboratory says there are three factors involved in motion sickness susceptibility - sensitivity, rate of adaptation, and how quickly the symptoms decay after they are elicited and each of these separately show order of magnitude variation with two orders of magnitude variation in how fast we recover from symptoms.
- sensitivity to stimulation (varies about 10 to 1)
- rate of adaptation to stimulation (adaptation constant), (varies about 10 to 1)
- time constant of decay of elicited symptoms (varies about 100 to 1)
He concludes:
The range of vulnerability in the normal population varies about 10,000 to 1.
(Lackner, 2014, Motion sickness: more than nausea and vomiting)
Of course we don't want our space stations to be only usable by some deaf people and others with a defective vestibular system, whirling dervishes, ice skaters and members of the public that are lucky enough to have a ten thousandth of the susceptibility of the most susceptible to motion sickness.
The vestibular system is not the whole story though. If it was, conditions while spinning in space would be identical to those on the Earth. But to complicate things we also have the otoliths. The otoliths are small particles of calcium carbonate in the viscous fluid of the inner ear. They sense linear accelerations, as distinct from the vestibular system which senses spinning motions.
The otoliths detect gravity and so help us to sense our orientation in space (Smith, 2019, The Growing Evidence for the Importance of the Otoliths in Spatial Memory)
So technically, no, an astronaut's vestibular system doesn't "switch off" in orbit. Tim Peake should be able to still sense whether he is spinning, but for some reason he doesn't get sick. That seems to be because of the otoliths. The Skylab researchers were reasonably certain of this.
"The rotating litter chair was used in the stationary as well as the rotating mode. In the stationary mode when head movements were executed aloft, the canals were stimulated in the same way as on the ground, but the otolith organs were stimulated in an abnormal manner because the impulse linear accelerations generated were not combined with a gravity vector as they would have been on the ground. These impulse linear accelerations were transient but well above threshold for stimulation of the otolith receptors. When the rotating litter chair was rotating, the intensity of the stimuli generated by head movement was a function of the rotational velocity, and although the angular and crosscoupled angular accelerations stimulating the semicircular canals aloft were the same as on the ground, the impulse and Coriolis accelerative forces generated aloft were not combined with a gravitational vector. These forces, nevertheless, were substantial at all levels of angular velocity used, and at 30 r/min the centripetal force was, respectively, 0.3 g and 0.6 g at radii of 1 and 2 feet."
(Johnston et al., 1977, Biomedical results from Skylab : 78)
So, the canals were stimulated exactly as on the ground but the otoliths were stimulated in an abnormal manner in zero g. The main difference in space is that there was no gravity acting along the spin axis towards what on Earth would be the ground - this is what they mean by the "gravitational vector"
… "Loss of the g-load would affect the "modulating influence" of the otolithic system. If the otolithic influence was inhibitory the responses elicited by stimulation of the canals are said to be "exaggerated" (ref. 30). The observations bearing on this point in parabolic flight, however, indicated reduced responses to canalicular stimulation (refs. 31, 32, 33) during the weight-less phase."
(Johnston et al., 1977, Biomedical results from Skylab : 86)
So - the "moderating influence" of the otolithic system is different in zero g because of the abnormal way it is stimulated. If in normal use the otolithic system had a "modulating influence" then you'd expect this to make things worse. But instead it seems that the effect of disengaging the otolith in this way actually lead to much less motion sickness while spinning, not more of it.
… "The difference in susceptibility between workshop and terrestrial conditions is readily traced to gravireceptors (mainly in the otolith organs; touch, pressure, and kinesthetic receptor systems possibly contributing) for the reason that stimulation of the canals was the same aloft as on the ground, and visual inputs were always excluded. If it is assumed that the otolith system is responsible, then the absence of stimulation to the otolithic receptors due to gravity must have a greater influence (tending to reduce the vestibular disturbance) than the disturbing influences of the transient centrifugal linear and Coriolis accelerations generated when head and trunk movements were executed in the rotating litter chair. Although these transient accelerative forces, as pointed out in the section on Procedure, are substantial their effectiveness as stimuli are virtually unknown. The otolithic zonal membrane has considerable mass, and transient accelerations lasting fractions of a second might have little or no effect. The absence of gravity, causing what has been termed "physiological deafferentation" of the otolith receptor system, would be expected to reduce not only the indirect modulating influence of the otolithic system on the canalicular system but also its opportunity to interact directly with this system"
(Johnston et al., 1977, Biomedical results from Skylab : 88)
Their reasoning here is that because the stimulation of the canals is the same in space and on the ground, by a process of elimination, it is the difference in stimulation of the otoliths that made the astronauts less susceptible to motion sickness. The membrane around the otolith has a lot of mass which reduces the effect of sudden short accelerations. Because of the absence of gravity, which disables most of the influence of the otolith system, what they call "physiological deafferentation", then the otoliths not only have less effect on the vestibular canals, they also are less able to interact with them.
Interestingly the lack of susceptibility to nausea actually persisted for a day or two after the flight.
Postflight, the Commander was symptom free on the day after recovery when he executed head movements with the rotating litter chair stationary and on the second day postflight when it was rotating clock-wise at 15 r/min. On the fifth day postflight an endpoint was reached that approximated his pre-flight susceptibility level."
(Johnston et al., 1977, Biomedical results from Skylab : 84)
So Tim Peake was right in a way but it is not his entire vestibular system that switches off. He doesn't lose his ability to detect spin motions. Rather at least based on the very limited data we have so far, it see,s that it's his otoliths that switch off, his graviceptors, which help to detect linear acceleration and his orientation in a gravitational field.
These experiments with Skylab were then followed up by experiments in parabolic flight. Even during brief periods of zero g, motion sickness is almost completely eliminated.
Blindfolded subjects rotating at constant velocity made head movements while exposed to weightlessness (0 g), to straight and level flight, and to 2 g background force levels, where g = 9.8 m/s2, the acceleration of Earth gravity (see Fig. 4). The results were unequivocal (Lackner and Graybiel 1984a, 1986a). Immediately on transition into 0 g, head movements during rotation were less provocative and less nauseogenic than head movements made in straight and level flight. By contrast, head movements in 2 g were much more provocative than in level flight, and most subjects could only make a few before becoming nauseated to the point of vomiting. Moreover, being in weightlessness (“0 g”) eliminated the disorienting effects of the head movements; the head movements felt nearly normal and were no more provocative than a head movement made in 0 g while not rotating
(Lackner, 2014, Motion sickness: more than nausea and vomiting)
When we spin and then stop suddenly we may get vertigo. The world seems to continue to spin or we seem to spin. When this happens we also have involuntary movements of the eyes, nystagmus. They gradually drift to one side then jerk back repeatedly (Carle Health, n.d., Nystagmus). This symptom is easy to detect experimentally
Researchers found subjects in zero g had far less nystagmus when the spinning is suddenly stopped or started. They also had far less nystagmus in zero g for the coriolis effect which leads you to experience unexpected sideways pushes when moving vertically in artificial gravity)(Lackner, 2014, Motion sickness: more than nausea and vomiting).
Looking more closely at why this happens, the researchers found out that we lose our ability to integrate velocity. When volunteers are set spinning while blindfold, and then stopped spinning, normally in full g or hypergravity they can indicate with high precision how far they turned before they were stopped. When they are turned in zero g, they detect an initial displacement but then they detect no more changes in position of their body (Lackner, 2014, Motion sickness: more than nausea and vomiting).
Text on graphic: When blindfold in zero g we can’t tell how far we turned and so don’t feel nausea.
Uses joystick to show how far they think they have been turned around.
Detects only 20° of turn instead of 120° for the same rotation in 0 g and only hen the spin starts or stops.
[Added the dotted green lines to match the description in the accompanying text that they only detected position changes at the start and end of the movement. ]
(Lackner, 2014, Motion sickness: more than nausea and vomiting : Fig. 5).
So it seems that when spinning in zero g we lose the ability to track our position in space. The green line may be a little confusing in that figure as it seems that the subjects only were able to detect the change in spin at the start and at the end of the movement. I have added two horizontal dotted lines to convey what the paper says in the accompanying text:
Figure 5 shows that in 1 g, the blindfolded subjects were accurate in indicating their angular displacement. By contrast, in 0 g, they made a slight initial joystick movement in the direction of the turn, but then kept it aligned with their body axis because they did not feel any spatial displacement, just a slight initial tug in the direction they had actually been turned.
(Lackner, 2014, Motion sickness: more than nausea and vomiting : Fig. 5).
This conclusion that we can't do position integration when spinning in space blindfold simultaneously resolves many observations that were puzzling before.
These results mean that in a weightless environment, the signals from the semicircular canals are not being integrated by the central nervous system to give rise to a sense of body spatial displacement. It also explains why the Skylab astronauts were not susceptible to Coriolis cross-coupling stimulation in-flight. The signals that give rise to spatial displacement and lead to disorientation were not generated. It also explains why astronauts sometimes lose track of their orientation in space vehicles and may fail to recognize their spatial location when they make body turns
(Lackner, 2014, Motion sickness: more than nausea and vomiting : Fig. 5).
It seems likely that astronauts living in a constant artificial gravity field with no gravity along the spin axis, spinning for artificial gravity wouldn't feel like they are changing position. Although we can't test this until we do the experiment in space, it seems likely that if astronauts were spinning for artificial gravity, if they looked out of the window it might seem like the stars are moving, and that they are stationary and it might be a powerful illusion of an actually spinning universe. Not in the sense of vertigo. Not a dizzy sensation. Just a feeling of being motionless with the universe itself really spinning around you. It seems likely that if you close your eyes or look only at the habitat walls spinning along with you, you won’t feel that you are spinning.
The nausea seems to be associated with feeling that your body is changing position constantly. If these conclusions are correct, there may be no nausea even for the most susceptible people and for long term exposure to spin motions so long as there is zero gravity along the spin axis. It would be interesting to test to see if there is any threshold effect in low gravity conditions. Would we detect changes in orientation as easily on the moon in lunar gravity as in full gravity? Or would it be more like the zero gravity case? Or something between the two. Is the gravity on Phobos enough to let us detect any changes in orientation?
So that leads to various options. They seem to stay switched off for several days after an astronaut returns to Earth. Which would seem to suggest an astronaut could withstand several days of artificial gravity in space at the end of a mission before they get queasy.
How much zero gravity do they need to disable the otoliths? How much do they need a day to keep them disabled? Will a few minutes or a few hours of AG a day re-enable them?
Also, even when living 24/7 in artificial gravity the otoliths would be stimulated - but in a different way because there is no gravity along the spin axis. Would they still be perceived as conflicting with the vestibular sensors if the otoliths only experience linear acceleration radially and not along the spin axis? It is at least a different combination of signals.
Also if an astronaut is constantly spinning in order to generate AG, during that time, the vestibular sensors are constantly stimulated. So, would they switch off in some way? Or be less active? What effect does this have?
We can't test this on Earth because the test centrifuges spin horizontally and so they have an extra force of Earth's gravity along the spin axis.
The Skylab astronauts were able to run around inside their large circular habitat with no apparent ill effects.
Video:Jogging on the walls of SkylabAlthough this features often in science fiction, it's never been tested at all in space. It might help to use a centrifuge in orbit around Mars and indeed during the journey to Mars, like the one in 2001 a Space Odyssey. Notice that for someone who stands still inside the centrifuge, they will have no visual sensation of motion. This of course is a film set, they actually did it by counter-rotating the drum as the actor ran so the actor was essentially running on the spot like someone on a backwards moving treadmill. But visually it would be no different from the experience of the actor.
Video: Centripetal Motion - 2001 A Space Odyssey]As the video demonstrates, astronauts would experience artificial gravity outwards from the hub, the center of the spin.
If humans can stay healthy long term in space using artificial gravity this leads to many more opportunities for exploration in the solar system as it would mean we can do multi-year journeys with no loss of health. It would also eliminate the need for a two hour workout every day on a long journey to reduce how fast their health deteriorates in space.
For the background see (Hall, 1994, From Sputnik to Skylab)
The Skylab data seems to be all we have by way of data from microgravity conditions on the topic of how humans might respond to the effects of artificial gravity spin in space.
The focus of current research in the ISS is on the effects of zero g. At one point NASA proposed to add an exercise bicycle to the ISS that would have generated its own artificial gravity. However it was never flown
Sketch of the AGREE centrifuge for the ISS. From (Trigg, 2013, Design and Validation of a Compact Radius Centrifuge Artificial Gravity Test Platform. : 15).
The plan was to replace the four racks at the end of the Permanent Multipurpose Module. Astronauts cycle in a seated position, an exercise that is excellent for health and suitable for an extremely compact radius centrifuge. Chris Trigg concludes:
"Given the compact design, subject positioning, available sensors, tested accuracies, and validated operations, the MIT Compact Radius Centrifuge represents one of the most unique yet realistic centrifuges currently in available for artificial gravity research. It is hoped that through these future studies the MIT CRC will provide a better understanding of the effects and capabilities of an inflight-centrifuge, and perhaps contribute in some small way to progressing towards the inevitable trip to Mars. "
It would be possible to do preliminary tests on the feasibility of small radius artificial gravity by getting astronauts to spin each other around for minutes or hours as for Tim Peake and measure what happens to them as for the Skylab experiments.
Or we can devise some light weight experimental apparatus designed to do that in a more controllable way. These are the spin rates and velocities we need to achieve:
- 0.5 meters: 40 rpm, 5 miles per hour (8 kilometers per hour)
[controlled version of Tim Peake spinning] - 1 meter: 30 rpm, 7 miles per hour (11.3 kilometers per hour)
- 2 meters: 21 rpm, 9.9 miles per hour (a little under 16 kilometers per hour)
[if interior racks of the ISS are removed as for the exercise bike]
For these figures see Theodore Hall's SpinCalc (Hall, 2000, SpinCalc from his artificial gravity page). These are relatively slow speeds. Slower than touring speeds for even beginner cyclists who typically achieve 12 miles per hour or more for a short distance of 10 to 15 miles (Road bike, n.d. Average cycling speed for new and experienced cyclists).
There is too little data from the Apollo astronauts to be confident yet of the health effects of lunar gravity. If lunar gravity is enough for human health, we can more than halve these figures (artificial gravity depends on the square of the speed and spin-rate)
- 0.5 meters: 17.2 rpm, 2 miles per hour (3.2 kilometers per hour)
- 1 meter: 12 rpm, 2.85 miles per hour (4.6 kilometers per hour)
- 2 meters: 8.6 rpm, 4 miles per hour (6.5 kilometers per hour)
This suggests that an apparatus resembling a spinning hammock might work. This could be very light weight and replace the same four racks as the exercise bike.
Sketch to show the two possible orientations of the hammock
Text on graphic: Hammock suspended from two pivots with motors to spin for artificial gravity.
More controlled version of Tim Peake spinning. Two possible orientations of the hammock. Motor, rigid rods
Needs a synchronized flywheel (e.g. on floor) spinning in opposite direction to counteract angular momentum effects on ISS
Need to remove some equipment racks to make space for it.
A hammock is low mass but can easily withstand full Earth gravity
Background graphic: (Micky, 2006, Lonely hammock) , ,
This hammock may seem flimsy compared to terrestrial centrifuges, but there is no gravity acting along the axis, so the rods only need to be able to hold the spinning astronaut under tension, similarly to a stationary hammock.
Let's join the hammock to the pivot motors with stiff rods rather than ropes or cables, to make it easier for the motors to spin it around. They could be telescoping rods that fold up. The hammock can be designed so it's easy to take it down or fold it to one side out of the way.
In this suggestion, the hammock and the rods use a strong lightweight material to save on weight, perhaps carbon fiber maybe reinforced with kevlar for additional strength. They could be telescoping carbon fiber rods with kevlar threaded through the poles for additional tension strength as a safety measure. The motors can be small and don't need much power. It's like spinning a cycle wheel, it's easy to spin up, and once set going it keeps going by itself.
Astronauts will need a way to stop the spin quickly to respond to an emergency. This needs to be robust and failsafe. Perhaps if you put your hand out and touch a wall, say, the end wall of the module, if any resistance is detected, it would stop you spinning immediately or you press an emergency stop button and it then presses brushes against the base of the hammock as it spins past to stop it. In the other direction, if someone pushes you to set it spinning or you push against a wall, the motor kicks in to keep you spinning at whatever speed you are going. making it effectively "frictionless".
Or it could be a purely mechanical system. The astronauts could install a circular track on the end wall of the module or around the outside of the module. This could be made of light-weight plastic segments slotted together similar to a toy train track. The astronauts pull on a lever which presses a brake-pad or a brush into the track to stop the hammock. This works similarly to the brakes on a cycle wheel.
However this is done the spinning hammock could stop very quickly. At its slow speeds it could stop faster than a bicycle can stop with no significant discomfort for the astronaut.
It would also have a pad to dial in a desired spin rate, from quarter of lunar or less all the way up to full gravity. Full terrestrial gravity would be the maximum, unless there is a reason to look into hypergravity effects in space, for instance, to test spin tolerance for astronauts in an emergency situation.
There are two possible orientations as shown in the sketch
- parallel to the axis:
: triggers a spinning sensation in the horizontal canals of the astronaut's ears. - at right angles to the axis, still parallel to the floor and ceiling, with head following feet (or feet following head) around the axis:
triggers a tumbling sensation, with vertical canals stimulated.
The spinning sensation parallel to the axis is closer to the sensation when sitting or standing up in a larger centrifuge. It's best to permit both arrangements. Humans might be able to tolerate one direction better than the other. Also there could be individual variation or preference here.
If you used such an apparatus, you'd be able to sit up, e.g. to eat a meal, or read. You could do exercises also, e.g. sit ups, and bench presses. You could stand up as well even in the 1 meter radius centrifuge that can fit in without removing the racks, but because it is just 2 meters in diameter, your head would experience gravity in the opposite direction similarly to Tim Peake's experiment. With the 2 meters diameter centrifuge your head would be close to zero artificial gravity.
In the sketch I suggest a spinning flywheel in the floor to compensate for the spinning motion. A space station like the ISS has stabilizers anyway, to keep it oriented in space, so this may not be needed, however it would reduce the demands on those stabilizers. The flywheel only needs to change angular momentum when the spin rate changes. Without it, as the hammock starts to spin this will cause the ISS to start a much slower tumble in the opposite direction. When the spin stops, the ISS also stops its tumble in its new orientation whatever it is.
The ISS is much heavier of course, with a mass of about 420 metric tons, and over 50 meters in length. Just one ton at a distance of 25 meters has getting on for 9000 times the moment of inertia of a human with weight of 70 kg at 1 meter. I'm not sure what the moment of inertia of the ISS would be, it depends on the distribution of the mass, but probably at least a hundred thousand times that of an astronaut in the hammock.
Nevertheless, that means that if you spin around in the centrifuge perhaps a hundred thousand times or so, the ISS would spin around once. At 30 rpm, that means the ISS would turn around perhaps once every day or two. When the astronaut stops spinning the ISS would stay in its new position.
That might not be much of an issue however, because the attitude of the ISS is controlled by its Control Moment Gyroscopes (Roithmayr, 2003, Dynamics and Control of Attitude, Power, and Momentum for a Spacecraft Using Flywheels and Control Moment Gyroscopes : 2)
Text on graphic: One of the Control Moment Gyroscopes in the ISS. These can likely compensate for the change of momentum when the hammock starts or stops spinning.
(NASA, 1998, Boeing technicians remove the cover from a Control Moment Gyroscope (CMG) in the Space Station Processing Facility at KSC)
It's also kept in position by the gravity gradient of the Earth's gravitational field.
If necessary, then we need to add a counter rotating flywheel to the design. This would be enclosed of course, and spinning far faster than the astronaut - perhaps attached to the ceiling or the floor around the motors (or both). If we don't need this in the ISS it might be a good feature in a smaller spacecraft.
We could do this experiment at any time but a good time to do it might be when the ISS is decommissioned. NASA plan to decommission the ISS at about the same time it starts to construct the Lunar Gateway in orbit around the Moon. NASA plans to transition to using commercial space stations in Low Earth Orbit for crew training. It plans to operate the ISS through to 2030 and then de-orbit it at some time after 2031 (NASA, 2023, Frequently asked questions about the International Space Station transition plan). It hopes to start using commercial space stations as soon as 2028.
In January 2020, NASA awarded Axiom Space a contract to attach commercial modules to the International Space Station. The modules are designed to eventually detach into a separate space station. Axiom is currently planning to launch their first segment in 2026.
A year later [i.e. 2021], NASA signed agreements with three groups of companies who plan to develop freestanding commercial space stations. After one team joined forces with another group, there are two station concepts in the works: Orbital Reef, which aims to be operational in 2027, and Starlab, which is targeting operations for 2028.
(Davis, 2023, How NASA plans to deorbit the International Space Stationk)
Though it may be delayed as that article goes on to say as it is dependent on the timetable for commercial space, if that's delayed NASA may keep the ISS for longer.
The de-orbit will take several years with visiting spacecraft to do the de-orbit and everything inside is going to be destroyed during the controlled re-entry and disposal at point NEMO in the Pacific. Commercial space weren't interested in buying the components and it would be impractical to move the ISS up to a high enough orbit to preserve it indefinitely.
After that NASA expect to have at least two crew using commercial facilities at any time
"We estimate that our agency’s future needs in low-Earth orbit will require accommodation and training for at least two crew members continuously,"
(Pearson, 2022, How the ISS is being retired, and what will happen to it)
So this is a good opportunity to make use of the interior of the ISS before it is burnt up in the atmosphere. NASA could remove some of the racks to make space for artificial gravity experiments, before decommissioning the life support. Since everything inside is going to be de-orbited and burn up in the atmosphere, it wouldn't make much difference if some of the racks are removed earlier to make room for artificial gravity experiments.
Alternatively, commercial space might do the experiments during that transition period. Future tourist hotels in space are likely to explore artificial gravity in some form as it has many potential advantages for the tourist visitors, even if humans can tolerate it in space only for a short time.
- an AG toilet would greatly add to comfort if humans can withstand spinning for a few minutes without disorientation.
- a circular dining room that spins while you eat would make a big difference to passenger comfort. This assumes that spinning in space doesn't trigger any nausea in vulnerable people, as the experiments so far suggest.
- AG for a few minutes while exercising could also help a lot with maintaining health.
- We may be able to withstand spinning for hours at night as we lie down asleep
- We may be able to withstand spinning in a small centrifuge in space all the time.
NASA and commercial space could also work together on collaborations. Perhaps private space could sponsor installation of a temporary hammock or lightweight centrifuge in the ISS to test ideas for use for construction in their space stations, during the decommission phase of the ISS. Axiom Space for instance could then do the tests using private astronauts when they dock with the ISS during the decommissioning (Davis, 2023, How NASA plans to deorbit the International Space Stationk).
NASA or commercial space could also perhaps sponsor tether based explorations of artificial gravity, Joe Carroll's idea which we'll come to in a moment.
It is clear from what we know so far that we'd be able to tolerate spinning for short periods of time minutes at least in a small centrifuge in space. The main question is whether we can tolerate spinning for hours on end in a small centrifuge.
If we can only withstand a few minutes in a small centrifuge in space we can explore larger tether based systems for longer term artificial gravity.
Even if most people can only tolerate short term artificial gravity for minutes or tens of minutes, the very small centrifuge systems would have still have great advantages for pioneer exploration of the more distant parts of the solar system.
We could se a future where long distance pioneering exploration is done by people with defective vestibular systems, and perhaps also by ice skaters, and whirling dervishes because of their past training to tolerate spinning motion, or others who train to become tolerant of spinning motion, and people who by chance have far less sensitive or defective vestibular system. Such people could use small light-wait pioneer ships at far less cost than those who require large centrifuges. This could let them keep healthy for years on end in space with much lower cost missions.
However there seems to be potential that everyone may be able to tolerate small scale artificial gravity for long enough for some activities perhaps even with short tethers or a small centrifuge. This seems to be a topic that is worth testing in space so we can design our future space missions such as the Lunar Gateway based on knowledge, rather than knowledge gaps, about the potential for artificial gravity for humans in space.
Joe Carroll has a paper that looks into these tether based experiments. A larger tether based system would have a hub like the hub of the centrifuge in 2001 a space odyssey which is where visiting spacecraft can dock, with a counterrotating docking port, and then the tether itself can be an airbeam with a lift in it for easy communications between habitats at either end of the tether. (Carrol, 2010, Design Concepts for a Manned Artificial Gravity Research Facility). He also found that it is possible for a spacecraft traveling to Mars to continue spinning for artificial gravity through course correction maneuvers.
He suggested testing human tolerance to these spinning motions on the way to the ISS. His ingenious experiment involves using the excess fuel a spacecraft always has when it reaches orbit. as a safety measure.
Text on graphic: Joe Carroll's ingenious tether experiment
Final stage, Dragon Capsule
They remain tethered and fuel used to spin up - then the tether is released and the released delta v boosts the capsule to the iSS and the final stage to a controlled re-entry in the Pacific.
Graphic is a screenshot from the Orbiter spaceflight simulator(Schweiger, n.d., Orbiter Space Flight Simulator 2016 Edition)
The spacecraft remains tethered to the final stage and in a series of timed boosts it increases the perigee of the orbit (most distant point from Earth) and at the same time sets the spacecraft and the counterweight of the final stage spinning around their center of gravity to test artificial gravity. Then when the experiment is over, the tether is released at the perigee. The delta v released simultaneously boosts the spacecraft to the ISS and the final stage to a controlled re-entry in the Pacific.
Text on graphic: Tether release happens here:
All the boosts happen here:
Graphic is a screenshot from the Orbiter spaceflight simulator(Schweiger, n.d., Orbiter Space Flight Simulator 2016 Edition)
Because this experiments uses excess fuel the spacecraft can still reach the ISS even if the tether breaks at the worst possible moment.
NASA and partners are in the process of designing a new space station from scratch, the Lunar Gateway. This is an opportunity to build in small centrifuge artificial gravity to make it far more habitable for the astronauts. For eating, sleeping, toilets, physical exercise and their general health. It would also mean they have more time in the day for other activities not required to spend 2 hours a day physical exercise to avoid the deterioration of health due to zero gravity.
Text on graphic: First complements may be launched around 2026.
If tests of small centrifuge Artificial Gravity support the Skylab results, NSA and partners can integrate it into the new Lunar Gateway to make it far more habitable.
Graphic from: (NASA, 2023, Their Paths to Gateway)
If we can stay healthy indefinitely in zero g using small diameter centrifuges, we can have far easier human exploration of small moons, the asteroid belt and so on. If we need slower spin rates we can still spend part of the day in trains driving around circular race tracks built on the surface of an asteroid or the Moon to augment gravity, For instance explorers or settlers could sleep in such tracks, or use them for exercise during the day.
"One method of augmenting gravity is a extraterrestrial railroad. A vehicle on a circular track banked with respect to the horizon creates centripetal accelerations related to the speed of the vehicle and the diameter of the track. Incremental accentuation of gravity may be accomplished by switching the vehicle to a track of larger diameter and steeper bank. Rotation creates accelerations on the vestibular canals of the inner ear that will limit the angular velocity of the vehicle. Colonists would have the opportunity to work part of each day in simulated Earth gravity and easily access the planet's surface. The magnitude of gravity that will protect us is unknown, as is the frequency and duration of exposure. This must be investigated. An extraterrestrial railroad, as one solution to this problem, does not involve exotic technology and is readily expanded."
(Schultheis, 2000, Artificial Gravity Augmentation on the Moon and Mars)
The large tether systems would scale up eventually to the Stanford torus. We have potential to use materials in the asteroid belt for habitats. The main limitation is the amount of material needed for radiation shielding and there is enough mass in the asteroid belt for a population of trillions with 1000 times the land area of Earth.
The first answers they came up with indicated there was more than a thousand times the land area of Earth as the potential room for expansion. They concluded that the surface of a planet was not the best place for a technical civilization. The best places looked like new, artificial bodies in space, or inside-out planets.
...
The idea of using a planet to provide gravity and to hold an atmosphere really represents the hard way to go about doing these things. Really tremendous amounts of material must be collected, enough to make a planet 5000 miles in diameter, before there is enough gravity to hold down an atmosphere and keep it from leaking into space. Even Mars isn’t quite big enough—its atmosphere has almost entirely leaked away. Ever since Wernher von Braun published his space-station articles in Collier’s over twenty years ago, people have been aware that a few tons of metal will suffice to build such an inside-out world, to give gravity and an atmosphere.
Also space is not an empty, hostile environment. It is a culture medium, rich in energy and in the resources needed for life. An artificial world in space gets solar energy full time, without the day-night cycles and the atmospheric absorption of a planet. Further, planets have strong gravity fields against which a spacecraft must fight. The earth’s gravity is strong enough to have the same effect as a hole, 4000 miles deep, out of which we must climb. If we wish to colonize the surface of another planet, we are just climbing up a deep hole, passing through the sunshine of space—and then going down another hole.
(Heppenheimer, 2007, Colonies in Space: Chapter 2 – Our Life in Space)
These habitats can also simulate any climate on Earth and also Mars gravity lunar gravity.
As Don Fuqua said when he chaired a subcommittee of the House committee on science and technology that looked at O'Neil's findings on artificial gravity in 1975 and allocated extra funding to NASA for research “to lay the foundation for advanced projects, such as moon bases and orbital colonies.”:
“It is hard to predict tomorrow, and although I do not have the vision to say precisely where the future will take us, I do know that our space program is only in its infancy stage.”
(Heppenheimer, 2007, Colonies in Space: Chapter 2 – Our Life in Space)
So artificial gravity research can make it easier for humans to explore space, to travel to Mars and to live in orbit around Mars and on the martian moons. It could also make it easier to live on the surface of Mars too if Mars gravity isn't sufficient for human health and needs to be augmented, and it can be used to experiment closer to home, in Earth orbit, to see how well humans are able to adapt to martian gravity.
Small or larger habitats spinning for artificial gravity could also simulate Mars surface conditions to test ideas for terraforming Mars, testing the sequence of beneficial microbes to introduce for the best way to make it more habitable for humans, or to explore ideas for restoration ecology, make it more habitable for native life if instead we aim for ecopoesis.
This could start with a small facility to simulate Martian gravity similarly to the centrifuge in the proposed miniature life detection lab, but somewhat larger simulating various surface processes in a highly realistic Mars surface simulation, then scale to larger experimental Mars simulation habitats until experimenters are confident their ideas would work on Mars, if they do reach that point.
Such a chamber could also be used to simulate conditions in the martian dust storms, or for the martian geysers and other surface processes, of course somewhat affected by coriolis effects which would need to be allowed for.
Phobos may have abundant ice and has mass for radiation shielding of habitats spinning for artificial gravity with land area of Texas, California, Montana, New Mexico, and Arizona
Mars' innermost moon Phobos may have ice in it. One recent study found it matched a model of 11% ice content for a 5 kilometer deep outer layer at 1.6 tons per cubic meter and 3% ice in a denser core 8 km in diameter at 2.8 tons per cubic meter. (Zhong et al. 2023, The Mean Moment of Inertia for Irregularly Shaped Phobos and Its Application to the Constraint for the Two-Layer Interior Structure for the Martian Moon). There are hydrated minerals on the surface though reduced. This doesn't necessarily mean dehydration, one possibility is that the surfaces are covered in a darker material that masks the hydration signature of both moons (Poggiali et al., 2020, Phobos and Deimos surface composition: search for spectroscopic analogues : 473)
If it is appropriate to use the mass of Phobos to turn it into habitats, assuming radiation shielding of 4.5 tons per square meter[CITE], there is enough mass for a habitable area of (10.6 × 1012 / (4.5 × 106) km2 or 2.35 million square kilometers. That is more than the combined area of Texas, California, Montana, New Mexico, and Arizona of 2110611 km2, the top five states by area excluding Alaska (US Census, 2010, State Area Measurements and Internal Point Coordinates). In terms of Europe it's larger than the area of France, Spain, Sweden, Norway, Germany and Finland, of 2193162 km2, the top six European countries by area excluding Russia and Ukraine. Perhaps an argument in favour of converting Phobos into habitats is that Phobos is spiraling inwards because it orbits faster than the Martian rotation period and will eventually crash on Mars, though only in the distant future.
Text on graphic: If it is appropriate we can use the material in Phobos for shielding of habitats with living area equivalent to:
Texas, California, Montana, New Mexico, and Arizona
or:
France, Spain, Sweden, Norway, Germany and Finland
It may also have 11% ice in the outer 5 kilometers (with a denser 8 km diameter core with 3% ice).
(NASA, 2008, Phobos from a distance of 6,800 kilometers)
This is based on figures for the mass of Phobos of 10.6 trillion tons. Deimos adds another 1.5 trillion tons:
- Phobos 10.6 × 1015 kg (ESA, n.d., Phobos) or 10.6 trillion tons.
- Deimos 1.5 × 1015 kg *ESA, n.d., Deimos) or 1.5 trillion tons.
Deimos may have ice too. David Kuck has proposed mining ice from Phobos to export to Earth orbit, as it requires less delta v to do that than from the Moon. Also every two years it requires less delta v to get to Deimos than to get to the Moon (Kuck, 1997, The Deimos water company). This could also be used to export ice to Earth orbit from Near Earth Asteroids some of which have very low delta v.
Asteroid belt materials can form the living area of a thousand new worlds as habitat bubbles if humans can't land on Mars
If we proceed like this, if we don't attempt to terraform Mars or Venus, the other two terrestrial planets in our solar system within the "Goldilocks zone" of our sun, what happens to hopes to explore, and find new homes in the galaxy? We can use habitat bubbles instead. These can orbit Mars, Venus, Earth, or indeed similar habitats can be used to colonize anywhere in the solar system right out to Pluto and beyond.
Though these are major projects, they can be completed far faster and with far less uncertainty about the eventual outcome than a project to terraform an entire planet. We can also do smaller ones first, then build up to larger ones. They could lead to terraforming later building on the experiences we gain from addressing the challenges of maintaining a self sustaining ecosystem inside space habitats.
We'd build up gradually from the smaller habitats that consist of just a spacecraft using its final stage as a counterweight, or two spacecraft connected together by a tether airbeam to larger habitats like the Stanford Torus which was a fully worked out proposal from the 1970s..
These ideas have been refined and improved on in many ways since then. One idea for a smaller space settlement is Kalpana one, which is multi-layer and uses the inner layers for lower gravity levels of the settlement. (NSS, n.d., Kalpana One Space Settle men)Text on graphic: The original Stanford torus design. Waste heat radiator, Habitat spinning at 1 rpm, Mirror to reflect sunlight into the habitat, Another habitat
Artwork by Don Davis. (NSS, n.d., Stanford Torus Space Settlement)
Asimov talked about fixation on planetary settlement as “planetary chauvinism”, a phrase he got from Carl Sagan (Asimov, 1982, interviewed by Bill Boggs, 35 minutes into this video). The asteroid belt between Earth and Mars has enough materials for a thousand times the land area of Earth reformed into large habitats, slowly revolving for artificial gravity (Heppenheimer, 1977. Colonies in space : Chapter 2 – Our Life in Space).
We can build these habitats in a decade rather than the millennia for terraforming. If large enough, they may be almost self sustaining, with the help of some technology, like a planetary biosphere in miniature. In the process we build up experience useful if we attempt to modify planets later.
It wouldn't add significantly to the area to add the intertidal zone, estuaries, coral reefs, kelp forest, seagrasses, mangrove swamps and other coastal and shallow sea areas of a thousand Earths. There is water in the asteroid belt we now know. Indeed it's possible Ceres has more water than Earth does (NASA, n.d., Ceres)
We would likely prefer to keep Ceres intact as it is. However, as for Phobos, there is likely to be enough water for the early stages of asteroid development in other asteroids including possibly large amounts from the ones that are extinct comets.
Then, in the not very distant future, prospective asteroid belt settlers wouldn't limited to the materials in the asteroid belt. The "Interplanetary Superhighway" allows slow transfer but almost with no fuel all the way from Neptune to the asteroid belt.
In this graphic the green curves are energy free transitions from the L1 gravitational point of balance between the planet and the sun inwards to orbits closer to the sun and the black curves are transitions from the L2 gravitational point of balance where the sun and the planet's gravity can keep an object in unstable equilibrium on the far side of the planet from the sun. It's possible to transfer between L1 and L2 and back with almost no fuel and where the green lines from the L1 curves cross the black lines of the L2 curves there is zero energy transfer from one to the other.
Text on graphic: Transitions shown can be made with almost zero delta v
(Lo, 2002, "The interplanetary superhighway and the origins program.: Figure 11)
In this way materials from as far as Neptune's L2 can be transferred to the asteroid belt with almost no fuel. In the other direction materials from Earth can be transferred out to as far as the Kuiper belt objects using almost no fuel.
Most asteroids and icy bodies are spinning quite rapidly and the spin can also be used to slingshot the materials to other locations in the solar system.
Since plants do well even in zero g, and much of a habitat may be used for plants, one suggestion might be to design some of the settlements around 1/100th of terrestrial gravity. That greatly reduces the engineering challenges. A hundredth of terrestrial gravity makes the load only 44.5 kilograms per square meter instead of 4.45 tons per square meter. These could be used for agricultural settlements or recreational areas, if ways can be found to integrate this with faster spinning regions for human habitation.
In short there seems potential here for most of the concerns and hopes of prospective Mars colonization enthusiasts to be met in other ways if we find we can never land on the surface of Mars because of issues of biological safety for humans, or for Earth's biosphere or Mars' biosphere (if any).
We can't control what we may find on Mars in the future, and can't know for sure in advance whether or not Mars is safe for humans. So it is good to have a "plan B" for the situation where we find that we can never land humans there safely, even if one is near certain in advance or very optimistic that we will find it is safe to land humans there.
For the scenario of a biologically unsafe Mars, Jupiter's moon Callisto with its abundant ice is an attractive destination with same planetary protection classification as the Moon
If we can travel to Mars we need ultra-reliable life support and ability to sustain it for years on end, Jupiter's moon Callisto then comes in reach.
It's much less of a step from Mars to Callisto than it is from the Moon to Mars, especially since they need to be able to handle any emergencies such as fires, chemical releases, explosions, major issues with life support and food or water supplies, and medical emergencies in situ in Mars orbit already. So, once humans are independent enough of Earth to be able to live in orbit around Mars this isn't far from a future where they are also independent enough to explore as far as Callisto and set up a base there.
Actually even for the journey time, especially with faster rockets, there's a much bigger difference between traveling to the Moon and traveling to Mars, than there is between traveling to Mars or traveling to Jupiter's moon Callisto. We need life support that can last for years without resupply for the journey to either place and with essentially no possibility of MEDVAC ito deal with any emergency health issue during the journey out or back.
This compares evacuation times:
ISS emergency evacuation a few hours, resupply every few months < day to arrive
Moon emergency evacuation 2 days, resupply takes 2 days to reach the Moon
Mars emergency evacuation minimum 6 months, emergency resupply minimum 6 months to arrive
(added text to this infographic from the Canadian space agency: Distances between Earth and the International Space Station, the Moon and Mars - infographic)
Actually especially with faster rockets, there's a much bigger difference between traveling to the Moon and traveling to Mars, than there is between traveling to Mars or traveling to Jupiter's moon Callisto. Because we need super-reliable life support that can last for years without resupply for either and capability to deal with medical emergencies with essentially no possibility of MEDVAC ito deal with any emergency health issue.
As our spacecraft get more capable (Adams et al, 2003, Conceptual design of in-space vehicles for human exploration of the outer planets), humans can also explore and even colonize Callisto, outermost of the Galilean moons of Jupiter (McGuire et al., 2003, High power MPD nuclear electric propulsion (NEP) for artificial gravity HOPE missions to Callisto). This is far more suitable than Europa which has major issues of potential forwards and backwards contamination as it may have liquid water near the surface and is positioned right in the middle of Jupiter’s deadly ionizing radiation belts.
Elon Musk’s artist’s impression of his spacecraft for a crew of 100, the Interplanetary Transport System. He said his spacecraft would use Europa as a refueling stop in the outer solar system. Callisto is a far better refueling stop because of the lethal ionizing radiation around Europa which is within Jupiter’s radiation belts. The artist’s impression actually more closely resembles Callisto as the surface of Europa is probably broken up and rough on the meter scale, at least with current understanding (SpaceX, 2016, Interplanetary Transport System)
Inset shows artist’s impression of an exploration base on Callisto (NASA, 2004, The Vision for Space Exploration : 22)
Many other destinations beckon as humans become more independent of Earth, such as Titan, only moon with an atmosphere, (depending on planetary protection status) out to beyond Pluto in time
To explore this alternative vision further see the Supplementary information section under:
- In all scenarios self sustaining settlement in space likely happens after we have easy colonization of the shallow sea floor, and floating sea cities and farms
- Even if we can never land humans on Mars, we can colonize our solar system with self sustaining habitats slowly spinning for artificial gravity to Pluto and beyond
- ⸽ Mars is only habitable with space suits and colonists living in pressurized habitats, never needed on Earth
- Earth as a jewel of a planet and we are evolved to live here
- Moon as a backup, closer to Earth and able to preserve seeds at liquid nitrogen temperatures at the poles
If we determine it is biologically safe to land on Mars, the surface colony will be far more resilient with a "Mars gateway" settlement in orbit
We saw how the landing on Mars is likely to be risky with the rockets skimming a few hundred meters above the surface. With NASA's current plans any Mars colonists would have very little by way of assets on the surface to support them as they see humans landing there early on in the exploration of Mars by humans.
With the Moon NASA see the lunar gateway as an important asset to help support astronauts on the surface even with only two -days flight from Earth (ADDCITE).
In this alternative vision we can see the Phobos settlement as like a Mars gateway - but it is also a gateway for the asteroid belt too and further afield or just a gateway to a thriving orbital settlement around Mars itself. Also unlike the lunar gateway, the martian moons make it one that can be supported using in situ resource utilization (ISRU);
In the same way as for the lunar gateway the Phobos gateway would help astronauts on the surface but even more so than for the Moon as it would give them a place to evacuate to with doctors, a field hospital by then, life support, provisions, fuel generated from the ice in Phobos if it does have ice or imported from Earth or the Moon or from asteroids, and so on. Likely also materials exported from Mars too such as fuel exported from Mars to orbit, depending how long it takes to establish that it is biologically safe for humans to land on Mars and that it won't interfere with either unique second genesis on Mars or interfere with potential future terraforming pans for Mars and so on.
As a result of all this, astronauts on the surface in this alternative vision are in a far safer position supported by a settlement of explorers in orbit. This also is far more resilient to public responses to a crash. If the first mission to Mars, private or public, ends with a crash with all onboard dying, then as for the Columbia and the Challenger space shuttle crashes it would be likely to lead to a review and might well stop space exploration as far as Mars by humans for some time.
If humans are already in orbit around Mars and living there, when a spacecraft crashes trying to land on Mars it would just stop / delay attempts to land on Mars and the orbital settlement would continue.
How NASA's permitted terrestrial contamination will mask any faint traces of past biosignatures reduced a further 1000 fold or more by only 70 million years of surface exposure
The permitted levels of organics likely seem low to engineers and geologists but is high for astrobiologists who design instruments to search for minute traces of early life on Mars, if there was life there the signal is likely to be weak and most of the organics we find is likely organic infall or organics so degraded as to be unrecognizable.
This is especially so however with new research from 2022 which found that ionizing radiation will reduce recognizable organics 1000 fold in just 70 million years. At that rate, from Pavlov et al’s result of a 1000 fold reduction every 70 million years, that’s a billion fold reduction every 210 million years. By 420 million years a gram of recognizable organics would be reduced to an attogram, a billionth of a billionth of a gram (Pavlov et al., 2022, Rapid Radiolytic Degradation of Amino Acids in the martian Shallow Subsurface … : 1111-2).
Previous research hadn't considered the effects of ionizing radiation on organics mixed with silica. Pure amino acids wouldn't be much affected but martian basalt will be around 50% silica and any organics even mixed with dry silica is rapidly destroyed in the presence of ionizing radiation.
NASA think they achieved:
- 8.1 ppb forward contamination by terrestrial organics, 0.7 per biosignature (Boeder et al.,
2020, Mars 2020: mission, science objectives and build : table 6). The target was 10 ppb total and 1 ppb per biosignature,
[These figures are for the levels of contamination of the returned samples themselves, not just the walls of the sample tubes.] - NASA's rocks may have up to 100 ppb of organics, but nearly all of that gets sliced into pieces by ionizing radiation, and the oxidizing effects of activated silica, which then recombine to the extent that the original structure of the molecules is unrecognizable. Consists almost entirely of small chain acids and the original composition is unrecognizable.
- We expect ≪ 0.1 ppb of recognizable past amino acids in even recently exposed surface organics on Mars after degradation by ionizing radiation (Pavlov et al., 2022, Rapid Radiolytic Degradation of Amino Acids in the martian Shallow Subsurface: Implications for the Search for Extinct Life : 111)
- This happens with surface exposure ages younger than the youngest exposure age measured by Curiosity - and Perseverance can’t measure exposure ages (Pavlov et al., 2022, Rapid Radiolytic Degradation of Amino Acids in the martian Shallow Subsurface: Implications for the Search for Extinct Life : 111 - 112)
- Most of the organics with young exposure ages decomposed to: formate (HCO2), oxalate C2O42-) and some benzoate (C6H5OO-) (Pavlov et al., 2022, Radiolysis of macromolecular organic material in Mars-relevant mineral matrices)
(N.B. the probability for a viable organism is per sample tube, their best estimate implies a 0.02% chance of finding a single viable terrestrial organism in at least
one of the 38 tubes).
The Curiosity and Perseverance drills are designed to sample to a depth of 5-6 cms which is enough to avoid the effects of the surface UV (blocked by a millimeter of rock) and oxidants (penetrate to a depth of several cms) (Pavlov et al., 2022, Rapid Radiolytic Degradation of Amino Acids in the martian Shallow Subsurface: Implications for the Search for Extinct Life : 1100). However it’s not enough to avoid effects of ionizing radiation which penetrates to several meters.
A mix of pure amino acids such as one would expect in an organically rich sample would be reduced only to only 45% by 80 million years exposure but nothing like that has been found on Mars. Reduced organics have only been found at less than parts per million (Pavlov et al., 2022, Rapid Radiolytic Degradation of Amino Acids in the martian Shallow Subsurface … : 1111).
Basalt by definition has between 45 and 52% silica (Le Bas et al.,1991. The IUGS systematics of igneous rocks), a low silica rock and one of the most abundant rocks on Mars so we can expect silica rich rocks on Mars,
Even low molecular weight amino acids are reduced from 100 ppb to 0.1 ppb in only 70 million years in the best case (just silica and no water or perchlorates). For clays with water incorporated, it’s only 30 million years to reduce 1000 fold (Pavlov et al., 2022, Rapid Radiolytic Degradation of Amino Acids in the martian Shallow Subsurface … : 1111). Even a 2 meters depth is not enough to shield against significant degradation over longer periods of time with 1000 fold reduction in 500 million years for dry silica rich rock without any perchlorates and a 100,000 fold reduction if the rocks contain perchlorates (Pavlov et al., 2022, Rapid Radiolytic Degradation of Amino Acids in the martian Shallow Subsurface … : 1111).
So:
There may be some potential for higher levels of complex organics than this from new results published in July 2023.(Sharma et al., 2023, Diverse organic-mineral associations in Jezero crater, Mars) and summarized in (Steele, 2023, An in situ search for organic molecules in Mars’s Jezero Crater). If Perseverance's Raman spectrometry did indeed spot organics, and the evidence seems good that they did, this is a finding of up to 20 ppm bulk concentration in the rock they call Máaz, in rocks that show signs of alteration by water in the past. This would suggest that it is organics produced indigenously rather than infall.The more complex organics were consistent with compounds with two benzene rings, including naphthalene (the rings share two carbon atoms in common) and biphenyl (with a carbon to carbon bond joining the two rings).(Sharma et al., 2023, Diverse organic-mineral associations in Jezero crater, Mars : fig. 3).
This is one of the motivations for adding bonus samples to the NASA Sample Retrieval Lander mentioned above:
NASA's permitted levels of contamination: based on research from 2014, which takes account of direct effects of ionizing radiation but doesn't consider activation of oxidants by the radiation
NASA did comply with requirements set by NASA's iMost team in 2017. This in turn was based on the Organic Contamination Panel from 2014. They used the levels of organics in martian meteorites to set the limits NASA now use. They tried other lines of evidence but this was the only one they had reasonable confidence in.
The main issue with this is that martian meteorites aren't exact analogues of surface rocks because they were ejected from at least three meters below the surface on Mars. The Organic Contamination Panel only looked at this briefly and didn't have access to the 80 million years exposure age measured by Curiosity.
The limits for Earth-sourced organic carbon in/on the samples originated from a nearly year-long study by a science/Planetary Protection team (OCP) carried out in 2014 (2). Their final recommendation was organized into three components: <1 ppb Tier 1 compounds (organic compounds that would be deliberately evaluated in returned samples as input to life-related interpretations), <10 ppb other°C -compounds (everything that is not on the Tier 1 list), and <40 ppb total organic carbon (TOC).
(Beaty et al., 2018, iMOST : 176)
This in turn was based on the deliberations of the Organic Contamination Panel in 2014 (that's the year long study they refer to in the quote).
The Organic Contamination Panel only briefly took account of the effect of ionizing radiation on the levels of biosignatures in the samples.
They say:
On the other hand, martian meteorites arriving on Earth were likely blasted into space from well below the martian surface, where any organics present would have been shielded from the highly oxidizing and radiolytic surface environment. While it is true that near-surface exposure to cosmic radiation likely degrades organic molecules, recent work on Curiosity shows that at least some locations on Mars are eroding fairly rapidly (Farley et al., 2013). Such locations are likely targets for sample collection.
(Simmons et al., 2014, Planning Considerations Related to the Organic Contamination of martian Samples and Implications for the Mars 2020 Rover : 36)
Their cite is to the Farley et al cite from 2013 / 2014 (Farley et al., 2014, In situ radiometric and exposure age dating of the martian surface)
However they didn't have access to more recent work on the rapid radiolytic degradation of even such young samples (Pavlov et al., 2022, Rapid Radiolytic Degradation of Amino Acids in the martian Shallow Subsurface: Implications for the Search for Extinct Life : 1100 - 1101) .
They looked at the likely levels of organics from infall from space and estimated it as 0.2 to 2 ppb for a 100 meter mixing depth or 20 to 200 ppb per biosignature for a 10 meter mixing depth, based from calculations by Benner et al in 2000.
They say most of these organics would be oxidized but that it would be possible to measure the oxidation products (Simmons et al., 2014, Planning Considerations Related to the Organic Contamination of martian Samples … : 40).
They looked at the in situ detection of organics by Viking, Phoenix and Curiosity but there is a significant limitation here that none of these instruments could measure levels of large molecules of interest in situ on Mars because they break them up into smaller molecules using pyrolysis (heating in absence of oxygen) to turn them into volatiles that can be analysed by the mass spectrometer. (Simmons et al., 2014, Planning Considerations Related to the Organic Contamination of martian Samples … : 39).
They said:
Finding #10: Because we fundamentally do not know what organics would be present on Mars, it is currently impossible to precisely determine what levels of contamination would be necessary in returned samples. There is thus significant uncertainty (in both directions) associated with the proposed limits.
(Simmons et al., 2014, Planning Considerations Related to the Organic Contamination of martian Samples and Implications for the Mars 2020 Rover : 29)
They suggested three ways to set a threshold
- Signal to noise ratio, the issue is that they don't know the compounds we expect to find or the lower limits of their concentrations
- Detection limits of the instruments used, this however would require 100% clean samples as some instruments have single molecule detection limits
- Levels of cleanliness that can reasonably achieved for constructing sampling hardware. This could mean developing new technology to achieve those levels.
(Simmons et al., 2014, Planning Considerations Related to the Organic Contamination of martian Samples and Implications for the Mars 2020 Rover : 29)
For the signal to noise ratio, they based this on an analysis of the organics in our martian meteorites such as Tissint meteorite, and carbonaceous chondrites, the organics in Murchison meteorite.
(Simmons et al., 2014, Planning Considerations Related to the Organic Contamination of martian Samples and Implications for the Mars 2020 Rover : tables 6 and 7)
They found that molecules of interest in the martian meteorites ranged from 1 to 100 ppb (nanograms per gram). Based on that they believed that setting a limit of 1 ppb would mean that many of the molecules are detectable against the noise of terrestrial contamination.
Based on the evidence discussed above, our best estimate for concentrations of the most abundant organic molecules of interest (i.e., Tier-I compounds) in returned martian rocks is in the range of 1-100 ng/g. We thus believe that these compounds would likely be measurable above background contamination comprising <1 ng/g per compound. Such background levels should be readily achievable given current technology, and would be at the low end of what is measurable by current survey analytical techniques, thus protecting their role in initial characterization of returned samples. We therefore propose a maximum limit for Tier-I compounds, on a mass/mass basis in returned samples, of 1 ng/g (i.e. < 1ppb).
It is of course possible that analyte concentrations in the returned samples may turn out to be lower than expected, and so the odds of scientific success would be improved by still lower contamination limits. Moreover, lower background levels would permit more accurate and precise measurements at any concentration. Nevertheless, given currently available evidence, it is hard to build a case that contamination limits substantially lower than this would be required to meet either scientific or planetary protection objectives.
(Simmons et al., 2014, Planning Considerations Related to the Organic Contamination of martian Samples and Implications for the Mars 2020 Rover : 42).
So they proposed a limit that would achieve a 10 : 1 signal to noise ratio.
They agree that it's inevitable many compounds of interest in the samples would be present at much lower levels, but most of the contaminants would also be present at significantly less than 10 ppb.
A frequent point of discussion for the OCP was “What happens to compounds that are less abundant in the returned samples than those discussed above?”.
Certainly it is inevitable that many compounds would be present at <1.0 ng/g. However, setting a contamination limit of 1 (or 10) ng/g does not imply that every compound in Tier I (II) would be present at that level. Rather, sampling surfaces would be cleaned until the most abundant contaminant meets that level, and most other compounds would then be present at much lower levels. The panel thus believes that this strategy represents a sensible compromise, providing reasonably achievable goals while at the same time ensuring that the vast majority (though not necessarily all) analytes of interest would be measurable
(Simmons et al., 2014, Planning Considerations Related to the Organic Contamination of martian Samples and Implications for the Mars 2020 Rover : 42).
For the total organic carbon they expect concentrations of up to 10 ppm (micrograms per gram) and setting a total organic contamination level of 40 ppb gives a signal to noise ratio of more than 100 : 1. ((Simmons et al., 2014, Planning Considerations Related to the Organic Contamination of martian Samples and Implications for the Mars 2020 Rover : 49).
The issue here is that the martian meteorites were ejected into space from at least 3 meters below the surface (Eugster et al., 2002, Ejection ages from krypton‐81‐krypton‐83 dating and pre‐atmospheric sizes of martian meteorites : 1355), so the levels of organics in those meteorites are not representative of what we might find on the surface, exposed to high levels of ionizing radiation for at least 80 million years for Curiosity's youngest samples. Our martian meteorites that left Mars most recently spent less than a million years traveling through space from Zunil crater (Hartmann et al., 2020, Do young martian ray craters have ages consistent with the crater count system? : 626), as we saw above in How NASA's central finding that any harmful life can get here better protected in meteorites is rebutted by BOTH of its main cites for it
They also have less oxidants than surface materials which makes them more resilient to ionizing radiation.
They considered in situ measurements of organics, but the data wasn't good enough to set a limit based on measurements from Mars itself because the organics are decomposed into smaller molecules using pyrolysis (heating without oxidation) as part of the processes of analysis used so far (Simmons et al., 2014, Planning Considerations Related to the Organic Contamination of martian Samples and Implications for the Mars 2020 Rover : 40)
For detectable levels by present day instruments, they found that it wasn't practical to consider detection limits because of ultrasensitive instruments and so they looked at instruments suitable for initial surveys, finding a range of 0.1 to 10 ppb, and selected 1 ppb as a representative value (Simmons et al., 2014, Planning Considerations Related to the Organic Contamination of martian Samples and Implications for the Mars 2020 Rover : 41)
As for achievable levels of contamination they were not able to answer this. Geologists don't attempt ultra clean samples but rather reduce levels of organics to be low enough for the measurements they want to make. So they didn't use this criterion (Simmons et al., 2014, Planning Considerations Related to the Organic Contamination of martian Samples and Implications for the Mars 2020 Rover : 41-2)
So they used the signal to noise ratio to reach their conclusion
That lead to their conclusion
Major Finding #13: We propose the following limits for organic contamination of geological samples by specific compounds: 1 ng/g for Tier-I compounds deemed as essential analytes for mission success, and 10 ng/g for Tier-II compounds (all others)
(Simmons et al., 2014, Planning Considerations Related to the Organic Contamination of martian Samples and Implications for the Mars 2020 Rover : 43)
With this level of contamination we would detect organics from Mars but it seems unlikely we detect the small trace amounts left after the combination of ionizing radiation and oxidants unless we can find samples with exceptionally young exposure ages.
No possibility of a genetic inventory of terrestrial contamination: over 1000 genera had too few reads to identify, with enough organics to mask thousands of ultramicrobacteria from Mars
We currently have no way to reliably distinguish terrestrial from potential martian biosignatures. We could recognize familiar life like chroococcidiopsis which we already cultivate and have already sequenced. However, the vast majority of microbial species haven’t been characterized or sequenced or cultivated in the laboratory; the problem of “microbial dark matter” (Dance, 2020, The search for microbial dark matter).
The iMost team suggested a genetic inventory:
To appropriately interpret evidence for Mars life in returned samples, we must be able to distinguish between terrestrial contaminates and indigenous martian life. For this reason, a genetic inventory of both the spacecraft and sample processing/analysis facilities is critical … A genetic inventory represents an important part of the background information related to detection of genetic material in Mars spacecraft and returned samples.
(Beaty et al., 2018, iMOST : 94)
However the issue of microbial dark matter makes it impossible to achieve this goal. Hendrickson did an attempt at a genetic catalogue using 98 swabs from the floors of the clean room that would be used to assemble the Perseverance rover before assembly started. However,
- of 54 identified genera, only 8 were spore forming
- Out of 1250 genera, only 54 had enough reads to be identified properly (using the 16s subunit of the ribosome). That leaves 1196 distinct but unidentified genera detected in those 98 swabs.
- 36 out of 49 spore forming species (not genera) were only found in one of the 98 swabs
- 4 of the identified species weren’t close to any known terrestrial species (<
98.7% similarity of the 16s subunit to the most similar relative).
(Hendrickson et al., 2021, Clean room microbiome complexity impacts planetary protection bioburden)
So, 1196 genera were known to be present but couldn’t even be identified. This is not unusual, indeed this is expected and normal.
They used the 16S RNA ribosome subunit for identifying microbial dark matter as it is very stable, and is the basis for the modern classification method for microbes and other organisms due to Carl Woese (Sapp et al., 2013, The singular quest for a universal tree of life). It is a short section of RNA which gets mixed with proteins to make up the structure of the ribosome used to translate RNA into proteins.
We are certain to detect many novel sequences like this after taking them to Mars and back. So we will get many genetic sequence false positives, and it will be impossible to prove they aren’t martian.
Also the life would have to be very abundant to spot it. A typical sample will be 10 to 15 grams. A 15 gram sample at 8.1 ppb is over 120 nanograms of material (Moeller, 2020, The Sampling and Caching Subsystem (SCS) for the scientific exploration of Jezero crater by the Mars 2020 Perseverance rover : 39-40)
Total Organic Cleanliness of a Sample: Each sample in the returned sample set has less than 10 ppb (parts per billion) baseline or 40 ppb threshold of total organic carbon. Here, 10 ppb baseline can be interpreted as the desired requirement while 40 ppb threshold is the not to exceed requirement. For a 15 g core sample, this equates to 150 ng baseline and 600 ng threshold.
(Boeder et al., 2020, Mars 2020: mission, science objectives and build : table 6)
One part per billion by mass is the same as one nanogram per gram.
Text on graphic : Example of how design decisions for Perseverance were based on engineering and geology rather than astrobiology.
This tube was used to collect the first sample from Mars.
For a geologist, it is exceptionally clean, at most 8.1 nanograms of organics and at most 0.7 nanograms per biosignature.
For an astrobiologist, 0.7 nanograms per biosignature is enough to fill at least 7,000 ultramicrobacteria with just that biosignature, e.g. glycine, or DNA (maximum volume 0.1 cubic microns per ultramicrobacteria)
Astrobiologists need 100% clean sample containers with no organics. Their life detection instruments designed for in situ searches on Mrs can detect a single amino acid in a gram.
For engineers, sterilization would add an extra mission critical failure point because they would need to open the sterile container for the tube on Mars.
Sample tube photo from (NASA, 2021, Perseverance Sample Tube 266)
These are the detailed contamination requirements:
(Boeder et al., 2020, Mars 2020: mission, science objectives and build)
Though as we saw they think they overachieved at 8.1 ppb of total organics and 0.7 ppb maximum for any tier 1 compound. They don't say which is most abundant but this would mean e.g. they could have 0.7 ppb of glycine and also 0.7 ppb of alanine because they are measured separately. It's not a total for all amino acids. They don't measure all the amino acids but just two representative ones to get an idea of the level of contamination.
By definition an ultramicrobacteria has a volume of at most 0.1 cubic microns (Nakai, 2020, Size Matters: Ultra-small and Filterable Microorganisms in the Environment). A micron is a millionth of a meter or a 10,000th of a centimeter. So a cubic micron has a volume of a trillionth of a cubic centimeter, or a mass of a trillionth of a gram, assuming density similar to water. So based on ultramicrobacteria at a tenth of a cubic micron each, there are 10,000 of them for every part per billion.
So those 120+ nanograms are equivalent to 1.2 million ultramicrobacteria per sample tube,. You'd find it hard to notice a few tens of thousands of them especially if they were related to terrestrial life - or based on some exotic biology that our tests don't pick up at all.
Spores would be a bit larger. The martian conditions might favour spore forming microbes and we might find spores in the martian dust. The b. subtilis spore, one of the smallest spore forming microbes, is typically 1.2 μm long and 0.8 μm wide so its volume is about a cubic micron, increasing to 1.8 μm long and 1.2 μm wide on hydration (Chada et al., 2003, Morphogenesis of Bacillus spore surfaces). So there would be a thousand of those in each nanogram.
Witness tubes will let us subtract some terrestrial contamination but with too much noise to reveal orders of magnitude weaker signals from any native li fe biosignatures
The "witness tubes" which collect terrestrial contamination in a dummy run of sample collection can help geologists subtract contamination to get a good idea of the original levels of organics - but are not enough to reveal the minute traces of biosignatures astrobiologists are interested in
They use witness tube assemblies to detect terrestrial organics. They are cleaner than the normal sample tubes, cleaned to a very high level and sealed and only opened on Mars, and they have materials inside which detect any organics. They go through similar processes to the normal sample tubes - and then they do a dummy run where the witness tubes go through all the same handling procedures as a normal sample tube except they don't collect any sample. Instead they collect any contamination from the sampling apparatus. (Moeller, 2020, The Sampling and Caching Subsystem (SCS) for the scientific exploration of Jezero crater by the Mars 2020 Perseverance rover : 30-31)
As another control they have a "drillable blank assembly". This is a sterile silicon dioxide brick covered in aluminium. Perseverance drills a sample from it just as it would from the Mars surface and collects it in a sample tube in the same way as the other samples. They then know any organics in this dummy sample were introduced by the drilling and sample collection process (Moeller, 2020, The Sampling and Caching Subsystem (SCS) for the scientific exploration of Jezero crater by the Mars 2020 Perseverance rover : 5). They also have a witness tube to witness the contamination of the drill bits themselves, from assembly through to launch and landing on Mars.
The idea is that they can subtract the organics they find in these witness materials from the organics in the contaminated samples to get an idea of what the samples were like before contamination. This will be very useful for geologists.
The problem for astrobiology - at least for the Perseverance samples - is that you are looking for very minute traces of biosignatures and 150 ng of terrestrial contamination or even 8.1*15 = 121.5 nanograms is a lot of contamination. That means that it is going to overwhelm the tiny traces you expect of past or present day life on Mars unless it is very abundant.
They expect to return some samples with over 100 ppb of organics, which would be 1,500 nanograms in a 15 gram sample. Compared to that, 150 nanograms or less, maybe only 121 nanograms seems little to a geologist.
However, astrobiologists are interested in biosignatures and recognizable organics. With even 70 million years of surface ionizing radiation those 1,500 nanograms are reduced to 1.5 nanograms of recognizable organics (Pavlov et al., 2022, Rapid Radiolytic Degradation of Amino Acids in the martian Shallow Subsurface: Implications for the Search for Extinct Life : 1100 - 1101). The rest would be short carbon chains like oxalate, formate or it might have benzene rings as benzoate. So how you are going to spot those interesting organics amongst 150 nanograms of contamination? Curiosity's youngest age is 80 million years (Farley et al., 2014, In situ radiometric and exposure age dating of the martian surface)and Perseverance can't measure exposure ages.
Perseverance could help here by collecting a sample from a recently exposed crater. They could get it down to less than 100,000 years of exposure age. In that case they might have 100 ppb of interesting organics.
However, even if we find a deposit as rich in past organics as our oil shales, then the most interesting organics, the original least altered organics, may be in small quantities and still modified after deposition through diagenesis
Diagenesis is a process of compaction under mild conditions of temperature and pressure. When organic aquatic sediments (proteins, lipids, carbohydrates) are deposited, they are very saturated with water and rich in minerals. Through chemical reaction, compaction, and microbial action during burial, water is forced out and proteins and carbohydrates break down to form new structures that comprise a waxy material known as “kerogen” and a black tar like substance called “bitumen”. All of this occurs within the first several hundred meters of burial
(Fuel Chemistry Division, Oil shale)
Then, most likely it's mixed with infall from space, and is chemically altered and most of the original past life organics, if any, has been washed out or modified. So they are interested in very small trace amounts that might perhaps still be unaltered from 3 billion years ago, so even then the 8.1 ppb of contamination would likely overwhelm the organics they are interested in. If there are any biosignatures left they might be in small quantities - and especially so if Mars hadn't yet developed photosynthesis 3 billion years ago.
I cover that (below) See:
It's the same for present day surface processes. Astrobiologists are interested in small trace amounts of organics in the atmosphere and in the soil. So even then it will be hard to do much meaningful astrobiology with this level of contamination.
The terrestrial organics would overwhelm the trace organics they are interested in, unless we are lucky enough to return recognizable past microfossils (with a high chance whatever we find is controversial as for ALH84001), or large amounts of present day life.
Why the COSPAR Sample Safety Assessment Framework can't be used to prove safety of unsterilized Perseverance samples
NASA propose to use the COSPAR Sample Safety Assessment Protocol (SSAP) Working Group and its Sample Assessment Safety Framework (SSAF), to prove safety of the samples to release unsterilized.
Ultimately, the SSAP Working Group findings, through an external independent peer- reviewed process, will evolve over time as knowledge of sample constituents evolves and scientists identify certain requirements and protocols that should be implemented to ensure sample safety throughout the sample management, handling, and curation process (Kminek et al. 2022).
(NASA, 2023. Mars Sample Return final PEIS : 2-17)
However there are many issues with this that we will see make it impossible to use it for the Perseverance samples.
The Apollo mission tested safety of the lunar samples with a number of terrestrial test organisms. However this isn't seen as a way to reliably do safety testing today.
The SSAP Working group came to the conclusion that it is in practice impossible to prove the samples are safe by testing the material with terrestrial organisms.
During the Working Group’s deliberations, it became clear that a comprehensive assessment to predict the effects of introducing life in new environments or ecologies is difficult and practically impossible, even for terrestrial life and certainly more so for unknown extraterrestrial life.
To manage expectations, the scope of the SSAF was adjusted to evaluate only whether the presence of martian life can be excluded in samples returned from Mars.
If the presence of martian life cannot be excluded, a Hold & Critical Review must be established to evaluate the risk management measures and decide on the next steps.
(Kminek et al, 2022, COSPAR Sample Safety Assessment Framework (SSAF))
So they concluded that the only way to prove the samples safe is to show there is no martian life in them. We can't do that by attempting to cultivate martian life either, because even most terrestrial life is not cultivable in the labs, though they do well in the wild, for instance if there is any martian life, it may require specific nutrient poor conditions and not be able to cope with the amounts of nutrients present in lab culture media, or may be symbiotic on other microbes in a biofilm, using biochemicals which they may also be able to source from terrestrial biofilms.
That left only one way to prove the samples are safe, which is to show that any traces of life in the samples come from Earth.
The plan is to do that by checking for biosignatures. See (Kminek et al, 2022, COSPAR Sample Safety Assessment Framework (SSAF): table 3). They can only say there is no life if they find no biosignatures, Even then they also have to consider the potential for martian life that may not be detectable with the usual tests for terrestrial biosignatures.
This step is bound to detect terrestrial contamination since the sample tubes are not 100% sterile and nor is the drill apparatus. See above:
If this step detects biosignatures that they can't prove are from Earth they have to assume they are from Mars and all the samples go to hold and critical review. That would then need new knowledge before we can show they are safe and it is hard to see how that knowledge could be obtained except by more in situ study on Mars itself.
For the SSAF to work the group says that it needs:
- contamination knowledge of the terrestrial material accidentally added to the samples during sample collection and retrieval
- terrestrial contamination levels to be ultra low
- that there is enough martian life in the sample, if present, to detect it by the methods used.
[They list many other requirements and here I have extracted three of their points that seem of especial importance]
On that last point, terrestrial microbes are so numerous that almost anywhere on Earth we can detect the native microbes even with quite high levels of contamination from our instruments. However the areas of earth with the lowest concentrations of microbes in the hyperarid core of the Atacama desert and the McMurdo dry valleys in Antarctica would correspond to high concentrations on Mars, microbial biodiversity hotspots.
This suggests we need ultra low levels of terrestrial contamination because anything more will easily mask the likely ultra low concentrations of microbes over most of Mars. Preferably we need zero terrestrial contamination.
There are serious issues with applying all three of these SSAF requirements to the Perseverance samples.
The Perseverance team don't have a genetic inventory of the terrestrial contamination that would be of much use for safety testing. They found 1250 genera in 108 swabs of the Perseverance clean rooms and were only able to identify 54 of them. The remaining 1196 genera couldn't be identified though they had enough information to know they were distinct from each other. Amongst the individual species they were able to identify, four had less than 98.7% resemblance to any previously known species. See above;
With the intense scrutiny of the returned samples astrobiologists are bound to find numerous new species and genera of terrestrial life in them, and it will likely be impossible to tell if they are closely related species and genera from Mars or are terrestrial genera or species. We would need contamination knowledge at the level of individual species or strains to pick up strain-specific toxins, see below:
Then the amount of terrestrial contamination though low for geology is enough for thousands of ultramicrobacteria per sample tube and so would easily mask any faint signal of a few microbes of native life. It would be possible to detect a habitat if the tubes directly sampled a habitable area of Mars but this is exceedingly unlikely. In the very harsh conditions of Jezero crater, most likely it just would have a few stray microbes some of which may be viable as we saw above:
Then the assumption that there would be enough martian life in the sample tubes to notice is not well established as there are likely to be ultra low concentrations of life in the samples.
There are many potential habitats that could be sources of ultra low concentrations of microbes in the Perseverance samples, such as local microhabitats such as caves, and salts that may be present even in Jezero crater or more distant microhabitats that may be a source of viable life that gets to the samples in dusts storms. The SSAF doesn't work for ultra low concentrations if there are only a few microbes, maybe even one viable microbe in the entire sample collection. See below:
I cover these issues in the attachment 7 (Walker, 2022, So many serious mistakes in NASA's Mars Samples Environmental Impact Statement it needs a clean restart) (docx)) in the section titled: "How would they prove the samples are safe on Earth? Permitted levels of biosignatures from contamination by terrestrial life guarantee false positives"
NASA's team didn't review the issues they just quoted a small part of it which didn't go into the details of the issues I found, and responded to it:
NASA: The safety and security of Mars return samples are of upmost priority for the MSR Campaign and includes a commitment to following the guidelines set forth by the SSAP Working Group. The campaign will leverage the latest scientific findings and protocols necessary to ensure sample safety and containment.
(NASA, 2023. Mars Sample Return final PEIS : B-65)
NASA use this requirement for safety testing of samples as a criterion to rule out of consideration any alternative that can't definitively determine if the samples are of sufficiently low risk to turn this from a restricted to an unrestricted sample return mission:
Additionally, given the constraints described above, there is no instrument or suite of tests that Perseverance can use on Mars or that the MSR Campaign could bring to Mars, to definitively determine if the samples collected are of sufficiently low risk so as to alter the "Restricted Earth Return" mission planetary protection designation and being treated as if they are potentially hazardous.
(NASA, 2023. Mars Sample Return final PEIS : 2-25)
However it seems that if they applied these rules to their own plans, they would have to rule out their own plans too. Their is simply no way to achieve their aim with the Perseverance samples especially with the high levels of terrestrial contamination for a mission that can only expect to find ultra-low traces of life on Mars, if it finds anything, with no capability to look for patches of past or present day life in situ.
We will also find that because of the possibility of ultra low levels of life on Mars it seems to be impossible to prove safety even for much cleaner indeed contamination free returned samples.
See:
This seems to be something that can only be resolved with more knowledge of Mars itself which we can only learn with in situ studies, rather than by studying the samples as we could never rule out the possibility of a stray viable microbe that we haven't yet found, say, imbedded in a grain of dust, in the samples.
We may only be able to resolve this with Sagan's "exhaustive program of unmanned biological exploration of Mars": (Sagan, 1973, The Cosmic Connection – an Extraterrestrial Perspective). However, NASA#s team are unaware of how difficult this task is, or how it has been further complicated by the terrestrial contamination in the samples and the lack of a genetic inventory.
For the NEPA issues with this see Supplementary information under:
- NASA required alternatives to definitively determine if it is safe to return unsterilized samples, even if all returned samples are sterilized, yet there will be no way even for terrestrial labs to prove the samples don't have small numbers of martian microbes
So now let's look at those issues with proving safety of the samples in more depth:
To prove the samples are safe, it is essential to have contamination knowledge at the level of individual species or strains due to strain-specific toxins, which is impossible
Also - even if we found very familiar life, such as another strain of chroococcidiopsis on Mars, it could have developed novel capabilities in the very different conditions on Mars. It could have added
- a new metabolic pathway,
- more efficient photosynthesis,
- some new toxin,
- an undetected new fungal parasite
- undetected inherited prions
- could co-exist in the sample with undetected novel biology such as life based on mirror organics
Any of these could make it unsafe to return to Earth. It's especially likely that related strains on Mars could produce some accidental new toxin because they have to adapt to such very different hostile conditions there. Microalgae produce many accidental toxins. As an example, Chroococcidiopsis Indica produces BMAA, a neurotoxin which can cause Lou Gerig syndrome, the disease Steven Hawking had. (Cox et al., 2005, Diverse taxa of cyanobacteria produce β-N-methylamino-L-alanine, a neurotoxic amino acid). (Warmflash et al, 2007, Assessing the Biohazard Potential of Putative martian Organisms for Exploration Class Human Space Missions).
There are numerous other toxins expressed by cyanobacteria and often they depend on a gene carried by a particular strain.
According to some estimates, 25% to 75% of cyanobacteria blooms are toxic in one way or another (Bláha et al., 2005, Toxins produced in cyanobacterial water blooms - toxicity and risks)
"Each toxin is produced by cyanobacteria only when the appropriate toxin gene is carried by a particular strain and if its expression is activated by environmental conditions
Cyanotoxins are usually classified in four classes according to their toxicological target:
- hepatotoxins that act on liver (Microcystins and Nodularin)
- cytotoxins that produce both hepatotoxic and neurotoxic effects (Cylindrospermopsin)
- neurotoxins that cause injury on the nervous system (Anatoxins, Saxitoxins and ß-Methylamino-L-Alanine -BMAA-) and
- dermatoxins that cause irritant responses on contact (Lypopolysaccharide, Lyngbyatoxins and Aplysiatoxin
(Sanseverino et al., 2017, Cyanotoxins: methods and approaches for their analysis and detection)
These novel toxins could also be transferred from martian life to terrestrial microbes via gene transfer agents even if the martian life can't grow on Earth.
More generally, we can’t know that unsterilized samples from Mars are safe for Earth until we have a much better understanding of Mars’s biosphere, if any, know what to look for, what its capabilities are and so on.
However as we've seen, because of the high level of forward contamination we are certain to detect terrestrial life and sequence it. Even with many more swabs of the Perseverance clean room it would have been unrealistic to classify all the terrestrial contamination at the level of strains or species. So we can never have sufficient contamination knowledge of the samples to know if what we detect is from Earth or is a closely related strain from Mars that may have strain specific toxins developed in the novel conditions on Mars.
The COSPAR Safety Assessment Framework is unusable even for clean bonus samples for ultra low concentrations of microbes, such as brought to Jezero crater in the dust
The COSPAR Sample Safety Assessment Framework (SSAF) refers to this problem that there is no guarantee that any martian life has got into the subsamples examined.:
There is also another complication: even if there is life somewhere in the sample tube, there is no guarantee that there will be life in the subsamples that are examined.
(Kminek et al, 2022, COSPAR Sample Safety Assessment Framework (SSAF))
The group uses a parameter
Capture rate (Cr): the probability that a subsample contains martian life, given that life is somewhere in the sample tube
(Kminek et al, 2022, COSPAR Sample Safety Assessment Framework (SSAF) : 3.1.2 )
Nearly all terrestrial samples will have abundant life in them leading to a capture rate of 1, i.e., if there is life in the samples, it will be in any subsample we take.
However it is possible to find lifeless samples even on Earth, for instance basalt that has only just cooled from a lava flow.
Freshly formed basalt is quickly colonized, but there has to be a first microbe to find it. What we return from Mars might be similar to a basalt lava flow which has only been found by one microbe so far.
So, we might in the worst case have only a single microbe in the entire collection of samples obtained by Perseverance, and in the worst case, that microbe might also be viable. This is possible because the conditions on Mars are so very inhospitable for life almost everywhere.
In this case the capture rate would be almost zero.
For a “toy model” to illustrate the idea, suppose the surface of Jezero crater has millimeter scale microbial oases regularly spaced every 100 meters in a grid pattern. Suppose it also has single viable microbes scattered from those oases throughout the surface, one every 10 centimeters. Now we return a 10 by 10 cm sized patch of dirt to Earth. There is only 1 chance in a million that it samples a microbial oasis with enough microbes to detect, but certainty that it contains a single viable microbe almost impossible to detect with methods we have available today.
In a more realistic situation, if there is life on Mars, there will be a mix of dead and viable microbes, and the microbial oases are likely much further apart and many may be larger. The microbes will also be distributed in dust storms and vary in concentrations over the surface.
However the number of microbes in the sample may be very small compared to terrestrial samples and whether any are viable may depend on factors such as whether there is a microbial oasis nearby, and how resilient martian life is to UV.
Also the microbes may be imbedded in cracks in dust grains and hard to spot. Suppose that only 1 in 10,000 dust grains contains a viable microbe, and that most of the microbes that haven’t been dislodged from dust grains yet are viable. Then we could inspect 10,000 dust grains and by chance none of them contain any viable life and conclude that it is safe to release the 10,001th dust grain which by chance contains a viable martian microbe.
We conclude it is impossible to achieve a high level of assurance that it is safe to release even the bonus samples from containment without far more in situ study on Mars.
This issue could be very acute in some scenarios
Scenario of life in the martian dust at ultra low concentrations, a few viable cells per gram or less, brought to Jezero crater from distant locations on Mars.
This was identified as a knowledge gap in the Space Studies Board review of SR-SAG2. The PEIS uses SR-SAG2 but doesn't mention the Space Studies Board review of it, which was commissioned by NASA and ESA out of concerns that SR-SAG2
"The SR-SAG2 report does not adequately discuss the transport of material in the martian atmosphere. The issue is especially worthy of consideration because if survival is possible during atmospheric transport, the designation of Special Regions becomes more difficult, or even irrelevant."
Microbes could be transferred from distant parts of Mars and still be viable, for instance in fragments of biofilms (Billi et al.., 2019, A desert cyanobacterium under simulated Mars-like conditions in low Earth orbit: implications for the habitability of Mars). They might also travel in bouncing sand grains (Finster, 2018, Wind-driven saltation: an overlooked challenge for life on Mars).
Sagan suggested a viable microorganism could be imbedded in a dust grain and be protected from the UV by the iron oxides in the dust ( Sagan et al., 1967, Contamination of Mars. : 8)
Billi et al made a similar suggestion:
… Our findings support the hypothesis that opportunistic colonization of protected niches on Mars, such as in fissures, cracks, and microcaves in rocks or soil, could have enabled life to remain viable while being transported to a new habitat
(Billi et al.., 2019, A desert cyanobacterium under simulated Mars-like conditions in low Earth orbit: implications for the habitability of Mars).
The largest moving dust grains detected by Curiosity reached above 6 microns in diameter for 3 days during the 2018 dust storm (Lemmon et al., 2019, Large dust aerosol sizes seen during the 2018 martian global dust event by the Curiosity rover : Figure 4 and discussion of it).
I can't find any experiments that test this hypothesis of microbial survival completely imbedded in cracks within dust grains with dust grains of any size. If anyone reading this knows of such an experiment please let me know.
Microbes evolved on Mars might also be likely to develop extra layers to resist UV.
Scenario of a random sampling of a few stray microbes from a nearby colony that Perseverance didn't detect
Perseverance has no in situ life detection capabilities and martian life could be mixed with the dust, dark in colour, and not obvious to the rover. Perseverance could randomly sample the very edge of a colony or just outside it where a few stray microbes might still be found, at similar concentrations - of just a few cells per sample tube or even only one viable cell per gram or less.
This issue of biological oases is especially acute in the most inhospitable places:
When searching for evidence of life, the probability of a false negative result is highest in environments where potential biosignatures occur at very low abundance (e.g., due to low productivity or to degradation/destruction processes), operating at a very low (or even dormant) metabolic state, or where life is not distributed homogeneously (i.e., biological oases amidst an abiotic landscape).
…Biological oases typically occur in areas where resources (water, nutrients, energy) are locally more abundant, or where lethal environmental conditions (e.g., radiation, excessive temperatures) are somehow mitigated. Life signatures can be relatively diverse and abundant in those oases, but quickly vanish with distance or time. Often, biological oases are associated with specific substrates or physical environments (rocks, sediments, subsurface layers, fracture surfaces) whose chemical or physical properties provide a survival advantage to organisms.
(National Research Council, 2022. "Origins, worlds, and life: a decadal strategy for planetary science and astrobiology 2023-2032." : 396)
The 2022 National Academy of Sciences cite goes on to say that we can learn more about where oases are likely to occur so we look for life in likely places to find it. However since Perseverance isn't an in situ life detection mission, if there are biological oases in Jezero crater it will only sample them fortuitously.
So if we need a high level of assurance, we can't do safety testing even for bonus samples with no terrestrial contamination, because
- even if, improbably, most of the sample was destructively tested for biosignatures, we couldn’t rule out a viable spore perhaps imbedded in a crack in a dust grain in the remaining fraction of the sample.
We can’t know that unsterilized samples from Mars are safe for Earth until we have a much better understanding of Mars’s biosphere, if any, know what to look for, what its capabilities are and so on.
Let's look more closely at those two challenging scenarios in more depth using proposals for potential microhabitats and localized habitable regions on Mars.
Potential for ultra low concentrations of life from microbial oases in Jezero crater itself
More generally these could be biological oases for higher organisms too such as lichen or moss analogues. The National Research Council refers to the possibility of biological oases in an otherwise uninhabitable desert (National Research Council, 2022. "Origins, worlds, and life: a decadal strategy for planetary science and astrobiology 2023-2032." : 396) as we saw in the last section
This is also a theme of the Space Studies Board review of SR=SAG2.
We now have many suggestions for such oases, some of which are candidates for hard to detect small localized oases in Jezero crater itself, inhospitable though most of it is likely to be.
[FIXCITES HTML MARKUP]
- Caves - In the conference report from 2019, caves were one of the four top
priorities for a search for extant (i.e. current) life on Mars (Carrier et al, 2020, Mars Extant Life: What's
Next? Conference Report (html) :
Abstract) - this could be relevant to Perseverance's samples if hardy life could have got out of the caves to the surface of Jezero crater recently enough to still be viable, uplifted in thermals or through explosive volcanism and still be present on the surface for Perseverance to pick up (Carrier et al, 2020, Mars Extant Life: What's Next? Conference Report (html) : 10) [relevant to Jezero crater]
We will look at this in detail in the next section: Caves in Mars habitability studies: a major focus and the difficulty of detecting them from orbit or using rovers on the surface - Water condensing on microbes inside micropores in salts (from the Atacama desert
Mars analogues) :microbes in the Atacama desert can grow using water that condenses inside minute micropores in rock salt
(Wierzchos et al., 2012, Novel water source for endolithic life in the hyperarid core of the Atacama Desert) (Vitek et al., 2012, Microbial colonization of halite from the hyper-arid Atacama Desert studied by Raman spectroscopy) (Davies, 2014, The key to life on Mars may well be found in Chile). Microbes can also use micropores in gypsum, at an external humidity of only 60%, imbibing water at higher humidity, gradually becoming more desiccated below 60% humidity (Wierzchos et al., 2011. Microbial colonization of Ca‐sulfate crusts in the hyperarid core of the Atacama Desert: implications for the search for life on Mars : Figure 1)
[relevant to Jezero crater] - Microbes in a biofilm can extract water directly from gypsum by converting it to anhydrite (Huang, 2020. Mechanism of water extraction from gypsum rock by desert colonizing microorganisms) The authors found that in addition to the microbes in micropores, others were deep within the gypsum attached to a specific plane of the gypsum crystals, which may be more favourable for extracting water. They found that the microbes were associated with anhydrite and likely responsible for transforming the gypsum to anhydrite which would yield water as a byproduct. This was confirmed by culture experiments, the anhydrite formed only when blue-green algae were cultivated in dry conditions without access to water. The authors say this may “also provide insights into potential life in even more extreme environments, such as Mars” (Huang, 2020. Mechanism of water extraction from gypsum rock by desert colonizing microorganisms)
- ultracold brines (salty water) found by Curiosity in the martian tropical regions which might be habitable to martian life if it can find a way to hold onto water through to the warm midday temperatures in late spring.
These brines don’t depend on ice. The perchlorate salts take up water directly from the atmosphere when the air is ultra cold at night and reaches 100% humidity.
Curiosity detected these brines in Gale Crater even on the surface (0 cm) through to late spring, with enough water content for life and on the last day that it recorded them in spring, they were still there in the early morning through to 6 am. However they were far too cold for terrestrial life, at below -70°C (Martín-Torres et al., 2015. Transient liquid water and water activity at Gale crater on Mars : fig 3b). The intriguing thing is that later the same day, surface temperatures reached 288 °K = 15 °C (Martín-Torres et al., 2015. Transient liquid water and water activity at Gale crater on Mars : fig 2a).
Perseverance doesn’t have the ability to detect these brines, but they probably form in Jezero crater too, where they may be more stable than for Gale crater (Chevrier et al., 2020, Global Temporal and Geographic Stability of Brines on Present-day Mars : Figure 7).
Nilton Rennó suggested in an interview that microbes might use biofilms to inhabit these brines (Pires, 2015, Mars liquid water: Curiosity confirms favorable conditions)Rettberg et al. in 2016 (Rettberg et al., 2016. Planetary protection and Mars special regions—a suggestion for updating the definition : section 2.1), asked if multi-species biofilms could form on Mars and if organisms could replicate there if the temperature and water activity separately reach levels suitable for life, but not both at once, for instance by storing water when it is cold and then using it to replicate as the brines warm up? [relevant to Jezero crater]
- Fresh water as melting frosts - fresh water is stable against freezing and
boiling over 29% of the martian surface but isn't stable against boiling because the air is too dry
- if frost melts faster than its evaporation rate, the melt water can be briefly habitable in
“slopes facing the sun, under clear sky and calm wind conditions, at locations with low
surface albedo [dark patches] and low soil conductivity [if the soil is a heat insulator like ice] ” (Martínez et al, 2013, Water and brines on Mars … : section 2.1). Some experiments
suggest this could happen even with frosts in Jezero crater a few 10s of microns thick which may be
habitable for a few hours in the morning (Ramachandran et al., 2021, Experimental Investigation of the Atmosphere-Regolith Water Cycle on
Present-Day Mars). There are possible direct detections of frosts in Gale crater a few microns
thick (Gough et al., 2020, Possible Detection of Water Frost by the Curiosity Rover) and indirectly (Martínez et al., 2016. Likely frost events at Gale crater: Analysis from MSL/REMS measurements : figure 5) but both times, the daytime temperature was too cold for the frosts to melt. Perseverance hasn’t yet spotted frost in Jezero crater as of writing this, though it is attempting to search for it by various methods (NASA, n.d. Searching for Frost at Jezero Crater).
[potentially might be relevant to Jezero crater]
The Viking 2 lander (NASA, 1997, PIA00571: Ice on Mars Utopia Planitia Again) and Phoenix lander (NASA, 2008, Morning Frost on the Surface of Mars) both imaged daytime frosts on the surface.
(NASA, 1997, PIA00571: Ice on Mars Utopia Planitia Again)
In short, there is a remote possibility of temporary fresh liquid water from melting frosts in Jezero crater. There is a better chance that Mars might occasionally have short lived liquid fresh water from melting frosts in other more favourable conditions, which might then be a source of viable life in the dust storms (see next section). - Recurring Slope Lineae [uncertain]
[most of those are likely caused by dust processes according to the latest research, but there is still the potential for liquid water to be involved in the process so they remain uncertain habitats - may need in situ studies to confirm (Kurokawa et al., 2022, Can we constrain the origin of Mars' recurring slope lineae using atmospheric observations?) ]
[potentially might be relevant to Jezero crater as some potential RSLs were found in Gale crater] - Salts such as gypsum and bassanite can hydrate in present day conditions.
- There is significant potential to find a novel microenvironment in Jezero crater (similarly to Curiosity's surprise discovery of the ultracold surface brines)
Olsson et al. discuss the potential habitability of liquid brines in locations throughout Mars all the way to the equator, so this includes Jezero crater. These metastable brines may be liquid for up to six consecutive hours for some days of the year. Though inhospitable there is nothing yet to suggest they would be totally uninhabitable.
Extensive studies have shown that deliquescent salts, for example., sulfate, perchlorate and chloride within regolith, can absorb water from the atmosphere and form liquid brines through deliquescence and hydration (Ramachandran et al., 2021). These salts are abundant on Mars; for example, measurements from Phoenix Lander and MSL showed that known abundances of deliquescent salts constitute up to ~0.5 wt % of the martian regolith (Hecht et al., 2009; Bish et al., 2013; Glavin et al., 2013; Clark and Kounaves, 2016).
For example, gypsum and bassanite have been detected, which can hydrate under present day conditions absorbing atmospheric water (Vaniman et al., 2018). A recent study has also suggested that in analogue environments on Earth, microorganisms are able to extract water from gypsum (Huang et al., 2020). Experimental data have shown that meta(stable) brines may exist from the equator to high altitude for a small percentage of the year, up to six consecutive hours on the surface and in the shallow subsurface (Rivera-Valentin et al., 2020).
Although, more recent work has demonstrated that;
- 1) metastable brines can also exist for shorter periods in open systems on the source (supported by salts or a salt/mix regolith);
- 2) pure liquid water can form spontaneously and persist during evaporation during short periods of a few hours when the near-surface is saturated (e.g. Vakkada-Ramachandran et al. 2021),
there is still limited knowledge about the kinetics of phase transition (Rivera-Valentin et al., 2021).
Although the conditions of these metastable brines have been deemed inhospitable to life (Rivera-Valentin et al., 2020), the potential for these brines to be habitable cannot be ruled out, as discussed previously (Carrier, 2020).
On Earth, brines impact growth due to low water activity, high ionic strength, extreme chaotropicity, and/or multiple extremes (Hallsworth, 2021 and references within). Although this may hinder growth, there is no evidence that the conditions would kill/eradicate microbial life in certain brines, e.g., sulfate salts. Experimental work has recently shown that cells of Halomonas sp. str. HL12 survived and grew after several cycles of desiccation (Cesur et al., 2022).
(Olsson-Francis et al., 2023.The COSPAR Planetary Protection Policy for robotic missions to Mars: A review of current scientific knowledge and future perspective : 32)
[paragraphing and bullet points added for readability
Caves in Mars habitability studies: a major focus and the difficulty of detecting them from orbit or using rovers on the surface
Mars has the full variety of caves that can form on Earth as well as some novel types of cave such as caves from subliming carbon dioxide impossible on Earth (Boston et al., 2006, Location, location, location! Lava caves on Mars for habitat, resources, and the search for life) - and Mars has had active surface geothermal processes perhaps as recently as in the last 20 million years for the steam explosions recorded in the rootless cones (Stacey, 2019, Interactions between Athabasca Valles Flood Lavas and the Medusae Fossae Formation (Mars): Implications for Lava Emplacement Mechanisms and the Triggering of Steam Explosions).
The report of the 2019 conference "Mars Extant life: What's Next?" lists caves as one of the four top priorities for a search for extant (i.e. current) life on Mars
Based on our existing knowledge of Mars, conference participants highlighted four potential martian refugium (not listed in priority order): Caves, Deep Subsurface, Ices, and Salts.
(Carrier et al, 2020, Mars Extant Life: What's Next? Conference Report (html) : Abstract)
Caves, due to their confirmed presence (see Fig. 1), have been considered high-priority candidate environments in the search for extant life on Mars ... The cave environment has several advantages over surface targets, including protection from harsh weather, solar radiation, and desiccation.
Caves provide direct access to the shallow subsurface, where relatively stable temperatures and humidity conditions could persist over geological timescales, and host ice and salts that are other priority targets in the search for extant life. Additionally, caves on Mars may provide windows into the deep subsurface by virtue of the fact that several meters of rock may be present above the cave.
(Carrier et al, 2020, Mars Extant Life: What's Next? Conference Report (html) : 788)
Mars is still geologically active with numerous young fissure fed flood lavas on the Elysium Planitia from 500 to 2.5 million years ago. There is evidence of explosive volcanism 53 to 210 thousand years ago by crater counting (Horvath et al., 2021, Evidence for geologically recent explosive volcanism in Elysium Planitia). Horvath et al suggest that the Cerberus Fossae region may still be active today (Horvath et al, 2020. Evidence for geologically recent explosive volcanism in Elysium Planitia). So it's plausible some areas of Mars at least could be subsurface geothermal heating not far from the surface which we couldn't detect.
It's also possible that liquid water finds its way to the surface, or to caves in the near surface, even without localized geothermal heating. The liquid water might form in subsurface ice buried below the detection limit of our orbital radar of 500 meters, deep enough to be melted by the insulating effect of the surface rocks over the hot interior of Mars. It could get to the surface along fractures that form in impact craters. These run deep below the surface and parallel to the interior surface of the crater all the way to the top of the rim of a crater and in this way water could be lifted to the rim of the crater under hydrostatic pressure through artesian upward leakage. This idea is based on Abotalib's proposed model for the Recurring Slope Lineae in Palikir crater (Abotalib, 2019, A deep groundwater origin for recurring slope lineae on Mars).
[ILLUSTRATION MAY NEED PERMISSION - NATURE]
Illustration shows how water could travel from deep below the surface to the surface, at the rim of a filled in crater. This could explain how water would get to the top of steep hill slopes for the RSLs if some of them are caused by seepage of subsurface brines
(Abotalib, 2019, A deep groundwater origin for recurring slope lineae on Mars : figure 5)
Water can travel through these fractures more easily than through solid rock. Since they are fractures, they also seem plausible locations for fracture caves. Whether or not this modelling explains any of the RSLs, it also suggests a possible source of water for other subsurface fracture caves that may not be associated with any clear surface features, or indeed previously formed solutional caves or other caves. Water may be able to get into caves through fractures from the deeper subsurface. The water would rise to the surface because of the pressure of the overlaying rock.
For potential for life in the Perseverance samples, the main question is whether any caves in Jezero crater could eject viable life on the surface occasionally for us to detect in dust spread in the winds
Cave air is thought to exchange with the atmosphere, potentially enhancing the ability to detect such chemical indicators by remote means at their entrances
(Carrier et al, 2020, Mars Extant Life: What's Next? Conference Report (html) : 788)
Caves are important for forward contamination, but even more so for backwards contamination because there is potential for contamination that’s almost all one way, outwards from the cave. A cave with a small entrance might be at low risk for contamination by microbes spread in the dust from a rover on Mars, but if the cave environment is productive for life, and there are ways for life in the cave to reach the surface, it might be a major source of microbes that are spread in the dust storms. For instance Boston suggested the methane plumes could come from subsurface caves, perhaps formed originally in temporary hydrothermal systems after a large meteorite impact into subsurface ice (Boston et al., 2006. The microbial communities of sulfur caves: A newly appreciated geologically driven system on Earth and potential model for Mars)
Kevin Webster suggests we may be able to detect a life modified atmosphere from outside a cave. He found that terrestrial cave air has lower levels of benzene, acetonitrile, and dichloromethane compared to the atmosphere and higher levels of Nitrous oxide (N2O) all likely due to metabolic processes.
When thinking about caves on Mars, a biological signature would appear to be the removal of reactive components of the atmosphere
(Webster, 2019 . Developing cave air as a biosignature).
Wynne et al., also suggest that the cave entrance itself could be made more habitable for life than the surrounding surface, due to modifications from the presence of the cave itself saying that the boundary for habitability at the cave entrance depends on complex interactions with the interior processes of the cave.
Importantly, could a life-modified atmosphere be detectable from inside the cave or possibly outside the cave if the cave is exhaling constituents produced by life?
,,,
The cave environment is the boundary condition for habitability but is determined by the complex interactions of several physical processes (e.g., conduction, convection [transport through the atmosphere], and advection [transport by flow of a liquid]), surface and subsurface conditions, and the cave geometry.
(Wynne et al., 2022 . Fundamental science and engineering questions in planetary cave exploration)
That suggests the possibility that subsurface life itself could also be lofted onto the Martian surface from the caves. Microbes can be turned into aerosols in terrestrial caves as a result of splashing from streams, or fungal spores (Mulec et al., 2012 . Prokaryotic and eukaryotic airborne microorganisms as tracers of microclimatic changes in the underground (Postojna Cave, Slovenia) : 614). Perhaps martian fungi could also use airborne spores to propagate in caves and perhaps these could be lofted to the surface through the entrance in thermals. Also some may be ejected to the surface as a result of steam explosions similarly to those that formed the rootless cones (Stacey, 2019. Interactions between Athabasca Valles Flood Lavas and the Medusae Fossae Formation (Mars): Implications for Lava Emplacement Mechanisms and the Triggering of Steam Explosions) or explosive volcanism (Hamilton et al., 2010. Explosive lava ‐water interactions in Elysium Planitia, Mars: Geologic and thermodynamic constraints on the formation of the Tartarus Colles cone groups)– or through active fumaroles.
Though no active fumaroles are known today on Mars there may be some that are temporarily inactive or not yet detected because they are hidden by an ice tower. See next section:
These don't need to be active today. Fumaroles active in the past few millennia or tens of millennia could have lofted spores to the surface that are still viable today. We find spores like this everywhere on Earth, the "geobacillus paradox" that spores adapted to high temperatures can be found in all habitats on Earth in surprisingly high concentrations. See Supplementary information section under:
SR-SAG2 and the Space Studies Board review of it both discuss caves and agree that there is potential for forward contamination of them.
SR-SAG2 Finding 4-11: On Earth, special geomorphic regions such as caves can provide radically different environments from the immediately overlying surface environments providing enhanced levels of environmental protection for potential contaminating organisms. The extent of such geomorphic regions on Mars and their enhancement (if any) of habitability are currently unknown.
(SSB, 2015. Review of the MEPAG report on Mars special regions : 24)
The Space Studies Board review looks at the potential for life to survive in such caves and says they are best classified as Uncertain Regions, treated as Special Regions until proven otherwise
Although their number and sizes are largely unknown, caves and other subsurface cavities on Mars would represent environments with ambient conditions (e.g., temperature, humidity, exposure to radiation) that are very different from those at the surface, and most probably, those conditions are likely to be favorable for microbial colonization. Consideration of caves and subsurface cavities is paramount for two reasons. First, they provide a protected environment (e.g., from extremely low temperatures and radiation). Second, they can provide a means by which terrestrial contamination can access martian subsurface environments.
…
In conclusion, there could be a number of possible primary sources of the necessary ingredients for life inside caves and subsurface cavities on Mars, and therefore, they are best classified as Uncertain Regions and treated as Special Regions until proven otherwise.
(SSB, 2015. Review of the MEPAG report on Mars special regions : 24)
SR-SAG2 lists the possibilities as:
- Drained lava tubes
- Caves formed by tension fractures
- Caves in the salts left after evaporation of salty water – e.g. in gypsum
The Space Studies Board review adds:
- Caves formed by water running underground (like many terrestrial caves)
- Caves formed by mud volcanoes or material expelled by hydrothermal processes
It says:
Consideration of caves and subsurface cavities is paramount for two reasons. First, they provide a protected environment (e.g., from extremely l
ow temperatures and radiation). Second, they can provide a means by which terrestrial contamination can access martian subsurface environments. In addition to drained lava tubes, voids resulting from tension fracturing, and possible caves in evaporites (e.g., gypsum karst), there are types of subsurface cavities on Mars not mentioned in the SR-SAG2 report that may have been produced by subsurface erosion by water (analogous to piping; e.g., Higgins and Coates 1990) or by expulsion of material through hydrothermalism (Rodríguez et al. 2005) or mud volcanism (Rodríguez et al. 2012). However, to the best of the review committee’s knowledge, there is no data on the availability of water in martian caves.
(SSB, 2015. Review of the MEPAG report on Mars special regions : 24)
Penelope Boston added several other potential caves not mentioned by either SR-SAG2 or the SSB review: glacier caves, wind scoured caves, caves formed from water by moving fine particles instead of dissolving the rock, and caves formed by ice and dry ice subliming directly into the atmosphere. Many of these could be connected to the surface. These are the main types of caves she looks at (Boston, 2010. Location, location, location! Lava caves on Mars for habitat, resources, and the search for life):
- Solutional caves (e.g. on Earth, caves in limestone and other materials that can be dissolved, either through acid, or water)
- Melt caves (e.g. lava tubes and glacier caves)
- Fracture caves (e.g. due to faulting)
- Erosional caves (e.g. wind scoured caves, and coastal caves eroded by the sea)
- Suffosional caves - a rare type of cave on the Earth, where fine particles are moved by water, leaving the larger particles behind - so the rock does not dissolve, just the fine particles are removed.
- Mars could have sublimational caves caused by dry ice and ordinary ice subliming directly into the atmosphere.
She points out a few processes that may be unique to Mars, including that last category of sublimational caves. Amongst many other ideas she suggests:
- For the solutional caves, the abundance of sulfur on Mars may make sulfuric acid caves more common than they are on Mars. There's also the possibility of liquid CO2 forming caves (which forms under pressure, at a depth that could become exposed to the surface, e.g. in a cliff wall).
- For the melt caves, the lava tubes on Mars are far larger than lava tubes we find on Earth.
She talks in more depth about the possibility of sulfuric acid caves on Mars and suggests the methane plumes could come from subsurface caves, She also talks about sulfur caves as of special interest for microbial communities. She looks at the sulfur inhabiting microbial communities of caves such as Cueva de Villa Luz in Mexico as analogues of sulfur caves on Mars (Boston et al., 2006. The microbial communities of sulfur caves: A newly appreciated geologically driven system on Earth and potential model for Mars)
Snottites in Cueva de Villa Luz, a sulfur cave in Mexico which may be an analogue of similar caves on Mars. With its abundance of sulfur Mars may have many sulfur caves.
Though we have not yet detected geothermal heating on Mars, it has been volcanically active within the last few tens of thousands of years. So it's not impossible some are geothermally heated and able to host life and may be open to the surface.
(NASA, 2006, Cave Slime)
The solutional cave and rock fracture caves are particularly challenging to detect from orbit. (Boston et al., 2006, Location, location, location! Lava caves on Mars for habitat, resources, and the search for life). For backwards contamination what matters most is the potential for nearby undetected caves that might be able to spread Martian microbes to the samples in dust storms or dust devils.
On Earth many caves are not easy to find as with the example of the Lascaux cave paintings. Ravidat’s dog Robot got entangled in a toppled Juniper tree and he discovered the hole that lead to the cave as a result of going to his dog’s rescue. The toppled Juniper revealed the hole (Eshleman, 2008 . Lectures on the Ice-Age Painted Caves of Southwestern France)
COSPAR give this photograph as an example to show the problem.
Photograph from Curiosity sol 712, openings to the subsurface shown with arrows.
(Olsson-Francis et al., 2023.The COSPAR Planetary Protection Policy for robotic missions to Mars: A review of current scientific knowledge and future perspective : Fig 2)
There could be cave entrances close to our rovers and they may not spot them even driving right past them, if the entrances are small and hidden in shadows.
The 2023 COSPAR report said cave surface openings could plausibly have habitable conditions at a temperature of -25°C which could support metastable water even when not geothermally heated or supplied by warmer water from below:
However, despite several decades of martian exploration with orbiters and landers, and a detailed characterisation of the landing site, there may not be enough knowledge at relevant scales to inform planetary protection decision making resulting in the need for a tailored process or an adaptive risk posture.
The caves and subsurface openings on Mars remain primarily uncharacterised, although they are believed to be extensive (Sauro et al. 2020). However, within these openings the temperature is predicted to be approximately -25?C, which could support metastable water (Williams et al. 2010). On Earth, analysis has demonstrated that metabolically active microbial life is plausible (Carrier et al., 2020).
(Olsson-Francis et al., 2023.The COSPAR Planetary Protection Policy for robotic missions to Mars: A review of current scientific knowledge and future perspective : 31)
Also the surface of Mars is not completely unchanging. The sand dunes move slowly and sometimes sand slumps down on the hillsides which might reveal caves similarly to the way a toppled juniper revealed the Lascaux cave opening.
This shows a sand slump detected by Curiosity from the changes between the image on the left and the image on the right:
Text on Graphic: It would be easy to miss an existing small concealed entrance to a large cave
Changes like this could reveal a new cave entrance.
Slide 15 from (Vasaveda, 2015. Gale Crater Observations of Relevance to Planetary Protection)
A slump like that could reveal an entrance to a previously unknown cave which wouldn’t be revealed even if a rover photographed the area close up just before the slump.
In addition, a large subsurface cave with only a small entrance to the surface could exist in complex terrain such as shown in that photo and often found in Jezero crater or Gale crater and not be detectable even from close up (like the hole that lead to the Lascaux cave). It could be undetected not only from orbit but also undetected by the rover or even marscopters flying over and photographing from above.
It seems impossible to be sure that any region of Mars is free of caves unless it can be shown to be geologically impossible, but fracture caves from meteorite impacts can happen almost anywhere on Mars.
NASA discovered potential for current habitats for terrestrial life in Gale crater AFTER Curiosity’s landing: RSLs, most are likely dry dominated but some still could have a wet dominated mechanism
We have an example already of how our knowledge of a landing site can change after a rover lands. Like Perseverance, Curiosity is not sufficiently sterilized to visit regions where terrestrial life could spread. NASA thought Gale Crater had minimal risk for forward contamination as required for partially sterilized rovers like Curiosity. But then they discovered potential habitats for terrestrial life in Gale Crater after Curiosity’s landing (JPL, 2016. NASA Weighs Use of Rover to Image Potential Mars Water Sites).
These were possible RSLs, recurring slope lineae. These features grow down slopes in spring, broaden through the summer and fade away in the autumn on sun facing slopes on Mars. When first discovered in 2011, the favoured explanation was that they were the indirect result of subsurface brine seeps which could be potentially habitable (McEwen et al., 2011. Seasonal flows on warm Martian slopes).
More recent research strongly suggests most or all of the RSLs are caused by dry dust flows though this is not yet proven. This is some of the evidence that strongly favours a dry mechanism:
- Mars analogue wet streaks in the McMurdo dry valleys fade over multiple years while the streaks on Mars recur without fading year to year (Toner et al., 2022. Wet streaks in the McMurdo Dry Valleys, Antarctica: Implications for Recurring Slope Lineae on Mars ).
- The RSLs on Mars became more active after the 2018 global dust storm and though this is also consistent with a wet mechanism supplemented by dust, the observations seem more consistent with a dust mechanism (McEwen et al., 2021. Mars: Abundant recurring slope lineae (RSL) following the Planet ‐Encircling Dust Event (PEDE) of 2018 ).
- The apparent connection with hydrated salts now seems an artifact of the data processing (McEwen et al., 2021. Mars: Abundant recurring slope lineae (RSL) following the Planet ‐Encircling Dust Event (PEDE) of 2018 )..
- The RSLs are found in locations not consistent with groundwater discharge such as the peaks of mountains (McEwen et al., 2021. Mars: Abundant recurring slope lineae (RSL) following the Planet ‐Encircling Dust Event (PEDE) of 2018 )..
- Frosts and deliquescing salts can’t supply enough water to be the main mechanism (McEwen et al., 2021. Mars: Abundant recurring slope lineae (RSL) following the Planet ‐Encircling Dust Event (PEDE) of 2018 ).
- The way the RSLs grow in spring may be because of seasonal variations in wind speed, direction, and turbulence (Stillman et al., 2021. Evaluation of grainflow mechanisms for martian recurring slope lineae (RSL) ).
- They may be caused by dust and bouncing sand grains and triggered by the dust devils [like miniature tornadoes on Mars], most of which don’t produce tracks detectable from orbit (McEwen et al., 2021. Mars: Abundant recurring slope lineae (RSL) following the Planet ‐Encircling Dust Event (PEDE) of 2018 ).
- A close study of RSLs in three representative craters can’t confirm the dry mechanism but the authors favour it, because they are not correlated with the times of year for brines for the wet mechanism and two of the sites may not have enough humidity to form brines (Stillman et al., 2021. Evaluation of grainflow mechanisms for martian recurring slope lineae (RSL) ).
However, we don’t have the surface resolution yet to be able to investigate them in detail and we can’t directly test for humidity near the surface, as the effects on the atmosphere would be too small to detect with current capabilities (Kurokawa et al., 2022. Can we constrain the origin of Mars' recurring slope lineae using atmospheric observations?. ).
Curiosity is not sufficiently sterilized to investigate these streaks. This led to difficult decisions for the Curiosity team when they found out that there were possible RSLs near to where they planned to drive the rover. After discussion they made a tentative decision that it could approach within a couple of kilometers to image them but not study close up, just in case local martian conditions there were habitable to terrestrial life (Witze, 2016. Mars contamination fear could divert Curiosity rover ).
Of numerous candidates, only two were considered to resemble RSLs sufficiently for concern, the sites 12 and 13 circled in orange in this slide (Vasaveda, 2015. Gale Crater Observations of Relevance to Planetary Protection) (Dundas et al., 2015. Slope activity in Gale crater, Mars)
Slide 24 from (Vasaveda, 2015. Gale Crater Observations of Relevance to Planetary Protection)
Curiosity is currently exploring the region of the possible RSLs but hasn’t approached either of those candidates
(NASA, n.d. Where is Curiosity ) with the map from (Dundas et al., 2015. Slope activity in Gale crater, Mars) overlaid and the two most likely candidates for RSLs circled in orange.
Potential for distant microbial oases as sources of ultra low concentrations of viable spores in dust storms
We have many testable predictions for more distant microhabitats on Mars. None of these suggested microenvironments could be seen from orbit. These would need subsurface ice or other processes that are not possible in Jezero crater but may be within range for viable life transported in the martian dust storms on occasion.
Some are relevant to Jezero crater. Others would need subsurface ice or other processes that are not possible in Jezero crater but may be within range for viable life transported in the martian dust storms on occasion.
- Fresh water as subsurface melt water in polar regions (this is the main way melt water forms in Antarctica and calculations suggest layers of 10s of cms thick of trapped fresh water should form over large areas of the polar regions about 5 cms below the surface wherever there is optically translucent ice, even with surface temperatures on Mars as low as 180 °K (-93 °C) (Martínez et al, 2013, Water and brines on Mars: current evidence and implications for MSL : sections 2.2.2 and 3.1.2).
- Liquid water might form briefly from any exposed ice in the Hellas basin where even fresh water might be stable for up to a few hours. In Hellas basin the atmospheric pressure by one model it is 12.4 millibars and water would boil at 10°C (NASA, 2000, Making a Splash on Mars)
- fumaroles that are currently inactive or hidden by ice towers but may be sporadically
active: fumarole ice towers would be up to 30 meters high in the low martian gravity. The
terrestrial towers often collapse and reform over a timescale of decades (Cousins et al., 2010, Volcano-ice interaction as a microbial habitat on Earth and Mars)
Fumarole ice chimney on mount Eberus (Turner, 2012, GOLF 4-3-9 Antarctica Expedition 2012). Hoffmann et al. suggest its Mars analogue could be up to 30 meters high and up to 100 meters in diameter in martian gravity and may be hard to spot because the ice would mask its thermal signature from orbit (Hoffman et al., 2003, The ice towers of Mt. Erebus as analogues of biological refuges on Mars).
This is not meant as a complete list, it's just a list of seven suggestions I found that seemed of especial interest.
Here are a couple more examples not mentioned in that list.
Potential for distant Mars habitat common in Antarctica: Fresh water drilled by sun warmed dust and prevented from evaporation by an ice lid
Cryoconite holes are one of many proposed terrestrial analogue habitats for Mars that I found in the literature survey that have a strong rationale but has had little attention in the Mars exploration community. They are common in the McMurdo dry valleys in Antarctica, one of our closest Mars analogue habitats on Earth. Calculations show that they should also form on Mars but would be impossible to detect from orbit. They are of especial interest as a way that present day Mars could have small quantities of fresh water - but not that small, over the entire ice cap this and other ways of providing subsurface ice melt such as liquid water around embedded meteorites in the ice could provide a total of many thousands of cubic kilometers of fresh water on Mars.
Cryoconite holes form when dust blown in the wind lands on ice in Antarctica. Heated by the sun the dust gradually sinks into the ice and as it sinks, an ice layer forms above it making a miniature greenhouse heated by the sun. Sunlight gets through the transparent ice above it but the heat is trapped. Fountain et al. found with rough calculations that the cryoconite holes contribute at least 13% of the meltwater for Canada glacier in the McMurdo dry valleys (Evolution of cryoconite holes and their contribution to meltwater runoff from glaciers in the McMurdo Dry Valleys) Cryoconite holes provide a refuge for life in ice sheets, rich in microbes such as mats of various types of cyanobacteria, bacteria, algae, and higher life such as rotifers and tardigrades (Ice shelf microbial ecosystems in the high arctic and implications for life on snowball earth) The algal mats in the cryoconite holes are as productive as algal blooms in the polar seas (ibid, page 141). This shows how it works:
Two ice covered cryoconite holes on the left and sketch of how they work on the right (Zamora, 2018, Measuring and modeling evolution of cryoconite holes in the McMurdo Dry Valleys, Antarctica)
These cryoconite holes have been proposed as a way that life could survive and propagate in the polar ice caps on Mars, as well as possibly in near surface habitats on comets and Europa (Hoover et al., 2004, Microorganisms on comets, Europa, and the polar ice caps of Mars) (Corenblit et al., 2019, The search for a signature of life on Mars: a biogeomorphological approach : 14)
Ice boulders thrown onto the surface in 2021 from a formerly habitable site on the flanks of Olympus Mons
The executive summary, and my preprint, discus this remarkable photograph from NASA’s Mars reconnaissance orbiter of a crater that was detected by the small earthquake tremors it generated as it impacted on Mars by NASA’s Insight Lander.
(JPL, 2022, NASA’s InSight Lander Detects Stunning Meteoroid Impact on Mars)
Video: Flyover of Mars Impact Using HiRISE Data (Animation)
This crater threw up boulders from the subsurface of the Amazonis Planitia region on the flanks of Olympus Mons, the largest known volcano in the solar system which has been geologically active recently and is clearly not yet dormant. This is especially interesting for recent or even maybe present day life because lava flowed there less than 24 million years ago (Fuller et al., 2002, Amazonis Planitia: The role of geologically recent volcanism and sedimentation in the formation of the smoothest plains on Mars ).
Fuller et al. suggest that if here was life there at the time, perhaps living below the surface in subsurface groundwater at the time of the lava flows, it’s highly likely it erupted to the surface, where standing water formed briefly. Any standing water would freeze over rapidly and the ice evaporate into the thin atmosphere leaving traces of any life on the surface where we may be able to detect it still today (: The role of geologically recent volcanism and sedimentation in the formation of the smoothest plains on Mars. )
Since it is not yet dormant it may have potential for subsurface warmed habitats. There may be subsurface caves with life there even today.
Present day life could get there from below or it might reach the region as viable spores or propagules in the dust, dirt or bouncing dust grains.
Some of the dust or bouncing sand grains would fall on the ice boulders, and they would then be warmed by the sun. If they form miniature melt ponds they could melt their way down into the ice (called cryoconite holes), maybe to the point where the melt pond freezes over to trap liquid water similarly to the way the cryoconite holes freeze over in the McMurdo dry valleys in Antarctica, again like the suggestion for the polar regions of Mars. The sand grains might bring life with them.
Through to 2021 HiRISE observed 48 small impact craters or clusters in mid to high latitudes. The ice thrown up remains bright for months to years (Dundas et al., 2021: 12). The ice disappears more rapidly at lower latitudes. These impacts may also throw up pore filling ice which is impossible to detect from orbit as it darkens to the colour of the regolith in days (Dundas et al., 2021: 8).
Mars also has several exposed pole facing ice cliffs at low latitudes near equatorial regions, which also show that there is ice not far from the surface. This suggests that there is ice over much of the region that can be exposed in this way (Dundas et al., 2018) (Dundas et al., 2021: 4).
There might be enough ice exposed to sustain life that grows for as long as the ice persists, for months to years, then spreads to another crater and ice boulders in the dust with hardy long lived spores.
If there is life in these ice boulders, cryoconite holes or other distant potential habitats, and it's adapted to transport in the dust storms, they provide another potential source for ultra low concentrations of microbes in the Perseverance samples.
If all samples returned to Earth are sterilized the main biosafety challenge for NASA is to ensure the level of sterilization is adequate in an open, transparent and interdisciplinary way
We should be able to sterilize even unknown martian exobiology by using levels of ionizing radiation that will break the covalent bonds that hold complex chemicals together - 100 million years equivalent of surface ionizing radiation may be sufficient.
This needs expert attention and though the issues involved are far less complex than for a comprehensive biosafety plan, there is still potential for concerns from the public and of mistrust of NASA and infodemic so the same principles would apply of the need for a multidisciplinary approach, which would also help greatly in finding optimal solutions.
This needs research into the level of ionizing radiation needed. We could use high levels with almost no impact on geology as most of the samples will have had hundreds of millions or billions of years of surface ionizing radiation, well beyond any sterilizing dose for terrestrial life.
We can assume that the Martian surface receives a dose of 100 milligrays a year, to simplify the calculations, based on Curiosity’s 76 milligrays a year (Hassler, et al., 2014. Mars’ surface radiation environment measured with the Mars Science Laboratory’s Curiosity rover).
So, 10 megagrays corresponds to 100 million years of surface ionizing radiation assuming a rounded 100 milligrays a year (132 million years at 76 milligrays a year). Ionizing radiation has little effect on geology - salt crystals change colour but at doses far less than expected for even recently exposed materials on Mars. These colour changes happen at 30 megarads, equivalent to 0.3 megagrays (one Mrad is 10,000 Gy). the equivalent of 3 million years of ionizing radiation. There are no changes in crystal spacing and no changes in the ratios of isotopes for radiometric dating at this dose (Allen et al, 1999, Biological sterilization of returned Mars samples).
We likely need much more than 3 million years, but there is no reason to expect any change in isotope ratios or crystal spacing at higher doses either, and most or all of the specimens except the surface soil / dust / salts would likely have had far more ionizing radiation dose already.
It's possible that the geological effects also depend on the choice of frequencies for the X-rays, and may also be different if we use gamma ray sources like cobalt 60 to sterilize the samples, so we could make choices on how to sterilize the samples to minimize any geological effects. Then there's the effect of any oxygen in the atmosphere, of humidity in the atmosphere and effects of warmth combined with ionizing radiation.
Radiodurans can repair 200 double strand breaks in a 3.3 million base pair genome after 0.0175 grays of ionizing radiation. That is enough to break it up into fragments of less than 20,000 base pairs each. These experiments don’t give the microbe time to repair them as they are broken. Instead, after its chromosome is already broken up into 200 fragments, it can stitch them back together to restore the original chromosome (Minton, 1994. DNA repair in the extremely radioresistant bacterium Deinococcus radiodurans : Figure 2) updated figures, (Horne et al., 2022. Effects of Desiccation and Freezing on Microbial Ionizing Radiation Survivability: Considerations for Mars Sample Return. : 1340)
Radiodurans is one of our most radioresistant microbes, and when frozen and desiccated and populations are reduced a million fold with 0.14 megagrays(Horne et al., 2022. Effects of Desiccation and Freezing on Microbial Ionizing Radiation Survivability: Considerations for Mars Sample Return.) or 1.4 million years at 100 milligrays a year, which would lead to 1,600 double strand breaks, or 0.05% of the double strands leaving fragments only 2000 base pairs long.
Based on the data of 200 double strands broken out of a 3.3 million base pair genome after 0.0175 grays
- A microbe ten times more radioresistant than radiodurans would withstand 1.4 megagrays or 14 million years of surface ionizing radiation, which would lead to 16,000 double strand breaks, or 0.5% of the double strands leaving fragments only 200 base pairs long. [using the small numbers approximation that it’s a linear increase]
- 10 megagrays or 100 million years of surface ionizing radiation would break 3.4% = 100 × (1 - ((3.3 million - 200) / 3.3 million)10/0.0175 with fragments only 29 base pairs long.
We should be able to sterilize biological entities even if not based on terrestrial biology
It is believed that if such a biological entity exists, humans would be able to kill it (by the sundering of covalent bonds in a rigorous sterilisation process).
(Ammann et al., 2012, Mars Sample Return backward contamination–Strategic advice and requirements : 12)
The main question is, what is the sterilizing dose needed? There doesn’t seem to be much research on the levels of ionizing radiation needed to sterilize any present day life on Mars if it is of some completely different biology. Rummel et al. say that the bond breakage should be similar if it’s based on other than carbon-containing molecules
If there were a life-form on Mars based on other than carbon-containing molecules, the energies holding such molecules together would not be much different than those for proteins and polynucleotides.
Hence, bond breakage by heat or gamma radiation should be similar for Earth and Mars life-forms, and sterilization conditions for Earth microorganisms should eradicate microorganisms of similar size from Mars.
There is no absolutely optimal approach to decontamination under these circumstances, but enough is known about the relationships among organism size, repair mechanisms, and survivability, that the maximum survivability of any martian organisms can be estimated with some confidence.
(Rummel et al., 2002, A draft test protocol for detecting possible biohazards in martian samples returned to Earth : 10,)
Irrespective of the chemical basis of any life-form, a confidence level of sterilization can be provided with only two assumptions: 1) any reproducing life-form must be based on macromolecules (i.e., polymers) with interatomic covalent bonds (not crystal lattices), and 2) since all such bonds have similar strength, destroying these bonds destroys the life-form.
(Rummel et al., 2002, A draft test protocol for detecting possible biohazards in martian samples returned to Earth : 10, 13)
Let's just look briefly at that first assumption (not crystal lattices). There's an intermediate possibility, the question of whether we need to sterilize prion aggregates - which only repeat in one direction. They aren't alive but they are able to replicate in living organisms which provide them with a supply of proteins to add to them. There is a change since the 1997 report, we now know of prions in microbes in all three of the domains of life, archaea, bacteria and eukaryotes, so they likely also occurred in the last universal common ancestor. See the supplementary information under:
Prions are proteins in their minimum energy state which lets them form amyloid fibres based on β-sheets. These consist of lots of parallel proteins in a stretched out low energy configuration, bonded together by hydrogen bonds.
(Wickner et al., 2008, Amyloid of Rnq1p, the basis of the [PIN+] prion, has a parallel in-register β-sheet structure. 2008 : Figure 6) (PNAS license)
If we need to sterilize these structures we need higher sterilization doses than for DNA which needs a double strand break to break it up, or for protein polymers which can be broken into pieces by cutting them in one place.
Then for crystal structures themselves, there's Cairn-Smith's suggestion in his book from 1985 (Cairns-Smith, 1985, Seven clues to the origin of life: a scientific detective story) that life might have started based on a crystal lattice of clays and replicating clay sheets that form on the surface and then flake off, before it developed cell walls and cell processes. Clays could propagate as defects in one layer can lead to defects in the next (Plaxco et al., 2011, Astrobiology: A Brief Introduction: 127). Cairn-Smith's idea originally seemed promising as an idea, with a lot about it that is in common with the way that DNA works. However experiments don't seem to support it, though they led to other interesting ideas for ways that clay could have helped seed some of the processes of early life (Kloprogge et all, 2022, Clays and the origin of life: The experiments).
Cairn Smith's theory was to explain present day life, so it starts 2D and then shifts to a 1D polymer and the modern DNA helix. For a life-form that keeps its genetic information in a 2D lattice it would need to remain 2D or else start from 1D and shift to 2D. If alien life could find a way to achieve high fidelity replication from one sheet to the next in 2D, and to store its genetic information in sheets rather than in helixes, it would be a simpler form of replication, and with higher density of information so it might have advantages.
There doesn't seem anything to support this as a possibility. I'm just mentioning it for completeness here. Do we need to consider it? If we need to consider 2D lattices It would still be possible to sterilize alien life by breaking the bonds - but it would take a higher dose to break up 2D sheets into discontinuous molecules, especially so if they were stored in stacked identical sheets.
I cover this in my literature survey preprint, concluding:
I find little in the literature by way of attempts to estimate how more resilient a totally alien biochemistry might be, evolved to resist ionizing radiation on Mars for billions of years. We can surely find a dose that would sterilize even a mirror life microbe with a PNA backbone adapted to Mars for billions of years. Is 10 megagrays enough, or 100 million years equivalent?
This is something that needs attention from experts in the relevant disciplines such as synthetic biology, early life, etc.
(Walker, 2023. NASA must protect Earth's biosphere even if Mars samples hold mirror life : Section: "Sterilization of the samples must be effective for any conceivable exobiology – 100 million years equivalent would reduce DNA strands through double strand breaks to fragments only 29 bases long though alien biomolecules such as PNA might be more resilient"))
This topic needs to be looked at carefully, but it seems reasonable that experts can come up with a level of sterilization that everyone is confident will work. The aim is to find a level of sterilization we can have confidence in before we have any knowledge of whether or not there is life on Mars or what form it may take. Then there may be value in experimentation to find a method of sterilization that is also optimal for studies of the sterilized samples.
There are some disadvantages with ionizing radiation. First practical issues for using it in space.
- Cobalt 60 sources are heavy though much of the weight is for shielding which can be reduced if there are no humans on board
- X-ray emitter sources use a fair bit of power, less so with carbon nanotube emitters but still significant.
For details and a discussion of how we can overcome the issues see below:
The other disadvantage is that ionizing radiation does break up large molecules. This doesn't matter for rocks and deposits that have been exposed to ionizing radiation from the sun and cosmic radiation for hundreds of millions of years, but if we want to look at chemical processes and possibly life processes operating on Mars today, or if we manage to locate recently excavated past life it will be significant.
Ultraviolet light can't sterilize interiors, cracks or shadows, is greatly reduced by a single grain of dust and any martian life is likely to have evolved exceptionally high levels of resilience to it
Ultraviolet light may seem like an easy way to overcome the power and weight issues, especially if we can just use the UV in unfiltered sunlight in space for sterilization. However ultraviolet light is still light and not very penetrating, easily blocked even by a single dust grain for a microbe that happens to be positioned directly below the center of the grain or imbedded in a crack in a grain.
As Velbel at al. put it:
Although UV-C might be used to sterilize surfaces within the SRF, UV radiation is non-penetrative and therefore ineffective at sterilizing the interior volumes or shaded areas of samples
(Velbel et al., 2022. Planning implications related to sterilization-sensitive science investigations associated with Mars Sample Return (MSR) : S-124)
UV is completely blocked by a millimeter of rock (Rummel et al., 2014. A new analysis of Mars “special regions”: findings of the second MEPAG Special Regions Science Analysis Group (SR-SAG2) : 901).
(Velbel et al., 2022. Planning implications related to sterilization-sensitive science investigations associated with Mars Sample Return (MSR) : Table 1)
Dust grains are at most microns in diameter. However a single grain of dust of size of around a tenth of a millimeter or a hundred microns is likely to reduce the UV dose by several orders of magnitude for microbes situated below the grain, leading to two different populations, sterilized microbes on top of or between the grains and unsterilized microbes that happen to be below a grain.
It seems likely that, in this experiment, organisms located below grains would experience levels of UV flux several orders of magnitude lower than those experienced by organisms located on top of grains. This results in, effectively, two different populations experiencing two substantially different UV regimes.
(Godin et al., 2023. Absorption and Scattering of UV and Visible Light Through Simulated Martian Regoliths and Rock Samples : 5).
Carl Sagan raised the issue of a microbe imbedded in a grain of dust
While it is probably true that a single unshielded terrestrial microorganism on the Martian surface — even the most radiation-resistant variety — would rapidly be enervated and killed by the ultraviolet flux, this by no means applies to all contamination scenarios.
The Martian surface material certainly contains a substantial fraction of ferric oxides, which are extremely strongly absorbing in the near ultraviolet. In fact, apart from the ferric oxide identification, the red color of Mars clearly indicates major electronic transitions at short visible wavelengths. A terrestrial microorganism imbedded in such a particle can be shielded from ultraviolet light and still be transported about the planet. In addition, microorganisms generally do not distribute themselves singly, but tend to clump. While the peripheral organisms may be rapidly killed by solar ultraviolet radiation, microorganisms in the clump interiors may survive for long periods of time.
(Sagan et al., 1967, Contamination of Mars. : 8)
Billi et al made a similar suggestion:
… Our findings support the hypothesis that opportunistic colonization of protected niches on Mars, such as in fissures, cracks, and microcaves in rocks or soil, could have enabled life to remain viable while being transported to a new habitat
(Billi et al.., 2019, A desert cyanobacterium under simulated Mars-like conditions in low Earth orbit: implications for the habitability of Mars).
This is a different situation from a microbe just positioned below a grain of dust, it's a microbe that is inside a crack or microcave inside the grain of dust so would be significantly more shielded by smaller grains. I can't find any papers on this topic.
Also we can expect any native martian life adapted to survive transport in the martian atmosphere in wind blown dust to be highly resilient to UV. It has likely developed additional coatings, and be more resilient than any terrestrial spores, and may have developed specific UV blocking biomaterials to protect itself.
We need research on whether or not microbes can be protected by small dust particles before we can consider this option. This research doesn't seem to have been done, at least, I can't find any papers investigating the potential for survival of a single microbe imbedded in a grain of dust a few microns in diameter as in Carl Sagan's scenario. If anyone knows of such research please let me know.
The other possibility is of a cluster of microbes that are adapted to protect themselves from UV altruistically as for some terrestrial life. We looked at this above:
So we need to allow for sterilization not just of terrestrial life but of potentially far hardier martian life with extra UV protection and possibly also with an intrinsically hardier biology than terrestrial biology.
See also below:
Other sterilization methods: chemical treatment to reduce proteins to amino acids and nanofiltration of gas and liquid under pressure
The technique of chemical treatment would lead to a much more controlled break down of proteins leaving the amino acids intact. As for nanofiltration, that is one of the best of all in terms of minimizing damage as any molecules less than the maximum particle size can be let through undamaged. If we follow the recommendations of the ESF in 2012 the maximum size the nanofibers can let through is below 0.01 microns or 10 nanometers.
Velbel et al. describe two other sterilization methods that may be useful for the returned samples if they can be used with non terrestrial biology: acid hydrolysis and microwave-assisted hot acid extraction.
Acid hydrolysis breaks peptide bonds releasing free amino acids and inactivating any amino acid biopolymers
...
Thus, the proposed sample preparation sequence of solvent extraction followed by acid hydrolysis in 6M HCl at 100°C for 24 h, which converts bound organic polymers into free organic molecules, could inactivate any biopolymers in the sample extract, rendering it sterile. Further study would be needed to demonstrate the efficacy of alternative sterilization procedures.
Microwave-assisted hot acid extraction in HCl, formic acid, and acetic acid ... extraction in 6M HCl at 100°C for 24 h would lead to complete protein hydrolysis and biopolymer inactivation
(Velbel et al., 2022. Planning implications related to sterilization-sensitive science investigations associated with Mars Sample Return (MSR) : S-123)
Both of these work by breaking the bonds between amino acids in proteins and they would also break apart prions but don't damage the bonds internal to the amino acids. These work for terrestrial biology. However given that the internal bonds of the amino acids are not affected, how confident can we be that this would work with a novel independently evolved biology on Mars? This seems to need expert scrutiny at a higher level to see if the results for terrestrial biology can be general enough to apply to independently evolved life if that is what we find on Mars.
Another way to sterilize is by filtration. This has been used successfully in water under pressure but I haven't found any records of its use for air filtration. The 10 nanometer or 100 Angstrom limit of size that the ESF recommended in 2012 as the preferred size limit if it can be achieved at a reasonable cost, and anything larger than that needs review.
This is still large enough for complex molecules, even if that is the new size limit after a review.
- Why NASA can't rely on HEPA filters for the ESF recommendation to contain 100% of ultramicrobacteria
10 nanometers is still large enough for a complex organic molecule, for instance a DNA strand around 30 base pairs could stretch across from one side to another, but DNA molecules are only 2 nanometers in diameter, so, longer DNA molecules could get through a 10 nanometer pore. In more detail, the B-DNA double helix molecule which is the usual form in biology has a diameter of~20 Angstroms or 2 nanometers, and the distance between base pairs ~3.4 Angstroms or 0.34 nanometers, see (Marcey, 2003,, 2022. An introduction to DNA structure).
This is too small to let through the larger ribosomes used for translating messenger RNA into proteins, as these are 20 or 30 nanometers in diameter. They would need to be broken up first. But a 10 nanometer micropore could let through the ribozymes that may have been used in place of ribosomes by RNA based life.
To take an example, the Hammerhead ribozyme molecule is 30 base pairs of RNA but it is folded up and elongated in shape and is a little over 30 Angstroms at its widest or about 3 nanometers by direct measurement of the model provided by the RCSB Protein Data Bank using the online measuring tools and a little over 6 nanometers long (Anderson et al., 2013. PDB 3D model for: Active-Site Monovalent Cations Revealed in a 1.55 A Resolution Hammerhead Ribozyme Structure)
So it would fit easily through a 10 nanometers diameter nanopore and length-ways it would even fit comfortably through a smaller 5 nanometer diameter nanopore.
Measurement of the size of the Hammerhead ribozyme which could easily pass through a 10 nm or 100 Angstrom nanopore. (Anderson et al., 2013. PDB 3D model for: Active-Site Monovalent Cations Revealed in a 1.55 A Resolution Hammerhead Ribozyme Structure)
It may be possible to achieve 100% containment of 0.05 micron particles in water under high pressure. A 2020 review of the literature found several studies that achieve a million fold reduction or more of small viruses in water ( Singh et al., 2020. Nanofiltration technology for removal of pathogens present in drinking water : 3) That doesn’t quite meet the target but Singh et al. found one study using carbon nanotubes loaded with silver that achieved 100% removal of very small viruses such as the polio, noro and Coxsackie viruses (Kim et al., 2016. A nanofilter composed of carbon nanotube-silver composites for virus removal and antibacterial activity improvement) (Singh et al., 2020. Nanofiltration technology … : 3).
This experimental filter could keep out 100% of polioviruses and the poliovirus is only 0.03 microns in diameter (Hogle, J. M., 2002. Poliovirus cell entry: common structural themes in viral cell entry pathways). These filters for smaller nanoparticles for water treatment are easily damaged, through chemical and biological deterioration by aging, scratches by particle like substances, or fouling of the membrane (Singh et al., 2020. Nanofiltration technology … : 8)
For the ESF release of a particle of 50 nanometers or larger is not permitted under any circumstances. However they also have the requirement of less than one chance in a million of release of a particle of 10 nanometers or larger, which can only be relaxed if it is shown safe to do so after expert review. We might need to do nanofiltration down to 10 nanometers or less especially after a new size limit and level of assurance review.
However if the aim is a one-off filtration of the liquid for sterilization before transferring the filtrates to laboratories on Earth for analysis, then it doesn't matter if they are easily damaged and not very durable so long as the filters can be guaranteed to be intact for a single use.
This could also be combined with chemical treatment to break up proteins into amino acids.
We could use water to wash out any easily soluble small molecules and filter those first through the nanofilters. That could yield some interesting large complex organic molecules.
Then we can use chemical treatment to treat the filter residue and the remaining liquid from the sample specimen to reduce proteins to amino acids and then pump those through the filter at high pressure as a second filtrate fraction.
Then finally we can sterilize both the filter and residue with ionizing radiation and release that also from containment to return to Earth for the satellite or release from the labs if this is done on Earth.
In this way the chemical treatment to reduce proteins to amino acids can be made safe by using nanofiltration after the chemical treatment to keep out any larger molecules of alien biology that may be resilient to the chemical treatment used.
We might also be able to do this for gases, a suggestion from the 2022 MSPG2 study:
- Will it be acceptable (for backward PP) to filter gas through nm scale mesh and analyze outside biocontainment?
- Will it be acceptable (in terms of sample quality) to
filter gas through nm scale mesh and analyze outside
biocontainment?(Carrier et al., 2022. Science and Curation Considerations for the Design of a Mars Sample Return (MSR) Sample Receiving Facility (SRF) : S-235)
We could do this with the bonus atmospheric gases sample, filter it or some of it through nanofilters and return it to Earth. See:
We could use this potentially for gases too. Though nanopore filters are not currently used for air filtration, for this application, we only need a nanopore filter that can withstand single use to filter out air under pressure.
As with water there would be a first stage of nanofiltration pumped through under pressure, collected in a chamber that is initially a vacuum (just expose it to space if this is done in orbit). Then if there are any large volatile molecules that would be blocked by the filter, they could be treated to break up proteins if that can also be done with gases, to make the second filtered fraction and then finally use ionizing radiation on the residue.
This needs to be looked at by experts. Can a nanopore air or water filter be made that is
- free from defects to the extent that we can be confident it can't let through larger molecules?
- sufficiently robust for a single use without damage?
Assuming the answer to both these answers is "Yes" this technique seems promising as a method of sterilization that can leave molecules as large as ribozymes intact without any extra damage from oxidizing radiation or chemical treatment or UV radiation.
Then we can use chemical treatment to break down the proteins that didn’t get through the nanofilters in the first round and make these also safe by nanofiltration.
If NASA still plan for unsterilized samples this seems to need a new Mars sample return study and new size limit and level of assurance review
If NASA wishes to return samples unsterilized, much more is needed. We need to know the size limit, level of assurance and what we need to protect against. Before we can do this, we need to follow the ESF study’s recommendation, to review the size limit of particle to be contained, and the level of assurance
However before we can do this, we need to know what it is we might need to contain.
The last major review for a Mars sample return was in 2009 and the last size limit review in 2012. Both are over a decade out of date so we need a new sample return review before NASA can start on the path of an unsterilized sample return to Earth.
So:
- We also need a new Mars sample return planetary protection report to take account of the many advances in our understanding of Mars, of potential habitats on Mars, of Mars analogue terrestrial extremophiles, and of synthetic biology and the potential pathways for a second genesis of life.
- It also needs to broaden its remit and take account of some topics not previously considered such
as fungal pathogens of blue-green algae, mirror life, Lederberg’s two papers and the potential
that we could all be immunocompromised to an extraterrestrial pathogen, the potential that we might
have no defences against a new genus of fungi, the potential that our immune system might over-react
to extraterrestrial biology with an allergic response or with sterile inflammation, and various
other considerations that seem to be new to this review.
It would help if the next Mars sample return study uses an interdisciplinary approach and gets help from other agencies including synthetic biologists, epidemiologists and experts from the WHO, experts on fungal pathogens of microbes, on allergic reactions and immune responses, and other areas of expertise.
The next section looks at some of the topics that a new Mars sample return planetary protection study would need to look at.
Some of the topics from a literature survey to cover in a future Mars sample return study
[from section of the same title in the preprint: (Walker, 2023, NASA must protect Earth's biosphere even if Mars samples hold mirror life): which links to sections in the preprint for more details on man of the bullet points]
Based on the new material found in my literature survey, a new sample return study should consider many topics not previously considered in the major Mars sample return planetary protection studies from the ESF in 2012 (Ammann et al., 2012, Mars Sample Return backward contamination – Strategic advice and requirements), the Space Studies Board in 2009 (SSB, 2009, Assessment of planetary protection requirements for Mars sample return missions) and the Space Studies Board again in 1997 (SSB, 1997, Mars Sample Return: Issues and Recommendations (1997)).
Some of the topics here seem to be new to the planetary protection literature (e.g. the mirror life scenario, the potential for allergic reactions to life from Mars, and looking more closely at potential effects of an alien biology generally).
This is not likely to be a complete list of all the topics they need to consider. It is just a list of the main ones that turned up so far in my survey
The review of the potential for extraterrestrial pathogens of humans should consider examples such as:
- Aspergillus
- Tetanus
- Our immune response to a new genus of fungi as invasive as Aspergillus but with no pattern recognition capabilities to recognize it
- Allergic reactions e.g. to fungi from Mars
The review of whether life from Mars could affect terrestrial ecosystems and the Earth’s biosphere should look at:
- Fungal parasites of microbes including parasites of photobionts
- Prions
- Example of permafrost microbe with optimal growth temperature 25°C and capable of growth at human blood temperature
- Species sorting which could lead to species adapted to higher temperatures than currently found in the environment life is returned from
- Geobacillus paradox - the possibility that volcanic vents on Mars produce large numbers of hardy spores similarly to the geobacillus spores that then spread widely in the dust and may be found almost everywhere
A new sample return study should look at possibilities with no terrestrial analogue such as:
- Joshua Lederberg's two papers looking at the possibility that terrestrial immune systems have no defences against alien biology - and Claudius Gros's similar suggestion
- and the possibility that this extends to even microbial terrestrial life with no defences against alien microbes
- Effects of returning mirror life or other life with a radically different internal biology
- Possibility that martian life could have much smaller cells that take up nutrients faster and avoid protozoan grazing
- Life that might be better at photosynthesis than terrestrial life
- Life that might be better adapted than terrestrial life and spread rapidly
- Life that could cause problems similarly to nanoplastics and microplastics even if terrestrial and martian life are mutually mystified and don’t attack each other or defend against each other
When considering whether samples could contain life it should look at:
- Potential for martian life to make the Curiosity brines habitable through adaptations such as biofilms, perhaps covered with surface moss analogues similar to terrestrial desert mosses that use hair structures that swell when hydrated to block escape of water vapour and maybe micropores that emulate the micropores in salt pillars and stomata-like structures that close at times of lower humidity or in response to daylight
- New studies on transport of biofilms in the dust
- suggestion that even if microbial life can't get started today, life on Mars could propagate via biofilm fragments
- Potential for individual microbes to survive in cracks in dust spores
- Potential for microbes to survive in cracks in bouncing grains of dust up to half a millimeter in size
- Potential for martian life to evolve spores or other propagules with hardened outer shells to survive bouncing which can be propagated similarly to bouncing grains
- The suggestion that Mars has fresh liquid water seasonally in the polar regions similarly to the subglacial melt in Antarctica which should happen at surface temperatures of -90°C because of the way ice lets light through but insulates the melt water and protects from evaporation in a vacuum which leads to ice melting at half a meter depth in Antarctica - in the different conditions on Mars it should melt at a depth of 5 cms
For the idea of testing samples before release it should consider:
- Testing can't protect Earth from life in a sample - that after 10,000 dust grains tested destructively you can't deduce that the 10,001th grain is safe
For human quarantine:
- Human quarantine can't work to protect Earth or even humans - issue of life-long symptomless superspreader like Typhoid Mary and that technicians might happen to be immune to a new fungal pathogen which most of the population have no immunity to
- if a technician got ill with a potentially life threatening disease, ethically you can't leave
them to die in quarantine they would be removed but that's the very time when they need to be kept
in to protect Earth
Impossibility of keeping out a pathogen of crops or microbes with human quarantine - it can become part of the human microbiome as with the example of the crop pathogen brought to the ISS - Impossibility of keeping out mirror life with human quarantine
A Mars sample return study
- shouldn't be set up with a remit to find a way to return a sample safely
- should be tasked to evaluate whether or not current technology is able to contain the samples to the required level of assurance, with “no” as a permissible answer
A Mars sample return back contamination study should be empowered to look at reasonable alternatives such as
- sterilizing all samples before they are returned to Earth,
- returning some samples of especial astrobiological interest to a satellite above GEO for study remotely, and sterilizing anything returned from that satellite to Earth.
The study could also benefit from a section looking at the benefits for Earth and space exploration of a completely alien biology, in a future where life can never be returned safely to Earth. This can encourage private space and governments to work together to accelerate the process of finding out if there is life on Mars. The search could be greatly accelerated with the interest and support of private space,
Literature survey identified a need for a broad inter-agency and interdisciplinary approach for a thorough updated Mars sample return study
This book has identified a need for an interdisciplinary approach for planetary protection for a Mars sample return. For a thorough investigation of the topic, astrobiologists need to reach out to those with other areas of expertise including:
- synthetic biologists
- researchers into early life
- epidemiologists and experts in infectious diseases - the WHO and the CDC should be involved in any reasonably comprehensive Mars sample return study, and they wouldn’t have missed the issue of symptomless carriers
- Experts on fungal pathogens of microbes,
- Experts on allergic reactions and immune responses
- Experts on the potential for improving on terrestrial photosynthesis and nitrogen fixation to consider whether extraterrestrial life could do the same.
- Experts on air filter technology
- Many other disciplines.
Then they need to follow up leads these experts suggest and suggestions from the general public as it is not easy to do a comprehensive study of the potential risks for extraterrestrial life from another planet.
We know of many possibilities for extremophiles, for microhabitats on Mars and so on today in the 2020s that wouldn’t even be considered in the 1960s. So we also need to give some attention to the possibility of future developments in science and our understanding of Mars to bring up even more possibilities in the future that we are not yet aware of.
We also need to give attention not just to developments in the size limit but also in the level of assurance. To keep focus on the main points relevant to the NASA mission plan, this is in the Supplementary information section.
Disciplines needed for Interagency Panel for adequate safety assurance for Earth's biosphere for a plan to return unsterilized samples
This book has identified many issues that would have been spotted immediately if there was anyone on NASA's team with the relevant expertise. This makes the PEIS a valuable and instructive basis for an expanded list of disciplines for an Interagency Panel or Review Committee for any similar plans.
If we wish to protect Earth's biosphere adequately plans, then based on the issues identified here we need expertise in, at a minimum:
- Planetary protection for a Mars sample return mission - this topic has an extensive literature spanning decades, and anyone familiar with it would have alerted NASA to most of the issues identified here immediately including the rebuttal of the Mars meteorite argument in both of NASA's main cites for it
See: NASA's central finding that any harmful life can get here better protected in meteorites is rebutted by BOTH of its main cites for it
Also: most of the sections of this book - Risk assurance - would have insisted on a campaign level target probability and would not have accepted the reasoning from a low risk of significant impacts to a conclusion that ecological impacts would not be significant.
See: NASA's novel deduction that there would be NO significant ecological impacts, based only on its assessment that samples are UNLIKELY to pose a risk of significant ecological impact
also: NASA clarified that the Mars Sample Return mission has no campaign level probability target for containment but this is not mentioned in the text of the PEIS - Studies on martian habitability and liquid water - would have alerted NASA to the most recent research on microhabitats, cryptic habitats, transport of biofilm in dust storms and so on,
See: Potential for ultra low concentrations of life from microbial oases in Jezero crater itself - Life detection instruments for astrobiology - would have alerted NASA to the rapid recent progress in instruments able to do in situ life detection as far away as Europa (are many teams working on this topic area)
See: Modern miniature life detection instruments that could fly to above GEO, or to Mars - Panspermia - would have alerted NASA that only the most hardy terrestrial microbes can travel between planets on meteorites and that the meteorites we receive from Mars come from many meters below the surface where conditions are likely cold, dry and inhospitable almost everywhere
See: Some of the many subtleties to the Mars meteorite argument that make it far harder for extant life on Mars to get to Earth - Invasive species - would have alerted NASA to the issues with species that can't get here on meteorites
See: If b. subtilis is like Canada geese in a popular analogy for martian microbes, other scenarios for martian microbes may be like Starlings, which can be invasive because they can't cross the Atlantic - Infectious diseases - would have immediately spotted the omission of soil pathogens with no adaptations to an infectious lifestyle in any organism in the example list of infectious diseases
See: NASA's Biological Safety Report's finding of near zero risk that martian life can harm us rebutted by soil pathogens like tetanus and aspergillus fumigatus not adapted to any terrestrial host - Epidemiology - would immediately ask awkward questions about the quarantine period - Sagan's insoluble vexing question, and about lifelong symptomless superspreaders similar to Mary Mallon
See: Even the most well prepared labs can have lab leaks - Sagan's insoluble "vexing question" of the quarantine period for a novel alien pathogen
Also: No quarantine period long enough to quarantine a "Typhoid Mary": a life-long symptomless carrier of extraterrestrial biology such as martian soil fungi or mirror life - Biosafety design and testing - would have immediately alerted NASA that HEPA filters can't contain ultramicrobacteria, that we have no technology currently available or in development to do so, and that there are major issues with testing to see if such filters work, replacing them and durability of any filters that might fulfill that requirement
See: Why more is needed than "NASA does not concur" for the issue of ultramicrobacteria containment
Also: Why NASA can't rely on HEPA filters for the ESF recommendation to contain 100% of ultramicrobacteria - Alien biology, early life and synthetic biology - would have alerted NASA to the potential for mirror biology on Mars. These disciplines are also essential for questions such as the potential for martian life that overlaps the range of terrestrial life but can also live in colder or drier or saltier conditions, establishing a size limit adequate for alien living cells that may be smaller than any terrestrial life, potential for life that is more resilient to Mars surface conditions than any terrestrial life and able to spread in dust storms, and establishing an adequate level of sterilization for alien life that can be assumed to have far higher tolerance of ionizing radiation than terrestrial life. For some examples of where these areas of expertise would be needed,
See: Mirror life as a potential astrobiological surprise like the unexpected carbon dioxide geysers for geology
Also: If all samples returned to Earth are sterilized the main biosafety challenge for NASA is to ensure the level of sterilization is adequate in an open, transparent and interdisciplinary way
Also: ⸽ Exploring Joshua Lederberg's suggestion of a risk to human health from alien biology through terrestrial analogues such as fungi, microplastics, and BMAA and following sections in Supplementary information
Also: A new size limit review could look at recent research into the very tiny ribocells in Supplementary information
Also: Researchers into synthetic life recommend containment requirements several orders of magnitude more stringent than a BSL-4 for their research in Supplementary information - Bioethics and law - would never have missed the numerous issues in complying with the NEPA guidelines
See: ⸽ How NASA's current PEIS fails many central NEPA requirements in Supplementary information - Communication with the public and dialogue with the public
- such experts would have drawn NASA's attention to public concerns at a far earlier stage and we would not have seen these many unanswered questions and unresolved issues raised by the public in both rounds under NEPA because the plan would have already taken account of them and resolved most of them through extensive public dialogue before the process started.
See: Recommendation of Mars sample return studies to involve public in risk governance as soon as possible, and NEPA requirement for interdisciplinary approach integrating social with natural sciences
This list is likely incomplete but covers the most important issues identified in this book.
The charter for the Interagency Panel that NASA set up in 2000 listed agencies that must take part but didn't list areas of expertise in any detail. The only disciplines mentioned specifically in the charter are: bioethics, law, public attitudes and the communication of science, the Earth’s environment, or related fields (SpaceRef editor, 2001, Planetary Protection Advisory Committee Charter).
This list is a complement to the charter. Some of these disciplines, for instance panspermia, alien biology and synthetic biology may need experts from outside any US agencies. Others could be missed depending on the selection of representatives. For instance it is essential to have expertise in all of: epidemiology, infectious diseases and biosafety design and testing in the representatives from the NIH or CDC.
One early task would be to identify whether there are any disciplines that they lack that need to be added after reviewing the literature and the plans. Also it would seem to be important to have a mechanism to recruit new experts to help write sections and chapters of the report or review. For instance experts on biosafety containment may say they need more specialist expertise on air filter and air incinerator design and testing to look adequately at the prospects for developing the technology to contain ultramicrobacteria.
For details of the charter for the interagency panel see above::
Simplest approach to leave next study of planetary protection for an unsterilized sample return study until after a thorough in situ search for life on Mars
This preliminary survey was NOT comprehensive and just suggests some of many areas that would need to be looked at. It suggests that a comprehensive study would be a significantly more challenging task than for the last comprehensive survey 2009, because of a much more complex understanding of Mars and the amount of research that has been done since then on relevant topics that would need to be considered.
However if we sterilize any samples from Mars that are returned to Earth this greatly simplifies the work that needs to be done to have the scientific background to make the decisions we need to make at this stage. This doesn’t need a comprehensive backwards contamination study - but it still needs care and attention, to look into the level of ionizing radiation needed to sterilize samples sufficiently to protect Earth. It also needs careful attention to communication with the public and it still needs a broad based interdisciplinary approach to make sure we choose a level of sterilization that is adequate even for an unknown alien biology.
We should be able to sterilize biological entities even if not based on terrestrial biology. See above:
This is something we would need to look at carefully when deciding the level of ionizing radiation needed or other means of sterilization.
More details on all this in the preprint.
My preprint with preliminary literature survey:
The preprint is here, with headers of sections as mini-abstracts so you get a good first idea by reading just the top level headers.
This includes my literature survey which goes into far more detail on many of the topics covered in this book.
A small step for NASA, a giant leap for planetary protection: the case for a miniature telerobotic lab above GEO
My suggestion is just one solution, which I also mentioned in the final comment on NASA's draft PEIS (Walker, 2022, Comment posted December 20th). In this way you can move forward from this PEIS with a new approach that has many advantages over NASA's current plans.
The advantages of returning to a safe orbit, compared to returning samples to a laboratory on Earth are:
- removes the two mission-critical roadblocks – it may not be possible to return to a ground based laboratory at a reasonable cost and with a reasonable timescale
- retains NASA's world-leading role in planetary protection,
- be an excellent example that’s easy for other space agencies and private space to follow to keep Earth 100% safe - they can either just sterilize their samples - or they can return to the satellite above GEO
- It could be the seed of a growing “miniature space station” but of small modules a few meters across staffed by small telerobotic handlers and miniature instruments rather than humans.
- It could include a very excellent Mars simulation chamber using a centrifuge to simulate martian gravity with windows that let in appropriately filtered sunlight, simulating a day night cycle inside which could be used for testing terrestrial organisms in sealed containers similar to the BIOMEX experiment in Mars surface conditions, or to study Mars surface conditions, or to reproduce Mars surface conditions to try to get any returned life to grow in the satellite.
- likely has less upfront cost this decade – because a small satellite in GEO at $150 million is likely to cost less than a ground based Mars Receiving Facility at half a billion dollars
- may not even add to the mass for the Earth Return System because there is no need for the aeroshell compensating for the need to get into a safe orbit in the Earth-Moon system
- No need to break the chain of containment with Mars greatly reducing the complexity of the sample return from the surface of Mars
- the suggestion for small bonus samples gathered by the ESA Sample Retrieval Lander in a clean container would greatly enhance the science return for astrobiology.
- Achieves far more science return, especially for astrobiology, because of the bonus samples
- Samples can be kept clean and returned to a sterile environment in Mars surface conditions unaffected by transport through Earth’s atmosphere (the capsule and tubes are not leak proof and the Earth’s atmosphere would seep into them)
- Has far less legal complexity and can be done with a simple NEPA statement similarly to sample returns from asteroids and comets
- Stimulates universities and other research institutions to work on novel miniature life detection instruments such as SETG to be space certified and send to the orbital telerobotic lab - which will then be exceptionally useful for in situ exploration on Mars - these instruments are also likely to have many terrestrial applications
- Prepares the way for future inspiring missions to Mars Also of great value for future astrobiological exploration of other locations in the solar system - such as to study samples returned from Ceres, Europa's ocean, Enceladus, Titan and so on
Then, we could just leave the samples on Mars and do in situ life detection on Mars instead. We do have this capability. NASA plan the Mars Life Explorer (Williams, 2021. Mars Life Explorer ) which will search for life signatures but is not very ambitious for in situ life detection. Dirk Schulz Mackuch has suggested focusing on in situ active life detection, attempting gene sequencing, testing for respiration similarly to Viking and so on, since the sample return mission seems stalled (Schulze Mackuch et all, 2024, How to get Mars life-detection missions back on track).
However there are great benefits for returning samples for life detection, with instruments we can’t send to Mars to a life detection lab as close as above GEO.
Compared to in situ study on Mars:
- Experiments can be far more massive than for life detection on Mars for the same cost
- Experimenters can control the experiments in real time with only a fraction of a second return trip latency
- A new experiment can be sent to the satellite quickly at low cost
- It’s possible to send spare parts and repair instruments telerobotically
- Easy to return sterilized samples to Earth, headspace gases and sterilized samples of martian life if any is found
- Adds a very excellent Mars simulation chamber using a centrifuge to simulate martian gravity with windows that let in appropriately filtered sunlight which can be used to study the martian surface conditions experimentally
- Gives universities a way to test instruments they can later send to Mars
- Gives a safer alternative to laboratories on Earth for samples returned from Mars
Many ways for the Titanic to avoid an iceberg: why NASA should listen to other scientists and the public for its biosafety plans to protect Earth from Mars samples
FIXCITES
There were issues with the levels of sterilization already by 2017, when NASA's first planetary protection officer John Rummel referred to issues with NASA's permitted levels of terrestrial contamination of the samples as a potential bureaucratic “train wreck”
If the issues aren't resolved, Rummel says, the rover could be headed for a bureaucratic "train wreck".
(Voosen, 2017, With planetary protection office up for grabs, scientists rail against limits to Mars exploration)..
The issue of sample tube contamination levels was also one of the last things the Planetary Protection Subcommittee tried to raise with NASA just before they were disbanded, See (NAC Science Committee, July 25-27, 2016: 9).
John Rummel’s image of a train wreck brought to mind the iceberg that sunk the Titanic. Here the iceberg represents public and scientific opinion in 2033 when they find out that
- NASA hasn’t prepared scientifically credible plans to protect Earth and its plans weren't prepared with the help of the CDC, NIH, HHS, DoA etc and
- the samples NASA returns will likely be valueless for the past life searches (and probably for present-day life too) because of the permitted levels of terrestrial contamination.
There may well be not just one way, but many ways to solve these issues, just as there were many ways the Titanic could have steered to avoid the iceberg - but the sooner we start work on looking for solutions, the better, for NASA, for planetary exploration science, for astrobiology and for the public.
Text on graphic: What we need to prevent in 2033 - NASA’s mission plans - NASA’s future science credibility - Public and scientific opinion - No scientifically credible plans to protect Earth - Samples likely valueless for astrobiology because of permitted contamination by Earth life
(NASA logo )
For the issues of contamination see above:
NASA may get many more suggestions if it opens up dialogue with the wider community of other agencies, international organizations and scientists in other countries, and the general public globally. NASA benefits and we all benefit.
Timetable tight to complete a sample return to a ground based lab before the 2040s; while ESA and NASA can achieve considerable cost savings for their sample retrieval spacecraft launched this decade with an early decision to return them to a life detection lab above GEO
[ADDCITES / FIXCITES]
Whatever we decide, whether to return to a terrestrial lab with better containment than a BSL-4 or to return to a miniature life detection lab above GEO we need to make this decision with the minimum of further delays.
We see this warning in the literature on planetary protection repeated over and over for the last decade and more. They always say that the planning for the Mars Receiving Facility needs to start right away.
As some of these steps may take time or involve unforeseen difficulties, this cannot be done soon enough.
Owing to the higher probability that Mars may currently have or may have had its own ecology (compared to the Moon), it is important to avoid repeating past mistakes and to prepare as much as is practical. This includes beginning the timeline as soon as possible so that any complications or bottlenecks can be worked through without impacting the launch schedule.
(Uhran et al., 2019, Updating Planetary Protection Considerations and Policies for Mars Sample Return : 6)
First for the terrestrial lab. Assuming it's agreed that a BSL-4 is not adequate to contain the samples, then the timetable is very tight indeed.
If NASA do a Mars Receiving Facility with the precautions just as recommended in the 2009 report it is already is too late to start, for it to be ready by 2033. It would have been better to start on it a decade ago.
The estimate cost based on the 2009 requirements was over five times the cost of a BSL-4 and with a timeline to complete it of 12 to 16 years, with another estimate of 11 years including an extra two years to prepare the technicians. So if we started today it would be ready to receive samples by 2034 onwards.
As an example the iMars working group estimate of 12 years to have the Mars Receiving Facility ready. The timetable starts with funding already in place. It is obtained like this :
- … Year X negotiate staffing of Institute Council and initiate searches for SAB, BCB, and PAB members
- SAB = independent Science Advisory Board
- BCB = Back Contamination Board
- PAB = Public Advocacy Board
- … Year X+1 initiate search for Facility Director
- … Year X+1.5 initiate searches for Science, Curation and Safety Heads
- … Year X+2 initiate searches for key personnel required for SRF design (multiple positions)
- SRF = Sample Receiving Facility
- … Year X+3 Begin design of SRF, including preparation of draft protocols for preliminary examination of samples, needed to design facility (allow two years based on Lunar Receiving Laboratory experience). Site selection process commences for the SRF
- Year X+5 SRF design in place
- Year X+6 Begin SRF construction (allow two years, based on BSL-4 experience, but may be less or more)
- Year X+8 Begin SRF analytical laboratories construction (allow one year); begin analytical instrument selection process (this should be left as late as possible to ensure cutting edge facility)
- Year X+9 Install and carry out specifications testing on laboratory instrumentation
- Year X+9.5 Carry out verification and validation of facility and laboratories
- Year X+10 SRF completed and “ready” to receive samples; carry out operational readiness testing
- Year X+12: Mars samples delivered to SRF
(Haltigin et al., and iMARS Working Group, 2018. iMARS Phase 2: a draft mission architecture and science management plan for the return of samples from Mars : .S-72)
[Red text for acronyms added]
From beginning the facility design, year X+3 to completion in year X+10 takes seven years. This assumes only two years to construct the facility, from year X+6 to year X+8, so that part of the timetable is similar to the timetable to construct a standard biosafety level 4 lab.
The three years from X to X + 3 are to get the team together and the scientific advisory boards and ensuring public participation which is so important to this mission.
The two years from X+10 to X+12 are to ensure that the technicians are thoroughly familiar with the new design to avoid the many lapses of containment in the lunar receiving laboratory for Apollo.
NASA have not yet reached year 1 in that timetable.
We also need to have the review of planetary protection for a Mars Sample Return mission and the review of the size limit and level of assurance because as we've seen so much has changed in the last decade. The reviews from 2009 and 2012 are not adequate for planning planetary protection for a mission a decade later. Assuming these are done independently, they could be done after the start of the timetable but need to be completed before year X+3 when the design work starts.
Then we need to allow for the extra requirements to contain ultramicrobacteria which is sure to add to the timeline and cost and complexity over the iMars timetable.
- Why NASA can't rely on HEPA filters for the ESF recommendation to contain 100% of ultramicrobacteria
- Why more is needed than "NASA does not concur" for the issue of ultramicrobacteria containment
- Air incinerators are not certified to contain alien ultramicrobacteria yet - could they be a solution?
Then we need to consider the potential for additional requirements as a result of the planetary protection review and the size limit and level of assurance review, possibly need to contain smaller organisms if alien life can be smaller than terrestrial life and at a higher level of assurance.
Then we need to allow for time and costs to resolve the issue that we can't contain mirror life or fungal pathogens and other soil pathogens with quarantine precautions for lab leaks.
Adding the 12 years of the iMars timetable to 2024 takes it to 2036. However those extra requirements to contain ultramicrobacteria and to be safe against accidental lab leaks which can't be contained with any known protocols or quarantine period would seem to take it to the late 2030s to 2040s as the earliest time to complete a ground based facility, given the need to develop novel technology.
Also such a facility will be so novel it seems not certain that we will be able to develop this technology at a cost that NASA will consider reasonable for the project. Probably the surest way to resolve all these requirements is in a completely telerobotic facility given the lab leak issues and possibly increased requirements for level of assurance and reduced size limit after review. But this may or may not be easily affordable depending on what is required.
This book sketched out one idea for a fully telerobotic future proof terrestrial lab. It is just to illustrate a way that might be possible though this particular approach would be a major challenge to build:
Timing is simpler for the proposed alternative to return samples to a life detection lab above GEO, though still tight to aim for 2033. The Technological Readiness Level seems higher as we saw above.
Then as another simplification, we don't need the review of planetary protection or a size limit or level of assurance review.
We still need a review to establish sterilization procedures suitable for any conceivable alien life for any samples to be returned from the satellite but this doesn't need to be completed until the 2030s and doesn't affect the design of the spacecraft we send to Mars. See above:
This level of sterilization review is also needed for the terrestrial lab for any samples removed from it as it doesn't seem that the samples can ever be shown to be safe to release unsterilized without more in situ study on Mars as we saw above in
- Why the COSPAR Sample Safety Assessment Framework can't be used to prove safety of unsterilized Perseverance samples
[and following sections]
The main spacecraft architecture changes we need for the life detection lab above GEO are for the Mars Ascent Vehicle and Orbiting Sample container (NASA, n.d., Mars Ascent Vehicle)., the Earth Return Orbiter (NASA, n.d., Earth Return Orbiter). and the Sample Retrieval Lander (NASA, n.d., Sample Retrieval Lander), due to be launched in the late 2020s though an independent review suggests they can't be launched until 2030 at the earliest (Figueroa et al., 2023. Mars Sample Return (MSR) Independent Review Board-2 Final Report : 16)., It would also mean changes to the Capture, Containment, and Return System (CCRS) and the Earth Entry System (EES) sent to Mars and then brought back to Earth in the ERO (NASA, n.d., Earth Return Orbiter).
The designs of those can be considerably simplified for a return to above GEO.
- No need to break the chain of containment with Mars as the sample container won't be returned to Earth, which simplifies many things for all three vehicles. We can focus the technology on protecting the samples from terrestrial contamination instead, especially the bonus samples. No need to sterilize any martian materials on the exterior of the Orbiting Sample container sent into orbit by the Mars Ascent Vehicle.
- No need for an aeroshell for the Earth Return orbiter. This gives it more payload for fuel for the journey back which as we saw could let it maneuver itself into the orbit above GEO instead of needing to be caught by a net or some such.
- Do need to add the ability to collect bonus samples to the ESA Sample Retrieval Lander
- Do need to add extra fuel so it can get into an Earth capture orbit though the extra amount will be small due to its use of an ion thruster and the existence of minimal energy transfer orbits and we have saved the mass of the aeroshell which saves fuel.
- It already will have the ability to navigate to rendezvous with the Orbiting Sample container so it can rendezvous with the life detection lab but we could add capabilities to make it easier to transfer the Orbiting Sample container to the life detection lab above GEO.
For the return to above GEO, the work on the life detection lab above GEO can start now, such as the centrifuge for martian gravity, and the method for robotic docking with it, but it won't need to be ready to launch until the late 2030s.
With this approach then a target date of the early 2030s may still be achievable.
There are two reasons why we need to resolve these issues as quickly as possible if we end up with the solution of a life detection satellite above GEO.
- Costs will be far less with in many ways a far simpler mission if the spacecraft are designed to return samples to above GEO than if designed to return to the Utah Test and Training Range
- Science return will be far greater as the bonus samples can only be added in the next few years before the spacecraft are launched to Mars and ideally long before.
[assuming this book is correct in the conclusion that the bonus samples are needed]
The builders of the spacecraft needed to know all this a year ago! The sooner the better.
I think that the immediate priority is to ensure that there is some understanding by the planners of these spacecraft that they might need to
- modify the designs to remove the aeroshell and add bonus samples.
- remove all the technology that is used to break the chain of contact with Mars
[This only needs to be broken between the miniature lab above GEO and Earth's biosphere] - add fuel for it to return to above GEO under its own power as far as possible.
- add a few more grams of payload to the Mars Ascent Vehicle for the bonus samples
The payload for the Mars Ascent Vehicle has implications for the design that would need to be built in from the start. Similarly the payload to be sent to Mars and returned to Mars for the Earth Return orbiter. Typically the payload mass is one of the first things a rocket designer needs to sort out. Without that they don't have a fuel budget and that makes detailed design impossible.
Samples can be safely sterilized in a satellite similar to geostationary satellites
The two alternatives are to use Cobalt 60 sources or to use X-ray emitters. Cobalt 60 sources can be very heavy, with much of the mass needed for shielding. However if the sterilization is done in an external airlock it will only need to be shielded on the side towards the spacecraft. Also the spacecraft would be designed to withstand the ionizing radiation of the space environment above GEO and could withstand some ionizing radiation from the cobalt 60.
A cobalt 60 source can't be switched off. It can only be blocked out by using blocking materials such as lead.
The simplest solution might be to use nanoscale x-ray emitters. In one experiment the tube operates at 50 kV with an emission beam current of 140 μA, or a power output of 7 watts which would suggest power consumption of 10s of watts, far less than a conventional X-ray. The dose at 3 cm is 8.19 Gy per minute (Kim et al., 2016). However, they were blocked by just 3 mm of a copper collimator and may be blocked by the walls of the tungsten sample tubes. This needs to be looked into carefully.
X-rays might be better as they can be switched on and off and adjusted. A satellite above GEO can potentially have a significant power supply for generating X-rays. The Inmarsat 5 F1 has a power supply of 15 kilowatts on launch (Inmarsat, 2013). That 8.19 kGy a minute or about half a megagray an hour at a distance of 3 cms using nanoscale X-ray emitters.
The dose required to sterilize the sample could be of order 10 megagrays depending on the outcome of a review of this topic:
The modern technology of carbon nanotube emitters leads to greatly reduced power requirements for the X-rays and seems to be enough to sterilize small samples but may need to be used for a long period of time to sterilize the entire sample container.
This needs more work, on the power requirements, X-ray doses available, the level of sterilization required, and the attenuating effects of the tungsten sample tubes on X-rays and on gamma rays from Cobalt 60 sources if they are to be opened on Earth rather than in orbit. If the tubes are opened in orbit then it may be easier to sterilize subsamples.
Velbel et al. describe two other sterilization methods that could be useful for the returned samples. acid hydrolysis and microwave-assisted hot acid extraction. Both of these work by breaking the bonds between amino acids in proteins and they would also break apart prions but don't damage the bonds internal to the amino acids. These would work for terrestrial biology but would it work with a novel independently evolved biology on Mars? This is another matter that would need to be looked at with the higher level review of the topic with experts with the necessary expertise to evaluate potential for sterilizing novel biochemistry:
The main reason for opening on Earth would seem to be to analyse the headspace gases, but these may well be of almost no value for understanding the martian atmosphere because of outgassing from the sample tubes and the samples themselves, and the terrestrial contamination and they are best opened at low atmospheric pressure for the geological specimens which might be done more easily in orbit. See above:
If we collect bonus samples and wish to open any of them on Earth rather than in the orbiting satellite, these can be collected in containers that are designed to be relatively transparent to ionizing radiation so that they are easily sterilized for return to Earth.
We can't rely on ultraviolet light for sterilization.
Ultraviolet light is significantly blocked by a single grain of dust and so may not be adequate for a high level of confidence to sterilize any possible microbes even on the exterior of the Orbiting Capsule
Martian dust could get onto the exterior of the sample container when NASA opens a door in the sample return vehicle to load the samples into the container.
NASA plan to use ultraviolet light to sterilize the exterior of the Orbiting Sample container (Kuthnor, 2023. NASA Will Zap Mars Sample Contamination With Powerful UV Before Returning to Earth). They need to sterilize it because it will be exposed to the martian atmosphere.
However this might not be adequate if NASA wishes for a high level of certainty that there can't be any unsterilized microbes in the dust.
Researchers have found that UV light is significantly blocked by a single grain of dust. So a microbe that happens to be beneath a grain of dust or imbedded in a crack might not be affected. See above:
Then we may need to consider the possibility of martian life that has evolved spores or other propagules that are far more UV hardy than any terrestrial spores after billions of years of evolution in conditions of very high UV. There seems to be scope for adaptations terrestrial life hasn't explored such as spores with extra coatings to block out UV.
“Earth Entry Vehicle” can be converted into an “Above GEO Insertion Vehicle” by replacing aeroshell with extra fuel for its ion thruster and returned to a safe orbit tens of thousands of kilometers above GEO - or perhaps to the Earth Sun L2 point like the James Webb telescope
[FIXCITES]
Whether we return the samples to a satellite just for preliminary sterilization before returning sterilized samples to Earth or whether we return them to a life detection satellite, we need to find an orbit that is safe and isolated from Earth's biosphere to return them to.
: 2We can’t return uncontained unsterilized samples to the Moon at present. COSPAR guidelines for restricted category 5 (sample return) missions currently say that
"(The Moon must be protected from back contamination to retain freedom from planetary protection requirements on Earth-Moon travel)".
(COSPAR, 2011. COSPAR Planetary Protection Policy, 20 October 2002, as amended to 24 March 2011 : 2)
As Cassie Conley put it:
Cassie Conley: “To preserve the ability for humans to travel between the Earth and the Moon, without needing decontamination protocols, it would be nearly as bad if the Moon got contaminated, as if the Earth were. So if there is anything hazardous in Mars samples, a failed facility could not be allowed to contaminate either the Moon or the Earth.”
(Marks, 2022.Calls grow for a safer Mars Sample Return)
So then there’s the question of where to return it safely in the Earth Moon system. Cassie Conley, NASA’s second planetary protection officer suggests one the Lagrange points.
Cassie Conley: “If somehow these cost and technical concerns were solved, the only location that would make sense for an orbiting facility would be in a fail-safe orbit near one of the Lagrange points — where the orbit would decay away from the Earth-Moon system if the facility lost power.”
(Marks, 2022.Calls grow for a safer Mars Sample Return)
There are two such Lagrange points that are natural candidates, the Earth Moon L2 on the far side of the Moon from Earth, a point of balance between the tugs from Earth and Moon that circles Earth once every lunar month. If it loses power it spirals outwards. The other one is the Sun Earth L2 which is further away on the far side of Earth from the sun, again a point of balance between the tugs from the sun and Earth.
However sadly the Earth Moon L2 point is not that safe because there are trajectories that can lead from the Earth Moon L2 under the influence of the sun’s gravity to an Earth flyby as in (Kakoi et al., 2014. Access to Mars from Earth–Moon libration point orbits: manifold and direct options : Figure 13)
So it would seem to require the Sun Earth L2 location. This is where the James Webb telescope operates, graphics from (NASA, n.d. Webb orbit).
Spacecraft enter into halo orbits around the L2 position where they circle around the line joining l2 to Earth like this:
If a spacecraft is in a halo orbit but a little closer to Earth than L2, then without station keeping, over time it will drift closer to Earth and into wider and wider halo orbits which would seem to be not very safe as it remains within the Earth Moon system with very complex orbits that it can get into such as interacting with the Earth Moon L2.
But if it is in a halo orbit further away from Earth than the Sun Earth L2, then if it runs out of fuel it will gradually drift further and further from the Earth in wider and wider circles. So this seems the best fit for Cassie Conley’s suggestion.
However the latency is quite high for teleoperating instruments in the Sun Earth L2 position. Around 10 seconds round trip.
It also takes more rocket fuel and takes a longer time to send a mission to the Earth Sun L2 than to above GEO though there are low energy transfer orbits that take longer but use far less fuel via Earth Moon L2
The orbit above Geostationary Earth Orbit first sight seems very safe, as it is over a kilometer per second delta v away from both the Moon and the Earth. However these orbits are not as stable as they might seem to be. They can gradually change under the influence of the gravity of the Moon and the Sun.
The main issue is that a breach of containment, such as an explosion of a supply mission or a tank on the satellite risks contaminating the satellites in GEO which at some point in the future likely get visited and serviced by human astronauts.
Then there’s the risk that any particles that are released from the satellite – either bits of cladding lost or after an explosion, may eventually collide with other satellites. Large particles might also be a risk for Earth’s biosphere if they are large enough for some of the material to survive re-entry unsterilized by the heat of re-entry though the lighter material and bits of cladding would just burn up in the atmosphere.
However even with all these issues, there are potentially safe orbits above GEO which may be free from the risk of contaminating the satellites in GEO or Earth’s biosphere. These are in the Laplace plane, which is where Earth’s ring particles would orbit if it had a ring system like Saturn. It happens to be inclined to the equatorial plane at 7.2° and any particles released in the ring plane well above GEO would never contact satellites in GEO.
It’s already been proposed as a disposal orbit for satellites in GEO at the end of their lifetime so that even if they collide with each other and break up there is no risk to the satellites in GEO.
The main complication here is that satellites often shed large light fragments of cladding called High Area to Mass Ratio particles. These can get their orbits changed rapidly under the pressure of the sun.
Satellites often shed large light fragments of cladding called High Area to Mass Ratio particles. These can get their orbits changed rapidly under the pressure of the sun.
However Rosenberg et al. found that even these particles can stay trapped well away from the protected distance and inclination space of GEO, through the balance of the light pressure from the sun and gravity. Based on their analysis in 2013 they concluded:
Based on our analysis of the modified Laplace plane, we showed that if satellites located in the classical Laplace plane graveyard orbit shed HAMR objects, their orbits will be trapped in (i, Ω) phase space, and will not likely cross through geostationary orbit. Thus, the Laplace plane graveyard orbit is analogous to the ocean gyres which are characterized by exceptionally high concentrations of marine debris that have been trapped by the currents. Future work will investigate the robustness of these solutions to more realistic SRP models and the economic viability of this proposed GEO graveyard.
(Rosengren et al., 2013. Long-term dynamics and stability of GEO orbits: the primacy of the Laplace plane : 15)
The evolution of eccentricity is also acceptable with no risk of the objects hitting Earth’s atmosphere (Rosengren et al., 2013. Long-term dynamics and stability of GEO orbits: the primacy of the Laplace plane : figure 4).
This would need to be looked at closely, but there may be orbits above GEO at suitable inclinations that would be very safe.
Since the satellite would be equipped with end of life-time sterilization with an onboard heater the main concern is for particles released before the satellite is decommissioned.
Another solution might be to design the satellite to be particularly robust so that the risk of cast-off fragments is next to zero. This is easier if we can also deliver the samples to the satellite in such a way that there is no risk of contamination of the cladding of the satellite with the martian material, and ensure that Mars samples would be contained for any possible internal explosion of fuel tanks or anything else that could explode, and if we can ensure that there is no real risk of a collision breaking up the satellite before end of life-time sterilization – and fail-safe multiply redundant ways to sterilize the sample at the end of the lifetime of the facility or after some irrecoverable accident.
Since the satellite would be equipped with end of life-time sterilization with an onboard heater the main concern is for particles released before the satellite is decommissioned.
Another solution might be to design the satellite to be particularly robust so that the risk of cast-off fragments is next to zero. This is easier if we can also deliver the samples to the satellite in such a way that there is no risk of contamination of the cladding of the satellite with the martian material, and ensure that Mars samples would be contained for any possible internal explosion of fuel tanks or anything else that could explode, and if we can ensure that there is no real risk of a collision breaking up the satellite before end of life-time sterilization – and fail-safe multiply redundant ways to sterilize the sample at the end of the lifetime of the facility or after some irrecoverable accident.
There are other “frozen orbits” and orbits that a satellite will remain in on timescales of centuries giving plenty of time for safe decommissioning, such as sun synchronous orbits which some Earth observation satellites orbit in where the influence of the Earth’s oblateness keeps the orbit continually progressing synchronously with the sun so it always approaches Earth on the sunny side with each orbit. These are similar to capture orbits for a sample returned to Earth so may be especially useful but if they come close to Earth we need to make sure they are far from any other terrestrial satellites.
Then there’s the lunar Distant Retrograde Orbit (DRO). That is an orbit around Earth in the same direction as the Moon (prograde) but the satellite overtakes the Moon on the inside when close to Earth then falls back behind it on the outside when furthest from Earth so that as seen from the Moon it is a retrograde orbit.
The DRO is an easy one to get into from Mars and a satellite placed there is stable for at least 100 years (Lock et al., 2014, March. Mars Sample Return Orbiter Concepts Using Solar Electric Propulsion for the Post-Mars 2020 Decade : 8). Latency for a satellite in a DRO is similar to the Moon.
To get the satellite into the lunar DRO, Lock et al use an Earth close approach at an altitude of 28,500 km. However this does not seem to be a significant risk to Earth, especially for a low continuous thrust spacecraft such as ESA’s Earth Return Orbiter, so long as it is trajectory biased away from Earth at all times during the approach. Similarly the lunar flyby seems to be no significant risk for the Moon with a low continuous thrust spacecraft.
But above GEO seems particularly suitable if it can be shown to work because it is especially easy to get to from Earth, and low latency compared to the other orbits.
From Lock et al.’s lunar DRO, it is easy to transfer to Lunar L2 (LL2) (Ming et al., 2009. Exploration of distant retrograde orbits around Moon), the gravitational point of balance beyond the far side of the Moon as seen from Earth. From there it can do a low energy transfer to a Lunar L1 halo between Earth and the near side, and then it can get to above GEO, using lunar flybys in fuel efficient ballistic transfer trajectories again, to reduce the total delta v requirements.
There is another especially promising low energy trajectory that may be worth mentioning as it avoids even flybys of Earth or the Moon. This is the reverse of the trajectory in (Kakoi et al., 2014. Access to Mars from Earth–Moon libration point orbits: manifold and direct options : Figure 13) which can be used in reverse. . It would need to be re-analysed for the reverse direction but the principle is the same. It threads through the Sun Earth L2 to the Earth Moon L2, and from there it can transfer to LL1 and then above GEO as before.
Earth’s “ring plane” is inclined at 7 degrees to the equatorial plane. Saturn’s rings superimposed to scale (Rosengren, 2013. Long-term dynamics and stability of GEO orbits: the primacy of the Laplace plane)
Cassini photograph of Saturn from above stretched and mirrored to approximate a circle (APOD, 2013, Saturn from above ), and Earth from above the North Pole (NASA, 2019. Arctic and Antarctic Sea Ice: How Are They Different? )
The proposed “graveyard orbit” in the Laplace plane is not far above GEO. The suggestion is to put the new satellite well above GEO, perhaps 50,000 or 100,000 kilometers higher. It’s highly unlikely we ever need to place satellites that humans need to visit at that location or in the Laplace plane. This makes it a perfect location for our sample receiving satellite.
This needs to be looked at carefully.
The satellite would need to
- be sterilized at end of lifetime
- orbit far from any other satellites
- be in or close to frozen orbits or in some other way ensure that any debris that could realistically be shed from the satellite before end of mission sterilization would be kept away from Earth for long enough to be thoroughly sterilized by ionizing radiation
- refueled by supply vessels refueling it at the same time as they bring supplies or new experiments and remove sterilized materials for analysis on Earth.
- Only risk of collision would be with supply satellites
- Plus risk of shed cladding which perhaps could be reduced by other means.
- low risk of explosion
Frozen orbits above GEO seem especially promising, but there are many alternatives to look at here. This needs to be looked at carefully by experts in orbital mechanics and frozen orbits. Can an orbit be found that will be safe for Earth’s biosphere and not risk contaminating astronauts in space?
A miniature lab above GEO can cost less than a Mars receiving facility on Earth - five times the payload for a mission to Mars for the same launch cost with far less complexity
[FIXCITES]
To compare the costs of the proposed miniature life detection lab above GEO with a ground based Sample Receiving Facility, we need to look at the launch costs to above GEO and the costs of the satellite itself.
For the Sample Receiving Facility this section just assumes a cost of half a billion dollars as for the fully telerobotic design considered in the 2010 decadal review, $471 million in 2015 dollars (Mattingly, 2010, Mission Concept Study, Planetary Science Decadal Survey, MSR Orbiter Mission (Including Mars Returned Sample Handling)) or $659 million dollars today. That is more than five times the cost of a standard BSL-4. See above:
A terrestrial lab that can contain even ultramicrobacteria and with full end of lifetime sterilization might cost considerably more. Then if the ground based lab is required to achieve the same high standard of biosafety of no appreciable risk as the telerobotic life detection lab above GEO, the cost will be considerably more. See Supplementary information under:
We will find launch costs up to 5 tons are likely to be of order tens of millions and even for a 20 ton satellite are likely to be less than $100 million dollars with these costs likely to be considerably reduced by the 2030s.
The complete for NASA to build and deploy the satellite is likely to be similar to a typical GEO satellite of $150 million to $500 million with the costs less than $100 million for a satellite of less than one ton (Hentry, 2020. Geostationary satellite orders bouncing back) with these numbers also likely to be reduced by the 2030s.
Most of the cost for life detection instruments and the Mars simulation chamber would be likely to met by universities and institutions who would be interested to take part in the mission. The main extra costs for NASA over a standard satellite above GEO would seem to be a docking port for supply missions, and cost for a sterilization unit to sterilize samples returned to Earth.
We also need the costs of missions to the satellite to deliver supplies and return sterilized samples but these would be greatly reduced in cost by the 2030s.
In more detail:
For the launch costs to above GEO, the Falcon 9 can deliver 8.3 tons to Geostationary Transfer Orbit (GTO) at a cost of $67 million and the Falcon Heavy can already deliver 26.7 tons to GTO at a cost of $97 million for the reusable rocket (SpaceX, n.d., Capabilities and Services).
We have to allow for transfer from GTO to above GEO and for station keeping. Although we likely wouldn't send anything this large we could send multi-ton satellites to above GEO. With a rough calculation, I make it about 5 tons dry mass allowing for fuel and station keeping if we use the Atlas V, to send to above GEO, compared to 1.025 tons for Perseverance. It's over 20 tons if we use the Falcon Heavy, in both cases using electric propulsion. The launch costs even for the Falcon Heavy at over 20 tons to above GEO would be less than a fifth of a fully telerobotic terrestrial facility.
The Falcon Heavy's 26.7 tons gets reduced because a satellite needs to use some of its mass to circularize to GEO, and by a little more because the destination is above GEO. But we can expect to deliver over half of the GTO mass to our final orbit above GEO, or over 14 tons. If the spacecraft uses electropropulsion to transfer from GTO to GEO we may be able to deliver over 20 tons to GEO using the Falcon Heavy. Then we need to allow for station keeping.
There is very little by way of extra delta v to transfer to a higher orbit at say 100,000 km above GEO. I did a little online calculator to help, as a first rough idea.
Missions start in LEO at altitude 250 km and velocity 7.755 km / sec.
Transfer to GEO at altitude 35786.154 km and velocity 3.075 km / sec:
Delta V = 3.912 km / sec = 2.44 km / sec (leave LEO) + 1.472 km / sec (insertion to GEO).
Transfer from LEO to orbit at altitude 100000 km and velocity 1.936 km / sec:
Delta V = 4.158km / sec = 2.886 km / sec (leave LEO) + 1.273 km / sec (insertion to orbit at 100000 km).
Transfer from LEO to the orbit at altitude 100000 km needs:
Extra delta V = 0.246 km / sec
- compared to the transfer to GEO.
Based on a 15 year mission, with chemical motors and station keeping, the dry mass is 27% of the initial mass including fuel which would be 7.2 tons. If we can use electric propulsion then the dry mass is 61% of the initial mass or 16.28 tons. These are illustrative figures based on a worked example of transfer from GTO to GEO in:(Thomas, 2016, A Comparison of GEO Satellites Using Chemical and Electric Propulsion : Table 2). If we use the Falcon 9 and the 61% figure, the 8.3 tones is reduced to a little over 5 tons to GEO.
It's reduced a little to target above GE and we also need to allow for returning the sterilized samples to Earth which eats into that 5 tons / 22 tons payload to GEO with electric propulsion. However, by comparison Perseverance has a dry mass of 1.025 tons and was launched on an ATLAS 541 (NASA, 2020, Perseverance launch) which can send a payload of 8.29 tons to above GEO (ULA, n.d., ATLAS V), almost identical to the Falcon 9 and almost identical 5 tons to GEO
So, based on that example anyway, a satellite in our safe orbit well above GEO can be around five times heavier than anything we can send in situ to Mars.
So, though we likely don't need anything as large as this, we could send something as large as five tons to above GEO if it's needed, at launch costs of a fifth or less of the at least half a billion dollars for a fully telerobotic sample receiving laboratory.
As we approach the 2030s, launch costs are sure to go down further. SpaceX might be flying the super-heavy by the 2030s and there may be competition from others to reduce costs. We may be able to send multiple missions to the satellite at very low costs compared to today.
As for the cost of the satellite itself, a lot of the cost would be for the instruments, and the Mars surface simulation centrifuge. Those may be supplied by universities at essentially zero cost to NASA.
The main cost to NASA in that case may be similar to the cost of a satellite to send to above GEO, needing to provide power for it, solar panels, etc. Those costs can be very low compared to the half billion dollars of the 2009 estimate of a Mars Receiving Facility, depending on the size. Assuming the satellite weighs no more than a ton then it may cost $150 million or even less for the total cost including launch costs, insurance, manufacturing and gateway stations (for ground based communication with the satellite).
This for instance is a summary from early 2020
Villain said the average capital expenditure for deploying a GEO satellite, including manufacturing, launch, insurance and gateway stations, ranges from $150 million to $500 million. She estimated satellites under 1,000 kilograms likely cost operators less than $100 million to deploy. Villain said that the “performance/capacity of GEO comsats is growing proportionally faster than their prices,” meaning operators are getting more capacity per dollar spent than they did in the past.
(Hentry, 2020. Geostationary satellite orders bouncing back)
These costs would go down a lot.
NASA would also need to provide a way to to dock with it robotically to transfer the samples to it. Then once samples are returned to the satellite, scientists would want to send new experiments to the station, and to return materials for analysis on Earth. It would also need to incorporate some way to sterilize the samples, X-rays or a cobalt source perhaps.
However most of these costs would be for the 2030s when cost to above GEO are likely to be significantly lower than today. This needs to be looked at more closely to see how it works from an engineering point of view. However it seems interesting enough for further study and the costs seem to be within the ballpark of a terrestrial Sample Receiving Facility and possibly a lot less.
The main alternative is to study the samples in situ on Mars or telerobotically on Earth (if one agrees on the need to prevent lab leaks). Pending a detailed review, this seems like it may be the lowest cost of those three alternatives and to have a higher technological readiness level.
There are other advantages to returning the samples to above GEO. This is an ideal location for an international partnership between multiple space agencies as for the ISS but far lower cost. JAXA (Japan), ESA, ISRO (India), ROSCOSMOS (Russia, if relations with Russia are better by then) and CNSA (China, if the US is on reasonable terms with China by then) would all be able to contribute modules or equipment just as they do on a larger scale for the ISS,
This collaboration again would be likely to find most of the funding for the Mars simulation chamber, which would be an exciting project for them because of the opportunity to reproduce the Martian surface environment far more faithfully than is ever going to be possible in a terrestrial laboratory.
As for the life detection instruments, there would be a lot of interest and competition between teams at universities to design and build instruments to send to the facility. It would be of special interest as a first step towards space certification to eventually send their instruments further afield to Mars, Ceres, Europa, or Enceladus.
This proposal also seems forward looking, a proposal not just for a Mars sample return, but one that would add capabilities above GEO that would be of enduring value for many other future missions.
How a miniature life detection lab above GEO can avoid the technological uncertainties of a fully telerobotic biosafety lab on Earth, at significantly lower cost, with a significantly higher technological readiness level
[FIXCITES]
There seem to be two main ways forward to handle returned unsterilized samples returned to Earth's vicinity, bearing in mind the need to contain lab leaks and the impossibility of using quarantine to contain unknown biology in a lab staffed by human technicians.
- fully telerobotic terrestrial biosafety lab rated to contain even extremely small and exceptionally hardy alien life,
- miniature lab in space such as above GEO
There may be other solutions available if NASA opens this to wider debate. But of those two ideas, the miniature life detection lab above GEO seems likely to win out once the engineers do a careful comparison, taking account of the need to do a new Mars sample return study first, review of level of assurance and size limit and to develop the novel technology for the fully telerobotic terrestrial biosafety lab.
We looked at costs in the last section, see:
The main focus here is the technological readiness level. This is not the place to do an engineering TRL assessment, so this is for illustrative purposes only, looking at some of the considerations.
Caption: Fully telerobotic laboratory complying with the European Science Foundation recommendation for 100% containment of ultramicrobacteria
requires
- update of Mars sample return study from 2009
- review of level of assurance and size limit to contain
- expertise in public health, biosafety, etc., not historically associated with NASA
- cost well over $500 million
Low TRL
Miniature lab above GEO, based on 2016 proposal by NASA / ESA for life detection on Jupiter's moon Europa.
requires
- review of sterilization levels suitable for an unknown lifeform of possibly alien biology
- costs less, more science return
- requires expertise NASA already has.
High TRL.
For illustrative purposes only, assessment of technological readiness level of the two alternatives considered would likely be low for the most important enabling technologies for a fully telerobotic laboratory and high for a satellite.Graphic from NASA, 2012, Technological Readiness Level)
I expect the reader to agree on the TRL for the miniature lab. The basic enabling technology is a satellite above GEO and this is TRL 9. There are many such in use. The other main technologies, with an estimate of the likely TRL, are:
- TRL 7: Centrifuge that operates in microgravity with slip-ring technology to supply power, liquid and atmosphere while the centrifuge is operating, already in use on a smaller scale in the ISS and only needs to be scaled up, TRL 7 if we treat the ISS centrifuge as a systems prototype in a space environment as there is likely to be little needed to scale it up in size.
- TRL 4 -9: Miniature instruments with many available that vary from TRL 1 to TRL 6 with the LDChip already accepted as part of the ExoMars payload. The Viking labeled release is TRL 9 as it flew on Viking. There are enough instruments at TRL 4 to 6 to satisfy the principal iMost goals.
- TRL 6:Sterilization is mature technology, and likely to be assessed at TRL 6, though not yet flown in space. We need a review of the level of sterilization needed but there doesn't seem to be any practical obstacle to putting a sterilization unit in space to achieve whatever level of assurance is needed, especially at above GEO where we can send tons of equipment in a payload.
- TRL 1 but conceptually easy to do:The idea of a 100% sterile contamination free container and the scoop to collect bonus samples is TRL 1 but can be expected to move up the TRL levels quickly once it becomes a priority as everyone agrees we know how to achieve 100% sterility, the issue is the extra mission critical failure point that we may not be able to open the bag containing the 100% sterile components on Mars, but this is a risk only for the bonus sample collection itself.
At first sight it might seem that the TRL should be higher than shown in this diagram for a fully telerobotic laboratory. After all telerobotics itself is mature technology.
The issues here that make the TRL low are the need to combine telerobotics with a far higher level of biosafety than ever attempted before, and also to combine it with the needs to keep the samples clean of any terrestrial contamination. Some of the technology requirements for the fully telerobotic lab are not yet even at TRL 1.
So some of the issues are
Before we start:
- We need a new sample return review before we know what we need to contain, for instance do we need to contain the very small ribocells or even prions?
- We need an update of the size limit (could be part of the same review)
Then there's the update of the level of reassurance, and depending on the outcome if this sets a high level of assurance, even a fully telerobotic facility wouldn't satisfy it because of issues such as accidents, theft, a plane crashing into the facility, etc. (though it could be upgraded to a higher level of assurance at higher cost).
- We need an update of the level of assurance and this needs broad participation by the public, not just decided by scientists as it is an ethical decision as much as it is a scientific decision, synthetic biologists have suggested that to contain mirror life we need far higher than a 1 in a million level of containment
- This would include discussion of the prohibitory version of the precautionary principle. If we require "no appreciable risk" the ESF study in 2012 concluded that this would simply lead to cancellation of the mission and this seems likely to be the situation today with present day technology
Technology doesn't exist yet for many of the requirements which are at TRL 1. Some would more properly be TRL 0 as they don't reach the minimum requirements of TRL 1 as research hasn't yet started though TRL 0 doesn't exist in the official scale which is normally used for situations where the proposers at least have some ideas for ways to implement it.
- TRL 0-1: We saw that the technology doesn't exist yet for air filters for the ESF recommendation and nobody is working on it yet
- TRL 0-1: Air incineration may work but we need first to look at potential resilience of alien life to air incineration.
- TRL 0-1: We need a way to remove sterilized samples to distribute to laboratories and to add new equipment, service the telerobots and so on, and all this needs to be done without releasing a single ultramicrobacteria if the lab contains mirror life, this also is at TRL 1 or lower as there doesn't seem to be anyone working on this yet.
- TRL 0-1: We may need end of life sterilization of the facility if it could end up contaminated with mirror life or something else that could remain dormant for long periods of time and must never contact our biosphere, and this is TRL 0-1 as there seems to be no work yet on biosafety labs with end of lifetime sterilization of the entire facility.
- TRL 1: The need to protect the Mars samples from terrestrial contamination means we also need to ensure the telerobots are sterile - we have a concept diagram for how to build such a facility but it couldn't be described as higher than TRL 1 at present.
There may be solutions to all this. For instance the miniature technology for the life detection lab above GEO could shrink the size of the ground based facility and make many of the issues far easier to solve than previously imagined. But currently this is at TRL 1 (or 0?).
Then we have issues involved in integrating of all this into a new facility, all would be TRL 0-1 too.
- We have no experience of operating a fully telerobotic laboratory
- once it is built we are likely to encounter issues with the integration of all the technologies used to construct it
- also with training of the technicians to operate the telerobots.
It will be hard to assess how easy or difficult this alternative is until we have the next Mars sample return study, the level of assurance update, and have basic concepts sketched out to bring this alternative to at least TRL1 throughout so that we can see what the overall plan is to achieve the recommended size limit and level of assurance.
A level of assurance update could even lead to a conclusion that we need to achieve no appreciable risk to Earth's biosphere and that we should also protect any intrinsic martian novel species, or second genesis or biodiversity in the forwards direction at the same level of no appreciable risk, at least until we know what we might lose.
In the worst case in the forwards direction, it is possible we make some novel form of life extinct on Mars that has only just managed to survive to the present for over 3 billion years since Mars was more habitable. In that situation even if the last martian microbe went extinct a year before we find it, we wouldn't be able to de-extinct it. So that is a positive of the telerobotic facility decision.
This topic takes up a fair amount of space if we go into the details so it's in a Supplementary information section. I cover that here:
- ⸽ The life detection lab above GEO achieves no realistic risk to Earth's biosphere, so is resilient to any future change in recommended levels of assurance
- The ESF in 2012 ruled out the prohibitory precautionary principle, because it was asked to find a way to return unsterilized samples, but a study with a broader mandate might require no appreciable risk for unprecedented harm
As I say there, whatever ones views on whether those are necessary requirements for planetary protection, they are clearly benefits that are good to achieve if we can. The miniature lab above GEO and 100% sterilization in the forward direction achieves those benefits in addition to its other advantages.
It also means that if we do make a decision to drop planetary protection, we do it at a later stage based on knowing what the impact would be if any, on a native martian biosphere, or on Earth's biosphere.
In this way we keep our future options open if we achieve no realistic risk both ways. If we reduce planetary protection letting any biospheres merge before we know what we'll find on Mars or what the effects will be of terrestrial life on Mars or martian life on Earth, we close down options that we would otherwise have had in the future that we might have preferred, and might perhaps have been of significant value to our civilization, or avoided harm, compared to what happens if we just let both biospheres merge without knowing in advance what might happen. It would seem wise to keep our options open until we can fill the knowledge gaps.
Conclusions: too many unknowns for NASA's plan
There are far too many unknowns at this stage for us to rely on NASA's plan as a way to protect Earth's biosphere and inhabitants. We need to fill in the knowledge gaps first.
The main take-home messages from this book are that:
- We have many knowledge gaps and need a respect for the unknown
- We could find something as astonishing on Mars for astrobiology as the martian geysers were for geology
- We need to consider worst case scenarios as well as best case scenarios
- Quarantine can't protect Earth from lab leaks of extraterrestrial life of unknown capabilities such as mirror life, alien pathogen, or a distantly related novel fungal genus
For unsterilized samples returned to Earth's biosphere:
- We need a new Mars sample return study, as the last one was 14 years ago and much has changed since then
- We need an update on the size limit to contain and level of assurance
- A fully telerobotic laboratory is likely sufficient but would cost at least half a billion dollars
- NASA doesn't have the expertise on its current team to design a biosafety plan for Earth or to run a first of its kind fully telerobotic laboratory
- NASA would need to assemble a team with the required disciplines, restore its interagency panel and learn to liaise with and listen to experts on human health and biosafety.
One solution that plays to NASA's strengths doesn't require NASA to make such substantial changes to its organization and its team:
- A miniature telerobotic lab above GEO based on "lab on a chip" microfluidics and other novel exquisitely sensitive instruments similarly to Europa / ESA's joint life detection mission plan from 2016
- Bonus samples would add greatly to the astrobiological interest
- This would have many co-benefits including the capability to provide exact simulations of Mars, and other destinations like Europa, complete with artificial gravity, seasons, day night cycles and so on
For NASA's plan to send human astronauts to Mars:
- NASA needs to prove it is biologically safe for astronauts land on the planet
- However on our present level of understanding, there are scenarios where it is biologically unsafe to land humans on Mars.
From a financial perspective, in view of the importance of protecting Earth's biosphere, its inhabitants and the potential superpositive value of Mars
- NASA and private space have a perverse incentive to reduce planetary protection to save on costs
- Congress could remedy this with a new organization which can supplement private missions and NASA missions with up to 10% (or more) of funding to be used only for planetary protection
- Staffed with independent planetary protection experts who would be tasked with deciding if planetary protection is needed and what needs to be done
- This body could also valuably be used to stimulate the technology needed for a rapid biological survey of Mars and safety assessment
- It could provide additional funding to develop landers that use commercial components (mostly) capable of withstanding a short period of heat to 300°C
- And additional funding for broadband infrastructure
- Both seen as public goods similar to public highways
In the broader context of enthusiasm for humans in space and space settlement
- We need a plan b for those who want to establish a terrestrial "planet b"
- A future where humans can never land on Mars is also an inspiring one to prepare for
- A future with humans studying a mirror "planet b" from orbit can achieve all the same goals that colonization enthusiasts have for their "planet b"
- With habitat bubbles in space instead of on Mars
- Habitat bubbles would be needed anyway for the first few centuries for any attempt to terraform Mars.
If we have a terrestrial mirror life planet or other unsafe biology, we can learn to value our terrestrial planet b as it is without changes
- A novel planet b of mirror life, or early prebiotic chemistry that hasn't quite made its first steps to life and settlers in numerous other locations in our solar system including the Martian moons and in orbit around Mars could be more valuable to our civilization than a less habitable clone of Earth
- The Moon is ideal for a backup of seeds, a library, and achievements of our civilization with conditions in the lunar poles and lava tube caves better suited for those purposes than anywhere on Mars and closer to Earth if we need to use the backup to replace damaged terrestrial seed banks etc.
- There are numerous other locations in our solar system that we can expand to after our first experiments in space settlement on the Moon once we find it easier to live in space with minimal resupply from Earth
We found many geological surprises on Mars. When we start to search for life in situ on the planet, we may find equally astonishing biological surprises. We also don't know enough about Mars to drop measures to protect whatever might be on Mars in the forward direction.
Some of the worst cases for planetary protection for a Mars sample return such as mirror life would be amongst the most interesting best cases for science interest, These combination worst cases / best cases would mean we can't and humans on the martian surface, but would stimulate space exploration and the need for humans in space, but in orbit around Mars rather than on the surface, operating telerobotic avatars on the surface to explore a planet with fascinating but unsafe alien or distantly related life.
Considered positively, we might have something of tremendous value on Mars, a superpositive, a heirloom for our civilization. We could confuse the science by mixing it with terrestrial life, or if it is vulnerable such as early life, destroy it, before we know it is there. We could lose something precious, perhaps with the nearest example we could reach beyond Mars at thousands of light years away. Mars gives us a unique opportunity in our solar system to explore a terrestrial planet like Earth but with a different history for the past 4.5 billion years. We have Venus, Earth and Mars. Any other terrestrial planet in a potentially habitable zone will not be closer than the closest star, Proxima Centauri, over 4 light years away).
If we need to find a terrestrial planet orbiting a star from the same birth nebula, we might need to look over 100 light years away. These could be of interest because the planets were formed from a nebula with the same chemical composition. They could also be of special interest in the search for extraterrestrial life because they could have shared the ancestors of modern life in the birth nebula. The closest such planet may be Mars. Apart from Venus if there is any life remaining in the atmosphere, after that we start with planets orbiting HD 162826 110 light years away in the constellation Hercules.
In their earliest days within their birth cluster, he explains, collisions could have knocked chunks off of planets, and these fragments could have traveled between solar systems, and perhaps even may have been responsible for bringing primitive life to Earth. “So it could be argued that solar siblings are key candidates in the search for extraterrestrial life,” Ramirez said.
(Texas University, 2014, Astronomers Find Sun's "Long-Lost Brother," Pave Way for Family Reunion)
There are many complexities involved in starting an anthropocene on Mars. Given how hard it is to predict even how the terrestrial permafrost will respond to melting it seems wise to do small scale experiments in Mars habitats before irreversibly introducing terrestrial life to Mars in what tight be a random seeding of terrestrial biology for the first time on a new planet.
If Mars is indeed habitable to terrestrial life, we need to bear in mind that a decision to introduce terrestrial life to the planet is a decision not just for our civilization now but for our civilization in the future and all future civilizations on Earth for all future time. If there is independently evolved or distantly related life on Mars and we return novel life in samples from Mars to Earth this similarly may result in an irreversible change to Earth's biosphere not just for our civilization now but for the future of our civilization and future civilizations too.
This mission has an ethical dimension which requires wider participation in decision making than is usual for a space mission. It is important to ensure that space agencies do follow the NEPA guidelines in the USA. We have found many instances where it would seem that NASA's PEIS needs closer examination to find out if it did indeed follow those guidelines.
This mission is especially important as a precedent for other space agencies and private space. This book has outlined a way forward that would make it far easier for private space and for space agencies to comply with the desire of many in the public to maintain a very high level of biosafety protection for Earth. It is also possible to achieve a similarly high level of protection for the martian biosphere. Such an approach is simpler, less costly and it also is resilient to any future changes in the levels of assurance and size limits It is resilient even to a future decision to adopt the prohibitory version of the precautionary principle, that we should permit no appreciable risk when the worst case is unprecedented harm. We can also move forward in a way that achieves no appreciable risk for forward contamination of Mars.
See Supplementary information under:
These approaches far from restricting space colonization are enabling. They permit a far wider range of options for the future. If we return life from Mars to Earth or start an anthropocene on Mars prematurely before we understand the planet, this closes down many future options we will have if we were more careful. We can make those decisions at the appropriate time once we have knowledge to base the decisions on rather than only knowledge gaps and a wide variety of conflicting expert opinions about how these gaps might be filled in the future.
We conclude that the complex legal process is both understandable and necessary. It has served us well in the past and needs to be adhered to carefully to ensure a mission that is beneficial for both NASA and the world.
END OF PAPER
=======================================================
SUPPLEMENTARY INFORMATION
=======================================================
This adds extra scenarios for alien life, extra material on the Swansong Gaia hypothesis, also a list of some of the organisms that do well in Mars simulation chambers and sections on bioethics, the precautionary principle for backwards contamination and the idea of a superpositive outcome, that we might have something of great intrinsic value on Mars that we'd want to protect.
It also has a section on requirements of NEPA that NASA's PEIS fails. These aren't subtle gray area issues for lawyers, but clear violations of many of the most central requirements of NEPA.
Supplementary information contents
- The scarcity of literature on alien biology and human health
- Sagan had to flip the War of the Worlds plot twist to warn about the low risk of harm from Mars microbes, and it remains a science fiction blind spot
- How terrestrial organisms detect "non self" and relevance for alien life: our natural broad spectrum antibiotics only work for bacteria with cell walls with acid coatings, not fungi
- Even microbial life might have no effective defences against alien fungi
- Our immune system is likely to ignore alien fungi with unfamiliar patterns, and our immune system's natural antifungals may not work with alien life
- If our immune system notices alien life it might trigger an allergic response
- Obstacles to developing tests for a novel fungal disease based on an alien biology and developing antifungals for it
- Nanoplastics and microplastics as an analogue of dormant alien life that accidentally triggers blood clots
- Alien amino acids could be misincorporated into proteins like BMAA leading to protein misfolding and accidental neurodegenerative disease
- An alien biochemistry could have many beneficial bioactive compounds but also many accidental toxins
-
Could Martian microbes be harmless to terrestrial organisms?
-
Enhanced Gaia - could Martian life be beneficial to Earth’s biosphere?
- Interactions of nitrogen cycle with Swansong Gaia - if life returns more nitrogen to the atmosphere when Mars is wetter, the Swansong Gaia cycle is reinforced
- Nitrogen fixation scenario 1: Martian life never developed nitrogen fixation – weaker Swansong Gaia effect
- Nitrogen fixation scenario 2: Martian life has nitrogen fixation and also denitrification to return nitrogen to the atmosphere, similarly to life on Earth – strong Swansong Gaia effect
- Nitrogen fixation scenario 3: Martian life has nitrogen fixation but no denitrification –Swansong Gaia effect varies in effect depending on deliveries of nitrogen by comets
- Nitrogen fixation scenario 4: Martian life behaves like the life in terrestrial hyperarid deserts – nitrogen fixation and denitrification but denitrification stops in the driest conditions - strongest Swansong Gaia effect
- Warming from methanogens limited by Swansong Gaia feedback from photosynthesis which produces oxygen which turns much of the methane to CO2 and also fixes the CO2
- Self limiting microbe colonies can convert underground aquifers to calcite to maintain a subsurface barely habitable Swansong Gaia hydrology
- Potential limits on biomass of a Swansong Gaia on Mars based on free CO and H2 in the atmosphere do not rule out Swansong Gaia
- Could there be alternating episodes of runaway warming and runaway atmospheric collapse in early Mars - and could we trigger a runaway warming today?
- Potential for a stronger biological pump during runaway warming if the early Mars atmosphere frequently collapsed and then recovered in a runaway warming again.
-
Estimated 100 millibars of dry ice absorbed in the regolith - is this involved in any feedback?
-
Feasibility of terraforming Mars or ecopoesis (making Mars more habitable for native martian life)
- Ethical decisions about acceptable levels of risk can't be decided using the methods of engineering or science
- Sketch for a future ground facility that is future proof to achieve no appreciable risk even for mirror life
- Why protect even microbes, mosses and lichens from another planet? Potential billions of dollars commercial value, intrinsic value like a work of art or rare species, potential to add new dimensions to the life sciences, and potential benefits of bioactive substances for health
- In all scenarios self sustaining settlement in space likely happens after we have easy colonization of the shallow sea floor, and floating sea cities and farms
- Even if we can never land humans on Mars, we can colonize our solar system with self sustaining habitats slowly spinning for artificial gravity to Pluto and beyond
- ⸽ Mars is only habitable with space suits and colonists living in pressurized habitats, never needed on Earth
- Earth as a jewel of a planet and we are evolved to live here
- If humans can't land on Mars we still have an excellent backup capability on the Moon, indeed the Moon is better than Mars, with Burroughs' proposal of a backup seed bank and library on the Moon
⸽ How NASA's current PEIS fails many central NEPA requirements
- Fails NEPA requirement for scientific integrity: for instance, should have said that the main cite for its Mars meteorite argument rebuts it
- Fails NEPA requirement to identify opposing views to its main arguments such as the Mars meteorite argument
- Fails NEPA requirement to discuss major points of view by not mentioning the view of a low risk of large-scale harm
- Fails NEPA requirement to include reasonably foreseeable environmental effects in discussion of the affected environment
- Fails NEPA requirement to select preparers with disciplines appropriate to the Impact Statement's scope and issues
- Fails NEPA requirements to consider reasonable alternatives that don't fully meet the agencies listed goals but better meet NASA and NEPA policies and requirements
- NASA required all alternatives to be able to determine if it is safe to return unsterilized samples, even if all the samples they return are sterilized
- NASA also required all alternatives to test unsterilized samples for present day and past life in a terrestrial laboratory based on out of date information about in situ capabilities
- Recommendation of Mars sample return studies to involve public in risk governance as soon as possible, and NEPA requirement for interdisciplinary approach integrating social with natural sciences .
⸽ Exploring Joshua Lederberg's suggestion of a risk to human health from alien biology through terrestrial analogues such as fungi, microplastics, and BMAA
When returning samples from Mars, It seems important to consider whether alien pathogens of an alien biology can harm us. Also if alien life can harm us, what might its mode of action be?
As far as I know the planetary protection literature to date has only touched on this topic briefly in two papers by Joshua Lederberg from 1999, in a section of a few paragraphs in each paper. Joshua Lederberg got his Nobel prize for discovering bacterial sex in 1946 (Johnston, 2016, Joshua Lederberg on Bacterial Recombination) (Nobel Prize, n.d., Joshua Lederberg The Nobel Prize in Physiology or Medicine 1958 ), and was a key figure in early work on planetary protection, first developing an interest in it in the late 1950s (Scharf, 2016, How the Cold War Created Astrobiology, Life, death, and Sputnik).
Joshua Lederberg put it like this
Text on graphic: Joshua Lederberg, winner of a Nobel prize in 1958 for his discovery of bacterial sex.
Whether a microorganism from Mars exists and could attack us is more conjectural. If so, it might be a zoonosis [infectious disease that jumps to humans] to beat all others.
Quote from: : Parasites face a perpetual dilemma (Lederberg, 1999, Parasites face a perpetual dilemma : 79 - Contemplating interplanetary zoonoses).
Photograph from: (Joshua Lederberg, n.d., Joshua Lederberg)
This is the full quote
Whether a microorganism from Mars exists and could attack us is more conjectural. If so, it might be a zoonosis [infectious disease that jumps to humans] to beat all others.
On the one hand, how could microbes from Mars be pathogenic for hosts on Earth when so many subtle adaptations are needed for any new organisms to come into a host and cause disease? Dozens if not hundreds of bacterial genes need to work in concert to enable a microorganism to be a pathogen. On the other hand, microorganisms make little besides proteins and carbohydrates, and the human or other mammalian immune systems typically respond to peptides or carbohydrates produced by invading pathogens.
Thus, although the hypothetical parasite from Mars is not adapted to live in a host from Earth, Very little in biology makes sense absent evolutionary insight our immune systems are not equipped to cope with totally alien parasites: a conceptual impasse.
Quote from: (Lederberg, 1999, Parasites face a perpetual dilemma : 79 - Contemplating interplanetary zoonoses)
From the context it's clear he is not implying that there are vertebrate animals on Mars. He just means an organism from Mars that is able to infect humans (a non standard use of the word or a metaphor).
John Rummel, NASA;s first planetary protection officer, said people have to have respect for the unknown.
People have to have some kind of respect for the unknown. If you have that respect, then you can do a credible job, and the public is well-served by your caution.”
(David, 2022, Controversy Grows Over whether Mars Samples Endanger Earth)
This topic of the potential for alien pathogens of human hosts, or indeed wildlife, seems a topic that needs more attention now that we are moving to a point where we will return our first samples from Mars about a decade from now.
Given the potential for unprecedented harm for human health if it turns out to be a "zoonosis to beat all others", I felt it was important to look at it more closely.
I worked up some preliminary scenarios for this. From these, it seems that alien pathogens could harm us in some scenarios. As an example, the way a fungus like Aspergillus fumigatus attacks us is very straightforward in principle, protects itself using EPS barriers, bioplastics essentially, and uses tendrils to inject other chemicals to attack and digest the organics. Its agnostic about our immune system and this same approach could be used by an alien fungus not even adapted to a pathogenic lifestyle in alien life.
I have not been able to find any other papers on the topic other than Lederberg's two papers. It has few cites and none of those expand on what Lederberg said as far as I can tell, or consider specific scenarios for alien life.
The scarcity of literature on alien biology and human health
Joshua Lederberg touched on this topic in two papers, neither of which refer back to any earlier papers on the topic:
- (Lederberg, 1999, Parasites face a perpetual dilemma) which has 8 cites in Google Scholar
- (Lederberg, 1999, Paradoxes of the host-parasite relationship) which has 17 cites in Google Scholar
Any paper on this topic would surely cite one or other of Joshua Lederberg's two papers.
My preprint with the literature survey is in both lists and there are a few other duplicates.
- Four other papers are common to both lists: (Profitiliotis, 2021, Planetary Protection Issues of Private Endeavours in Research, Exploration, and Human Access to Space: an Environmental Economics Approach to Forward Contamination) (Bagagli et al., 2008, Paracoccidioides brasiliensis: phylogenetic and ecological aspects), (Bagagli et al., Phylogenetic and evolutionary aspects of Paracoccidioides brasiliensis reveal a long coexistence with animal hosts that explain several biological features of the pathogen) and (Tsugushi et al, Riddles of Tuberculosis),
- The Tugushi paper in both lists is a duplicate of a paper in Japanese shown with a japanese title and also with an english title in both lists
Excluding my own preprint, Google Scholar turns up a total of 19 distinct cites.
Those lists are incomplete as they don't mention the ESF Mars sample return study which also cites Lederberg (Ammann et al., 2012, Mars Sample Return backward contamination – Strategic advice and requirements : 12) citing the first paper (Lederberg, 1999, Parasites face a perpetual dilemma) which brings the total up to 20.
It is possible that this list leaves out other modern papers that cite it but the literature can't be extensive. I have been unable to locate any papers or studies that add to Joshua Lederberg's work with investigations of their own and if anyone reading this knows of such a paper please alert me to it.
Sagan had to flip the War of the Worlds plot twist to warn about the low risk of harm from Mars microbes, and it remains a science fiction blind spot
Just about all the science fiction greats set stories on Mars. Examples include Arthur C. Clarke, Isaac Asimov, C. S. Lewis, Edgar Rice Burroughs, Robert Heinlein, H.G. Wells, Gregory Benford, Kurt Vennegut, Frederick Pohl, Ben Bova, Greg Bear, Ray Bradbury, Phillip K. Dick, Kim Stanley Robinson, Andy Weir, and Larry Niven amongst others.
None of these stories feature Martian life that harms Earth's biosphere. Indeed, as a science fiction enthusiast myself, I can’t think of even a short story that features native life returned from Mars that causes large scale harm to Earth’s biosphere or human health.
When Carl Sagan warned us of the risk from Martian life he needed to use the plot twist of the War of the Worlds in reverse. For some reason human science fiction authors don't seem to write much about harmful alien life.
"Precisely because Mars is an environment of great potential biological interest, it is possible that on Mars there are pathogens, organisms which, if transported to the terrestrial environment, might do enormous biological damage - a Martian plague, the twist in the plot of H. G. Wells' War of the Worlds, but in reverse. … The chance of such an infection may be very small, but the hazards, if it occurs, are certainly very high.
(Sagan, 1973, The Cosmic Connection - an Extraterrestrial Perspective)
The difference here is humans don’t get to write the story line for a real-life return of microbes from Mars.
Since then we only really have the “Andromeda Strain”, a very exotic imagined form of crystalline life, which by the end of the story rapidly mutates to be harmless (Andromeda strain (Bookrags, 2023, Andromeda Strain plot summary) (Santa Fe Institute, 2020, ‘Andromeda Strain’ series tackles astrobiology and life’s origins)..
If anyone reading this knows of any science fiction story that describes large scale harm to human health or Earth's biosphere, or a permanent change in the terrestrial biosphere based on microbes returned from Mars do contact me.
This seems important because science fiction authors often explore ideas in advance for their ethical and sociological implications, and this hasn't been done for ideas of large scale harm from a Mars sample return. This may also influence perceptions of harmlessness of Martian life by mission planners not familiar with the planetary protection literature.
How terrestrial organisms detect "non self" and relevance for alien life: our natural broad spectrum antibiotics only work for bacteria with cell walls with acid coatings, not fungi
I found a new approach that makes it possible to look at this issue even without any examples of an alien lifeform to test.
I look at recognition of “non self”. Our defences against harmful microbes have to distinguish between our cells and harmful cells. So I looked at how the natural antimicrobial peptides in our body’s first line of defences work.
Some of them are broad spectrum and work against bacteria. These would work against alien life if it has cell walls coated in acid groupings like bacteria but not if they have neutral cell walls like fungi or other eukaryotes.
There seems to be no particular reason why the cell walls of alien life would resemble bacteria more closely than fungi. Antifungals have to work in a narrower more specific fashion that is unlikely to work with an alien biology.
Text on graphic: Antimicrobial peptides (short protein chains). Our body’s first line of defence against microbes.
Recognizes “non self” as negatively charged cell walls (acids)
Very general but won’t work if alien life has neural cell walls
May work with some alien life.
Internal target structures.
Less likely to work with alien life.
(Martin et al., 2015, Antimicrobial Peptides in Human Sepsis : Figure 1)
Even microbial life might have no effective defences against alien fungi
Now lets look at fungal infections, including fungal infections of microbes, that have a mechanism that could be used by an alien microbe, to insert tendrils into a host from outside to extract its organics. The discovery of fungal infections of bacteria and other microbes is very new. This discovery is too recent to be covered in the 2009 Mars back contamination study. It’s an example of what John Rummel said:
We keep finding Earth organisms doing new things that are quite interesting from the standpoint of potential life elsewhere.
(David, 2022, Controversy Grows Over whether Mars Samples Endanger Earth)
Text on graphic: How chytrid fungi attack diatoms. Alien organisms could do the same, insert filaments to extract organics.
Description: false-colour red shows chytrid-like [zoo-]sporangium structures.
(Kilias et al., 2020, Chytrid fungi distribution and co-occurrence with diatoms correlate with sea ice melt in the Arctic Ocean : Figure 7)
Early research focused on fungal diseases of fresh water microbes. However more recently, scientist discovered that there are many fungal diseases of marine microbes too. They just found the freshwater ones first. A review from 2022 describes them in the title of the paper as a “mystery yet to unravel” (Ilicic et al., 2022, Basal parasitic fungi in marine food webs—a mystery yet to unravel)
If we did return an alien microbe that penetrated cells and organisms with filaments like fungi, and which has a neutrally charged cell wall, it might well be that we have no natural defences in our immune system. We’d then need to look at therapeutics to protect ourselves.
We might be able to develop drugs to target the alien biochemistry. However that might take longer than drugs to treat terrestrial pathogens because first we’d have to understand how the alien biochemistry works, which might take some time. There is some work on broad spectrum antifungals, similarly to our natural broad spectrum antimicrobial peptides.
Perhaps something similar would work for alien biology. The challenge, as for their use against terrestrial microbes, is to provide them in a way that avoids harming the host (Hancock et all, 2006, Antimicrobial and host-defense peptides as new anti-infective therapeutic strategies). Could we develop an analogue of the peptides that recognizes some feature of the alien biology and uses that to distinguish it from the neutrally charged eukaryotes?
Then we would also have the issue that the alien fungal analogue might attack terrestrial eukaryotes more generally. If it is able to attack humans, it’s not likely to be specific to us. So would we have to develop therapeutics to protect our cows, parrots, blue whales, etc., too? How could we administer them?
This example suggests a mixed picture. Some of our immune system’s recognition of “non self” might continue to work for some forms of alien life. But some forms of alien life might not be recognized by our immune system at all, if they happen to have accidental resemblances to features of terrestrial biology.
I also looked at fungal pathogens of blue-green algae. We likely have no reason to be concerned about viruses of alien microbes similar to bacteriophages. They would be adapted to hijack the cell machinery of an alien biology and are not likely to be able to attack our microbes.
However in the last few years researchers have found numerous fungal pathogens of microbes. This was a surprise discovery.
This discovery is too recent to be covered in the 2009 Mars back contamination study. It’s an example of what John Rummel said in the quote we just saw:
We keep finding Earth organisms doing new things that are quite interesting from the standpoint of potential life elsewhere.
(David, 2022, Controversy Grows Over whether Mars Samples Endanger Earth)
Researchers found these dark matter fungi as a result of advances in rapid gene sequencing. They called them “Dark Matter Fungi”, so called because they are not easy to study or cultivate (Grossart et al., 2016, Discovery of dark matter fungi in aquatic ecosystems demands a reappraisal of the phylogeny and ecology of zoosporic fungi). They are parasites of microalgae (phytoplankton).
Stages of infection of a freshwater cyanobacteria Anabaena macrospora by two species of chytrid fungi.
This is a discovery too recent to be covered in the 2009 Mars sample return study.
Fungal dark matter was discovered as a result of rapid gene sequencing, first as parasites of fresh water phytoplankton.
Scientists are now finding many fungal parasites of marine microbes too and call them "A mystery yet to unravel".
Terrestrial phytoplankton might have no defences against a novel fungal pathogen of martian cyanobacteria.
Suggestion: pathogens of an unrelated alien biology might also be able to infect terrestrial microbes in a similar way.
Scanning electron micrograph of possible chytrid fungi infecting diatoms
The main graphic is from here: (Gerphagnonet al., 2013, Fungal Parasitism: Life Cycle, Dynamics and Impact on Cyanobacterial Blooms : Figure 7)
The scanning electron microscope photo is from here, putative chytrid fungi coloured in pink false colour: (Kilias et al., 2020, Chytrid fungi distribution and co-occurrence with diatoms correlate with sea ice melt in the Arctic Ocean : Figure 7)
Early research focused on fungal diseases of fresh water microbes. However more recently, scientist discovered that there are many fungal diseases of marine microbes too. They just found the freshwater ones first. A review from 2022 describes them in the title of the paper as a “mystery yet to unravel”(Ilicic et al., 2022, Basal parasitic fungi in marine food webs—a mystery yet to unravel)
Microbes from Mars might be infected with fungal pathogens which could already be adapted to infect other microbes. Many of these diseases are chytrids (Ilicic et al., 2022, Basal parasitic fungi in marine food webs—a mystery yet to unravel), This class of fungi is best known for the amphibian fungal disease chytridiomycosis which is caused by the fungus (Batrachochytrium dendrobatidis), which had severe effects on many amphibian species around the world (Australian Government, n.d., chytridiomycosis). However it is also one of the main classes of fungi that attack microbes.
Chytrids have a fossil record that goes back half a billion years and are the simplest of the fungi (Roehl, 2016, Characteristics of Phylum Chytridiomycota). They are the only fungi with zoospores (“baby” fungi) that can swim to find a new host to infect using hair-like flagella to propel themselves. Some chytrid species live on dead organics, others are parasites of algae, microscopic worms, plants and amphibians. Some are useful in ecosystems because of the way they can break up cellulose, chitin and keratin (Roehl, 2016, Characteristics of Phylum Chytridiomycota).
Since Mars could have analogues of our blue-green algae (cyanobacteria), it’s reasonable to take the example of fungal infections of terrestrial blue-green algae. Cyanobacteria (blue-green algae) defend themselves from chytrid fungi similarly to the way the human immune system defends us from fungi, using peptides specific to this genus of fungi. The antifungals they use against chytrids are microcystins, microviridins, or anabaenopeptins.
In one study, researchers used genetic engineering to knock out the capability of a strain of the cyanobacteria Planktothrix to make these antifungals. When they did this, the cyanobacteria lost its resilience to the fungi. In one example the wild type cyanobacteria were completely immune to one of the chytrid strains they studied while it could infect all the cyanobacteria mutants even with just one of these classes of antifungals removed (Rohrlack et all, 2013, Putative antiparasite defensive system involving ribosomal and nonribosomal oligopeptides in cyanobacteria of the genus Planktothrix). So the cyanobacteria seem to need all three types of antifungal for protection against chytrids.
This suggests terrestrial blue-green algae might initially have no defences against a novel fungal disease of their analogues on Mars.
With the very rapid evolution of microbes we can expect them to develop defences rapidly. However this analogy would seem to suggest that initially they might have no defences against a novel fungal pathogen of an alien biology, in the worst case.
It does also suggest the possibility of fungal pathogens of our blue-green algae in the case where Mars has life related to terrestrial life.
Our immune system is likely to ignore alien fungi with unfamiliar patterns, and our immune system's natural antifungals may not work with alien life
I cover Aspergillus in my worked out scenario of a species from a novel fungal genus from Mars
Our body protects itself by using patterns that let it recognize the fungus. In a healthy immunocompetent person this is what happens in the lungs:
Text on graphic: Thousands of Aspergillus spores inhaled every day.
Our immune system has no broad-spectrum anti-fungals.
If the immune system recognizes the spores, most are trapped in the mucus and cleared by fine beating hairs.
Some spores get into the alveoli where oxygen exchanges with the lungs.
They are recognized and eaten by white blood cells.
This depends on recognizing the spores.
Problems arise if the immune system over-reacts or doesn't recognize the spores
Graphic from: (Palmieri et all, 2022, Recent Advances in Fungal Infections: From Lung Ecology to Therapeutic Strategies With a Focus on Aspergillus spp : figure 1)
Our immune system probably stops many fungal infections by recognizing particular patterns, the pathogen-associated molecular patterns (PAMPs). It likely does this using pattern recognition receptors (PRRs) which then trigger the immune response.
These are targeted to the molecular patterns from the most common fungi that attack humans, species from three genera: Candida, Aspergillus, and Cryptococcus with different molecular patterns specific to each genus (Kumar et al., 2018, Antifungal immune responses: emerging host-pathogen interactions and translational implications : table 1)
Looking at the two fungal genera that infect via the lungs, I have shown in bold the patterns and the receptors shared in common between the two genera:
Aspergillus fumigatus
PAMPs: β-1,3-glucan, chitin, galactomannan, DHN-melanin
PRRs:TLR2, CLRs (dectin-1, − 2, mincle, DC-SIGN), NLRs (NOD1, NLRP3), CR3, PTX3 MelLec
Cryptococcus neoformans
PAMPs: Mannose, capsular polysaccharide, glucuronoxylomannan
PRRs: TLRs (−2,-4), CLRs (dectin-2, MR), NLRs (NLRP3)
Suppose hypothetically that our human immune system only ever encountered Cryptococcus, and never encountered Candida or Aspergillus. It would have three pattern recognition receptors it could potentially use with Aspergillus,
PRRs: TLR2, Dectin-2 and NLRP3.
However, none of its acquired pathogen-associated molecular patterns would work with Aspergillus. It still wouldn’t see it.
PAMPs: None
If our immune system had only ever encountered Candida, it would have more chance of a response:
Aspergillus fumigatus
PAMPs: β-1,3-glucan, chitin, galactomannan, DHN-melanin
PRRs:TLR2, CLRs (dectin-1, − 2, mincle, DC-SIGN), NLRs (NOD1, NLRP3), CR3, PTX3 MelLec
Candida albicans
PAMPs: β-1,3-glucan, O-mannan, N-mannan, chitin, mannose
PRRs: TLR2, TLR4, CLRs (dectin-1, − 2, mincle, MR, DC-SIGN, Mcl), NLRs (NLRP3, 4,10), CR3, FcγR, galectin-3, MDA5
The worst case here is that our immune system might not have genus specific PAMPs for a martian fungus in a novel genus with a shared terrestrial biology. Perhaps with adaptations to three genera already we have a decent chance of some PRRs and PAMPs for a fourth genus of fungi from Mars, but we can’t guarantee it.
It’s not at all likely to have PAMPs for a fungus with a totally alien biochemistry.
So it seems indeed, that there is some potential that we might all be immunocompromised against a fourth opportunistically pathogenic genus of fungi from Mars. We are likely even more immunocompromised if challenged by fungi with a totally alien biochemistry.
The challenge for the immune system is to get the right balance between not responding to the alien exobiology at all and over responding in an allergic reaction.
If our immune system notices alien life it might trigger an allergic response
So far, I have found no discussion of the potential for allergic reactions to an alien biology in the planetary protection literature. It seems to deserve attention in future backward contamination studies. Putative martian life won’t be harmless if it can cause severe allergic reactions. In the worst cases these can even be fatal.
Our immune system has to make sure that its T cells don’t attack the body’s own cells, and don’t harm beneficial microbes either. One of many ways it does this is to use Treg cells that have an anti-inflammatory effect (Clark, 2010, Skin-resident T cells: the ups and downs of on site immunity)
The immune system is faced with the difficult problem of mounting immune responses to dangerous pathogens while maintaining tolerance to the body's own tissues and to harmless or commensal organisms. Regulatory T cells (Tregs) are one of many mechanisms developed by the immune system to enforce tolerance to harmless and self antigens.
These dampen down allergic responses to harmless microbes, for instance in the lungs the Treg cells prevent allergic responses to dust mites, Aspergillus fumigatus and plant pollen. Similarly Treg cells in the gut and other barrier tissues help to dampen down responses to the many different species of microbes we are exposed to (Atias et al., 2019, Mechanisms of human FoxP3+ Treg cell development and function in health and disease)
Our immune system has to clear the aspergillus microbes from our lungs, but at the same time it has to avoid over-reacting in a harmful inflammatory response.
This response is modulated by T-helper cells and almost all classes of T-helper cells are involved in this response and need to be finely regulated in a healthy individual. The most important ones for our adaptive immune response to aspergillus are the Th1, Th17, Th22, Th2, Th9, Treg and Tr1 cells
(Dewi et al., 2017, The multifaceted role of T-helper responses in host defense against Aspergillus fumigatus).
This graphic shows some of the complexities of the system of our immune response to Aspergillus at various stages of infection, and how cells of the immune cell including the T helper cells modulate the response.
Graphic from: (Dewi et al., 2017, The multifaceted role of T-helper responses in host defense against Aspergillus fumigatus: Figure 2) .
If our immune system encounters a novel or even completely alien genus of fungi, it might
- misrecognise the alien life as familiar and harmless, and dampen down the response so that it can't clear the microbes from our lungs, when our body really needs protection,
- fail to dampen down an allergic reaction to a harmless alien microbe.
So there is a delicate balancing act here. It’s not clear how our immune system learns to respond appropriately to harmless microbes with the allergen specific Treg cells. But there’s evidence children exposed to allergens early on in dairy farms are less likely to develop asthma, especially if they have early life exposure to hay, unprocessed cows milk, manure and contact with cows and straw (Deckers et al., 2021, Protection against allergies: Microbes, immunity, and the farming effect). If this can be generalized to life with an alien biology, then since we all have no previous exposure to alien life, all except the youngest children might be prone to allergic reactions to it. That’s assuming that our immune system is able to develop Treg responses to it to moderate the allergic response.
Allergic bronchopulmonary aspergillosis affects 2.5% of patients with asthma, and an estimated 4.8 million people globally. Chronic pulmonary aspergillosis (CPA) affects around 400,000 globally and only occurs in people who are not immunocompromised with symptoms of “weight loss, profound fatigue, productive cough, significant shortness of breath, and life-threatening hemoptysis [spitting out of blood from the lungs]” (Denning et al., 2013, Global burden of allergic bronchopulmonary aspergillosis with asthma and its complication chronic pulmonary aspergillosis in adults).
So far we’ve looked at the response to the alien invader itself.
Our immune system can also respond to the damage even if it can’t see the invader, with DAMPs (Damage Associated Molecular Patterns) a bit like the PAMPs mentioned above, but they respond to cells that get damaged, rather than the agents that damage them.
DAMPs help trigger inflammation, which can turn the area of your body red. That’s because of disease fighting cells leaking out of the blood stream into the surrounding tissue. However sometimes the inflammation can cause more damage leading to DAMPs responding to the damage caused by the inflammation they themselves triggered in a positive feedback loop leading to chronic inflammation (Cunha et al., 2012, DAMP signaling in fungal infections and diseases). DAMPs are involved in many chronic inflammation disorders (Roh et all., 2018, Damage-associated molecular patterns in inflammatory diseases).
DAMPS are also involved in sterile inflammation, inflammation caused by over reaction to non living particles, such as the reaction to silicon particles in silicosis. Detailed imaging shows the white blood cells called macrophages try to destroy the silica particles, and fail, which damages the white blood cells. That leads to the inflammation response and feedbacks leading to the chronic disease (Kazazian, 2014, NLRP3 inflammasome activation by crystal structures). We also get sterile inflammation to other non living particles such as urea crystals (in gout) and microplastics.
So this seems another possible scenario, that the alien biology completely ignores our biology but the alien microbes either contain material our white blood cells can’t destroy, or perhaps a hard coating to protect themselves from martian dust-storms. They could lead to an inflammation response without any direct harm. In this scenario our immune system harms itself in its attempts to attack them.
Obstacles to developing tests for a novel fungal disease based on an alien biology and developing antifungals for it
Often aspergillosis isn’t the first guess of the doctor. Chronic pulmonary aspergillosis (CPA) has high mortality within 5 years, and is often confused with tuberculosis. It looks similar to tuberculosis in medical images of the lungs, and also clinically (Brown et al., 2015, Hidden killers: human fungal infections : 6). It can be distinguished from tuberculosis by testing for antibodies to Aspergillus.
There are many marketed tests now, but they are still not 100% reliable. The ELISA IgG antibody tests for CPA vary in accuracy but on average they are 93% reliable (sensitivity) but with 3% false positives (97% specificity) (as of 2020). One of the issues here is distinguishing between harmless colonization and the disease (Chaves et al., 2020, Accuracy of serological tests for diagnosis of chronic pulmonary aspergillosis: A systematic review and meta-analysis).
Fungi are evolutionarily closer to humans than most microbes, which makes it harder to develop antifungals. The introduction of echinocandins and third-generation triazoles improved the options for antifungal therapy but they initially had modest success in preventing death from fungi (Brown et al., 2015, Hidden killers: human fungal infections : 6).
We now have many modern anti-fungals and many of them target processes unique to fungi. As if writing this the list is Polyene, Azole, Allylamines, Echinocandins, Griseofulvin, and Flucytosine.Each of those refers to a class of antifungals which operate in different ways in a broad spectrum way against a wide range of fungi though not all (e.g. azoles don't work with Aspergillus). However it typically takes 12 years from discovery to dispensing a new medicine (McKeny et al., 2023, Antifungal antibiotics)
This would surely be accelerated if we had to find an antifungal quickly for an alien fungus that attacked humans, as happened with the COVID vaccines. However, as for COVID, it might still take some time before we have those anti-fungals, or before we have diagnostic tests that can be used to recognize the signs of presence of the fungus in a patient’s lungs. It might take a longer time with an alien fungus than for COVID given the challenges of a completely novel fungal genus, especially if it involves an alien biology.
This would surely be accelerated if we had to find an antifungal quickly for an alien fungus that attacked humans, as with the accelerated development of the COVID vaccines. However, as for COVID, it might still take some time before we have those anti-fungals, or before we have diagnostic tests that can be used to recognize the signs of presence of the fungus in a patient’s lungs. It might take a longer time to develop an effective response than for the COVID pandemic, given the challenges of developing an anti-fungal for a novel fungal genus. We probably wouldn’t be able to use vaccines. We don’t yet have vaccines that work with fungi though there is work in progress to develop them (Avina 2023, Breakthroughs and Challenges in Fungal Vaccine Development). Even once we have vaccines for terrestrial fungi, vaccines can’t work if our immune system has no way to recognize the alien fungus.
Nanoplastics and microplastics as an analogue of dormant alien life that accidentally triggers blood clots
if the two forms of life are radically different, perhaps neither form of biology has any adaptations to recognize the other form of biology as ether something to eat or to protect itself from. In that case perhaps they may just co-exist peacefully each form of life unaware of the existence of the other at the immune system or metabolism level. Just an obstacle in the environment to avoid like a speck of dirt. However, as usual we need to look at worst case scenarios for planetary protection. Even this possibility may not be as benign for terrestrial life as it seems.
We can use nanoplastics and microplastics as an analogue of alien life that may not even notice terrestrial life. Though microplastics and nanoplastics don’t attack our bodies and can’t reproduce they can impact on our immune system in various ways, including coronas, the blood plasma sticks to nanoparticles of some types of plastic, in this case polystyrene
Text on graphic: Microplastic. Blood plasma sticks to the microplastic. Corona can pick up fragments of pathogens. This can trigger an inflammation response.
These can then coagulate to form blood clots:
Text on graphic: Plasma coronas can cause the microplastics to stick together and form blood clots
These figures are from: (Gopinath et al., 2019, Assessment on interactive prospectives of nanoplastics with plasma proteins and the toxicological impacts of virgin, coronated and environmentally released-nanoplastics : figure 7)
We don’t get enough blood clots from microplastics and nanoplastics to be a concern. But this analogy suggests as a possibility to consider, that if some time in the future Earth’s environment is filled with large numbers of alien microbes we might get significant levels of blood clots from them in our blood.
Possibilities for symbiosis between terrestrial and alien life with multi-genesis microbiomes, biofilms, lichens or microbes, even multi-genesis microbes similar to eukaryotes which may open the door to multi-genesis multicellular life
If neither form of biology has any adaptations to recognize the other form of biology as either something to eat or to protect itself from, there are several other possibilities here. Some martian microbes might survive competition with terrestrial life, for instance if it is better at photosynthesis. Or the other way around some terrestrial life could survive competition with martian life. That could lead to multi-species microbiomes or multi-species biofilms.
That leads to the possibility that these multi-genesis biofilms are better adapted to martian conditions than the martian biofilms already there or introduced purely terrestrial biofilms, especially given that one form of propagation on Mars might be fragments of biofilms broken off and dispersed in the dust storms. See Mosca et al's scenario above:
Multi-genesis biofilms might also be better adapted to Earth than terrestrial biofilms.
Another possibility is that the mix might lead to multi-genesis symbioses. Perhaps we could get a lichen-like organism with a martian algal analogue component and a terrestrial fungal component or vice versa.
Finally, speculatively, the most interesting and radical symbiote. All terrestrial multi-cellular life is based on eukaryotes, cells with a nucleus with complex organelles inside that perform various tasks. Some of these, the mitochondria (which produce energy from oxygen) and the chloroplasts (in plants, which produce oxygen) have their own independently evolving DNA. These seem to have evolved from ancient cells without a nucleus, prokaryotes that got incorporated in the original precursor cell, which was unable to use oxygen by itself. For details of the cell structure for eukaryotes see (Cooper, 2000. The Cell: A Molecular Approach: Eukaryotic cells : figure 1.7)
Could martian and terrestrial microbes that don't recognize each other as life, be so compatible that they can form similar symbiotes, with terrestrial microbes living inside martian microbes or vice versa? Could these even lead to new forms of multi-genesis - multi-cellular life?
We would need to be very sure that we are happy with any consequences before making the bold experiment of creating a mixed genesis biosphere on either Mars or Earth, with possibly multi-genesis microbiomes, biofilms, lichens, or even new forms of multi-genesis microbe that may most speculatively have capabilities to evolve into new forms of multi-cellular life.
Dormant alien life could still cause sterile inflammation like silicosis or severe allergic reactions
These coronas can also pick up fragments of pathogens. The immune system can respond to those with antibodies and try to get rid of the microplastics as if they were pathogens, which leads to sterile inflammation I.e. inflammation triggered by something harmless.
We might also get sterile inflammation responses to alien life even if it doesn’t have a blood corona. This is similar to the sterile inflammation of gout (responding to harmless urea crystals) or silicosis (responding to harmless silica particles). (Yang et al., 2022, Impacts of microplastics on immunity).
More generally, as with the allergic reactions to Aspergillus, we might be allergic to harmless alien microbes. These allergic reactions might sometimes be serious.
Perhaps sterile inflammation from microplastics is our closest analogy to sterile inflammation from a mutually mystified alien biology (Yang et al., 2022, Impacts of microplastics on immunity). It’s broadly similar to other forms of sterile inflammation as discussed in the previous section. This figure shows one proposal for what may be happening in detail with microplastics. The reactive oxygen species, DAMP, inflammation and cell death are all detected but the other details need to be clarified.
(Yang et al., 2022, Impacts of microplastics on immunity : Figure 4)
First, coated microplastics (MP) shown in blue get taken up by the white blood cells (macrophages). The microplastics interfere with the mitochondria, the energy powerhouses of the cell that turn oxygen into energy. This leads to a build up of very reactive highly oxygenated chemicals like peroxides, perchlorates, etc., which leads to oxidative stress Next in response to the oxidative stress, the white blood cell may self destruct (apoptosis or programmed cell death)
The DAMPs then may be activated by the damage to the white blood cell, which triggers an inflammation response. When the white blood cell breaks open, it may release the microplastics to start the process again
Alien amino acids could be misincorporated into proteins like BMAA leading to protein misfolding and accidental neurodegenerative disease
I look at the possibility that an alien lifeform with novel amino acids might cause protein misfolding similarly to the misfolding that results from incorporating BMAA in place of serine.
An extraterrestrial biology could have proteins built up of many more amino acids than the 20 encoded in RNA and used to build proteins in terrestrial biology. There are 140 that are never used in proteins but occur elsewhere in terrestrial biology. Extraterrestrial biology might encode for any of those and use them in proteins (Ambrogelly et al., 2007. Natural expansion of the genetic code). A computer search turned up nearly 4,000 biologically reasonable amino acids (Meringer et al., 2013, Beyond terrestrial biology: Charting the chemical universe of α-amino acid structures) (Doyle, 2014, Mapping Amino Acids to Understand Life's Origins). Many of those won’t occur in nature, but terrestrial biology also includes non natural amino acids. Meanwhile also many of the natural amino acids don’t occur in terrestrial biology and might potentially be used in extraterrestrial biology. 52 amino acids have been identified in the Murchison meteorite (Cronin et al., 1983. Amino acids in meteorites).
Some of these novel amino acids might be like BMAA and bind to transfer RNA (Sen, n.d., Transfer RNA (tRNA)) for a similar amino acid, through accidental similarities and so get misincorporated. Like this:
Video: From DNA to protein - 3D
Frame from here
How the transfer RNA molecules work is explained here in the video
For a video that shows more realistic shapes for the molecules see: From DNA to Protein
This may be a contributing cause to neurodegenerative diseases such as ALS which Steven Hawking suffered from, as it can bind to serine transfer RNA and so get misincorporated into proteins in place of serine (Holtcamp, 2022, The emerging science of BMAA: do cyanobacteria contribute to neurodegenerative disease?).
An alien biochemistry could have many beneficial bioactive compounds but also many accidental toxins
We could expect an unrelated exobiology to produce novel bioactive compounds, since that is what life need to do to survive, grow, and reproduce. These could harm us or help us. Let’s look briefly first at some of the ways they can help us.
Many modern medicines are based on bioactive compounds from microbes (Abdel-Razek et al., 2020. Microbial natural products in drug discovery). That can include medical use of human toxins produced by microbes, for instance botulism toxin, properly used, has many medical benefits (Jankovic, 2004. Botulinum toxin in clinical practice).
Martian life could benefit industry. For instance aspergillus niger, a bacteria whose natural habitat is soil and decaying vegetation, is used for industrial production of citric acid for beverages, food, detergents, cosmetics and pharmaceuticals (Behera, 2020, Citric acid from Aspergillus niger: a comprehensive overview).
Extremophile fungi may be a source of bioactive compounds for medically useful drugs (Chávez et al., 2015, . Filamentous fungi from extreme environments as a promising source of novel bioactive secondary metabolites).
There are many other ways a novel biology could benefit humans and our biosphere. I cover this in the preprint:
(Walker, 2023, So many serious mistakes in NASA's Mars Samples Environmental Impact Statement it needs a clean restart) (docx) : NEW: Enhanced Gaia - ways that introduced martian life could be beneficial to humans, ecosystems and Earth’s biosphere)
However for the topic of back contamination and what we need to do to protect Earth, what matters is whether it can also harm us.
Terrestrial; bioactive compounds for medicine have to be screened for toxicity (Madariaga-Mazón et al., 2019, Toxicity of secondary metabolites). The situation may be the same with an alien biology. Some of its bioactive compounds may be beneficial, some useful in industry, some useful in medicine, some toxic but still useful in small doses in medicine and some might be very harmful. There are many types of accidental toxins produced by terrestrial biology and the same may be true of alien life.
Some of the ways alien life might accidentally harm us include:
- Allergens (already covered with the example of Aspergillus)
- Secondary metabolites, e.g. which inhibit the growth of other microbes, Wallemia which is adapted to low water activity in salt or sugary solutions spoils food with secondary metabolites, the most toxic is wallimidione (Desroches et al., 2014, Extrolites of Wallemia sebi, a very common fungus in the built environment). As another example, Aspergillus species produce numerous toxic secondary metabolites (Pfliegler et al., 2020, The Aspergilli and their mycotoxins: Metabolic interactions with plants and the soil biota)
- protoxins, which when metabolized break down into toxic products, such as methanol which is converted into toxins when digested (Mégarbane et al., 2005. Current recommendations for treatment of severe toxic alcohol poisonings) or hypoglycin A, which is broken down into the highly toxic MCPA-CoA on digestion and can lead to the fatal Jamaican vomiting sickness after eating the unripe fruit of the Ackee tree, a national foodstuff in Jamaica (Holson, 2015, Ackee Fruit Toxicity)
- The chemistry of alien cells may itself be toxic to Earth life. martian life might use hydrogen peroxide and perchlorates in its intracellular fluids in place of the chlorides used by Earth life, similarly to the composition of the brines it inhabits (Schulze-Makuch et al.. 2010. A perchlorate strategy for extreme xerophilic life on Mars)
- As well as the internal chemistry, the waste products and the various chemicals that are produced by alien life in the process of digesting organics (metabolic intermediaries) could also be accidentally toxic or allergenic.
- Any of these could also be accidentally carcinogenic.
- Life based on an alien biology in the gut microbiome could impact on our ability to digest food and on human health.
So, even if we find unrelated Martian organisms, it would be no great surprise to find they produce bioactive compounds which affect humans in various ways, sometimes beneficial, sometimes harmful.
Whether we want to introduce martian life itself to Earth would depend on what the effects would be on our biosphere and on humans and the animals within it.
One scenario is that novel martian life has potential for mixed effects on Earth’s biosphere, with some positive effects, and some negative. If so, we might prefer to leave it on Mars and exploit it on Mars and export only the products of martian life to Earth, even if the life itself could also lead to some benefits on Earth.
In a situation where we believe martian life will have mixed effects, we will have a difficult decision about whether or not to return the life itself to Earth. That’s especially so if it affects some ecosystems or some human communities positively and some negatively.
Probably many would agree it is not ethical to return something that has a risk of significant harm to a fraction of the world population, even if overall it’s a benefit.
Worst case scenario where terrestrial life has no defences to an alien biology - humans survive by ‘paraterraforming’ a severely diminished Gaia
The physicist Claudius Gros looks at a clash of interpenetrating biospheres in his paper on a "Genesis project" to develop ecospheres on transiently habitable planets. Gros reasons that the key to functioning of the immune system of multicellular organisms, plants or animals, is recognition of “non-self”. He presumes that biological defense mechanisms evolve only when the threat is actually present and they don’t evolve to respond to a never encountered theoretical possibility ((Gros, C., 2016. Developing ecospheres on transiently habitable planets: the genesis project)
“How likely is it then, that ‘non-self’ recognition will work also for alien microbes?"
"Here we presume, that general evolutionary principles hold. Namely, that biological defense mechanisms evolve only when the threat is actually present and not just a theoretical possibility. Under this assumption the outlook for two clashing complex biospheres becomes quite dire."
"In the best case scenario the microbes of one of the biospheres will eat at first through the higher multicellular organisms of the other biosphere. Primitive multicellular organisms may however survive the onslaught through a strategy involving rapid reproduction and adaption. The overall extinction rates could then be kept, together with the respective recovery times, 1–10 Ma, to levels comparable to that of terrestrial mass extinction events."
"In the worst case scenario more or less all multicellular organism of the planet targeted for human settlement would be eradicated. The host planet would then be reduced to a microbial slush in a pre-cambrian state, with considerably prolonged recovery times. The leftovers of the terrestrial and the indigenous biospheres may coexist in the end in terms of ‘shadow biospheres’ "
In such a worst case, where terrestrial life is naive and offers no resistance when eaten by Martian life, after a clash with life from an alien biosphere, almost all multicellular organisms on Earth could be eradicated. All that would be left would be some small rapidly evolving organisms.
Even microbial life might offer no resistance to start with though with its shorter generation time it has more chances to evolve resistance faster as we saw above
This is an argument similar to the worst cases of Lederberg, Rummel and Sagan (Lederberg, 1999b. Parasites face a perpetual dilemma) (Sagan, 1973, The Cosmic Connection - an Extraterrestrial Perspective : 162) (Meltzer, 2012. When Biospheres Collide: A History of NASA's Planetary Protection Programs).
Even in this scenario, it would be possible to preserve higher life in enclosed habitats protected by use of technology. The habitats could have self contained biospheres based on plants grown for food, and oxygen, which in turn take up carbon dioxide and water from humans. This seems feasible as we have already designed almost completely self-sustaining habitats that should work in space, a more challenging situation (Salisbury, 1997. Bios-3: Siberian experiments in bioregenerative life support).
So long as our seed banks were protected from the invasive Martian life, whatever it is, we could gradually re-establish plant life inside these habitats too, and populate our habitats with any animal life rescued from deteriorating ecosystems. The seed banks preserve most plant species (apart from some tropical plants such as mangoes which can’t be preserved for long as seeds). Eventually much of the world could be covered in expanding joined together habitats in a process similar to paraterraforming. Perhaps a similar process would work for parts of the sea bed too, and the sea shore
In this way, at least some humans could survive any of these scenarios. However in this worst cases, our biosphere would be severely affected, for a significant period of time.
This scenario and some of the previous ones such as the introduction of mirror life may seem like a scene from a science fiction book or movie. Hopefully that is exactly what they are. Hopefully these are not future possibilities.
However, the idea of returning a sample from Mars itself would seem a science fiction scenario as recently as the early 1960s. We are entering a future where what used to be science fiction is becoming a reality, and we have to seriously consider real world outcomes from such scenarios. Unlike a movie script, we can’t rewrite this story to a happy ending if we don’t like the outcome.
Our intuitions about what is credible or incredible based on past experience can easily lead us astray in novel situations like this, never encountered by any previous human civilization.
Humans wouldn't go extinct in such a scenario, as we would have time to recognize what is happening and build habitats to survive in. Also, we would be able to preserve much of the Earth’s biodiversity including all the plants with preservable seeds (which is most of them). However such a paraterraformed Earth would severely diminish life prospects for several generations.
Eventually life outside the habitats would reach an equilibrium, with small microscopic single cell and multicellular terrestrial lifeforms able to evolve fast enough to take advantage of the new microbial environments. Over millions of years, perhaps faster with assistance from humans, there would be higher life forms again able to survive in an environment with both kinds of biology. Perhaps humans also could artificially adapt our progeny to survive outside the habitats or find ways to supplement their own immune systems so that they are protected from the extraterrestrial microbes that our naive immune systems don’t recognize as life. But essentially this process would turn Earth into an alien planet for macroscopic terrestrial biology in its current (original) form.
Although we have technology we could use to survive this scenario today, it would have been much harder with the early technology of the 1960s. The first “bubble boy” David Vetter who lived his life in an isolation room was born in 1971 (Gannon, R., 1962. Life in a Germfree World). Without experience of such technology, it would be that much harder for 1960s humans to survive back contamination of Earth’s biosphere with life that our biology is not able to protect itself against naturally.
We can't know, but we may be lucky with our Moon, that there was no extraterrestrial life there. This might be an extinction risk that extraterrestrials have already encountered at a similar technology level to 1960s humans.
If an intelligent alien species returned alien life to their planet with inadequate planetary protection, at the level of technological development of the Apollo mission, they could go extinct. They might not manage to develop the technology for self-sustaining habitats in time to keep out the alien microbes. It’s not impossible that this has already made some other alien intelligent species extinct on one of the billions of exoplanets in our galaxy or in the billions of other galaxies in the observable universe. That's especially possible since on Earth humans are an exceptionally versatile omnivore that can live almost anywhere with basic technology and find something to eat or grow almost everywhere on our planet. Other intelligent alien species might have much more limited nutritional and environmental requirements and be more at risk from their first sample returns from a nearby alien biosphere.
⸽ Many ways martian microbes could be harmless to terrestrial organisms as dandelions in the USA
This doesn’t mean that there is a high risk for life from Mars. Expert opinion seems to be that most likely Mars doesn’t have any life at Jezero crater. Even if we do return martian microbes accidentally, many introduced species are harmless or beneficial, or cause very little harm. An example would be the dandelion in the USA; it is only listed as an invasive species in Alaska and Oregon. In most states it doesn’t harm American ecosystems, it just adds to the biodiversity.
Although they may be tough to control in a traditional lawn, they are only listed as an invasive species in Alaska and Oregon. In most areas of the country there is a very low risk to pushing out native species and they provide no real damage to the ecosystem. In fact, they are an excellent food source for many of the native bees, insects and other wildlife.
(James River Association, 2021. Native, Non-Native, Invasive - and Dandelions! )
Text on graphic: If Mars has native microbial life, it may well be harmless to Earth like dandelions in most of the USA:
… no real damage to the ecosystem. In fact, they are an excellent food source for many of the native bees, insects and other wildlife.”
But they could be pests for agriculture like the Giant African Snail - or harmful to humans or the environment - we need to know, not guess.
(Monteregina, 2021. Dandelion - Dent-de-lion ou pissenlit)
(Jenner, 2010. Achatina fulica [Giant African Snail] in Ubud, Bali)
Even the dandelion does cause harm in Alaska because it can crowd out native plants because it grows faster than them in the short growing season (National Park Service, n.d., Alaska)
It is striking that identified human microbial diseases are all bacteria or eukaryotes (e.g. fungi). Earth’s third domain of life, the archaea, are not known to cause diseases in humans, animals or plants. The archaea could be implicated as opportunistic pathogens in some diseases like tooth decay, and diverticulosis, but the evidence is circumstantial. The archaea are present but it’s not clear they are a cause (Kumondorova et al., 2019. Archaea and their potential pathogenicity in human and animal diseases) (Chong, J., 2017, Comment: Archaea: closet pathogens?)
Whether or not there are genuine archaeal diseases, this example suggests it is possible that an entire domain of life from Mars could be harmless, even beneficial, to terrestrial life. After all, a microbe normally has no incentive to harm its host. Although this is not true for all diseases (polio, and smallpox are examples or diseases that have never evolved to be less deadly), for most microbes, keeping its host alive is its priority and harming its host is maladaptive {Chong, J., 2017, Comment: Archaea: closet pathogens?).
Interestingly, archaea are more closely related to animals and plants than bacteria, though less closely related to them than fungi. It seems that an evolutionary distance for Martian microbes would be no protection, nor would evolutionary closeness be protection either.
That leads to an interesting question, if we find a new domain of life on Mars that we believe may be harmless to terrestrial life, how could we prove it? How could we prove the archaea to be harmless, in a hypothetical scenario where we introduce them to Earth from Mars for the first time this century? It wouldn't be possible to test interactions exhaustively, though some of the most important interactions could perhaps be tested in experiments, also how would one predict how it could evolve?
This is a question we may have to address at some point in the future, for instance if we find related life on Mars but in a new domain not yet present on Earth.
Ways that martian life could be at the same level of evolution as terrestrial life or more highly evolved
At first sight, terrestrial life seems likely to have a more complex genome and to have much larger vocabulary of metabolic pathways, enzymes and other adaptations it can draw from because it comes from a planet that likely has many more species and much larger numbers of microbes per species. This would both increase genetic diversity and help to speed up evolution.
However there may be a few points in the favour of faster evolution on Mars.
- The ionizing radiation would speed up evolution if martian life is able to tolerate the faster mutation rate. If martian life responds by improving error correction this might have no effect.
- Rapid climate change may stimulate evolution. For example there's evidence that the first complex multicellular life such as sponges might have evolved to feed on the abundant planktonic marine algae between two "snowball" Earth phases (Sturtian and Marinoan) after oceans were flooded by nutrients from the melting glaciers. Jarrett, co-author of the study, put it like this:
QUOTE “In these rocks we discovered striking signals of molecular fossils. We immediately knew that we had made a ground-breaking discovery that snowball Earth was directly involved in the evolution of large and complex life,”
(Lazarro, 2017. ‘Rise of Algae’ in Cryogenian Oceans Led to Appearance of First Complex Organisms, Study Says)
Paper: (Brocks et al., 2017, The rise of algae in Cryogenian oceans and the emergence of animals)
Mars seems to have had frequent rapid warmings and coolings from the geological record. We cover these above in:
Vulnerability of such a treasure as early pre-Darwinian life to terrestrial life - Martian life might just have an intrinsically faster evolutionary rate. As we saw above in: Exploring ways that Mars could still have early life or prebiotic chemistry , Sharov et al. graphed genetic complexity of non redundant nucleotides against the time of origin of an organism. The complexity of the most complex organism increased 7.8 fold in complexity every billion years (Sharov, 2006. Genome increase as a clock for the origin and evolution of life).. However, the most complex archaea increased only 1.9 fold every billion years and the most complex Eubacteria increased only 2.5 fold every billion years (Sharov, 2006. Genome increase as a clock for the origin and evolution of life).
There might be even more variation between life of different origins. For instance martian life might focus more on accommodation of errors than correction of errors compared to terrestrial life which might permit faster evolution. Or vice versa. - As we saw, Carl Woese suggests that the earliest cells might have had no intercellular competition and only competition of the genes perhaps enclosed in vesicles. It might be plausible that this would lead to a faster rate of evolution of the genome, and if so the complexity of martian life might be enhanced if it spent a longer period in the pre-Darwinian phase of evolution.
See above: Vulnerability of such a treasure as early pre-Darwinian life to terrestrial life
One could likely think of other arguments either way. The short answer is we don't know. However it is not implausible that some martian life is as complex and highly evolved as terrestrial life. It could be more complex and have explored pathways that terrestrial life has never explored.
It would also be adapted to martian conditions for billions of years. That would lead to different selection pressures.
We will look at one of these possibilities in the next section. It's possible that photosynthesis evolved differently on Mars, but as a result of adaptations to very low light levels they may also be able to do better in terrestrial conditions too.
Martian microbes better adapted to terrestrial conditions than terrestrial life, example of more efficient photosynthesis
Alien life doesn’t have to invade our bodies, or even create accidental toxins to harm us. It can also harm by competition with our microbes. To take an example, photosynthetic life on Earth operates at well below its theoretical peak efficiency for photosynthesis. Martian photosynthesis could be more efficient than terrestrial photosynthesis.
Martian life would then be like an invasive weed. If the result isn’t a “drop in replacement” for the photosynthetic life already in our ecosystems this might change how the ecosystem functions, which could be beneficial or harmful, but it would be different.
Photosynthesis proceeds through the reaction: (Melis, 2009. Solar energy conversion efficiencies in photosynthesis: minimizing the chlorophyll antennae to maximize efficiency)
CO2 + H2O + 8 hv ⟶ (⅙) C6H12O6 + O2
The coefficients here are moles, so this means that with 8 moles of photons are needed for conversion of one mole (44 grams) of CO2 and one mole (18 grams) of H2O into a sixth of a mole (30 grams) of C6H12O6 biomass and one mole (32 grams) of O2.
One way to measure the efficiency is to measure the evolved oxygen for given levels of sunlight. At low light levels, the green alga chlorella vulgaris is able to achieve 84% efficiency at using photons to generate oxygen, absorbing 9.5 moles of photons for each mole of evolved oxygen.
However the efficiency rapidly falls as the light intensity increases, eventually saturating at less than half full sunlight intensity and producing no more oxygen as the sunlight levels increase further (Melis, 2009. Solar energy conversion efficiencies in photosynthesis: minimizing the chlorophyll antennae to maximize efficiency : 273). This graph from the paper shows how saturation is reached in measurements of a wild-type microalgae
[Figure needs permission]
Empirical data from wild-type microalgae. The vertical axis is the amount of oxygen produced per second by each mole of chlorophyll. It starts below zero because in the dark, respiration consumes about 3 mmol of oxygen / mol Chl / sec
The oxygen production increases linearly up to about 400 μmol m⁻² s⁻¹ of light, and then it saturates. After about 1000 μmol m⁻² s⁻¹ no more oxygen can be produced by increasing the light intensity. Full sunlight is 2200 to 2500 μmol m⁻² s⁻¹ (or 2.2 to 2.5 mmol m⁻² s⁻¹)
This is due to limitations in the speed of photosynthesis.[ Figure 1 of (Melis, 2009. Solar energy conversion efficiencies in photosynthesis: minimizing the chlorophyll antennae to maximize efficiency : 273)
There are other losses in cellular processes and inefficiencies in photosynthesis, with the result that only part of the energy from photosynthesis is converted into usable energy by the algae. The theoretical maximum is that 8-10% is converted into biomass in conditions of full sunlight (Melis, 2009. Solar energy conversion efficiencies in photosynthesis: minimizing the chlorophyll antennae to maximize efficiency : 274)
The best case scenarios in labs and small scale microalgal cultivation achieve 3% efficiency under normal illumination (Melis, 2009. Solar energy conversion efficiencies in photosynthesis: minimizing the chlorophyll antennae to maximize efficiency : 274).
This low efficiency is due to the large numbers of chlorophyll antenna molecules attached to each reaction center, to absorb the light. At high light levels, terrestrial life absorbs much more light than it can process, which it has to re-radiate it as heat or photoluminescence. A smaller antenna size with fewer molecules per reaction center means light can penetrate deeper into a culture at the same cell density and more of the light is used at high light levels. Cultures with a smaller antenna size use less chlorophyll antenna molecules, so are lighter green at the same cell density(Schenk et al., 2008. Second generation biofuels: high-efficiency microalgae for biodiesel production : 37)
According to Mellis, it would be possible to increase the typical 3% efficiency of green algae another three fold, close to the theoretical maximum of 8 to 10% by truncating the light-harvesting chlorophyll antenna size (Melis, 2009. Solar energy conversion efficiencies in photosynthesis: minimizing the chlorophyll antennae to maximize efficiency). Experiments back this up, though with smaller improvements (instead of tripling, they achieve modest increases of 55% to 60%) (Kirst et al., 2014. Maximizing photosynthetic efficiency and culture productivity in cyanobacteria upon minimizing the phycobilisome light-harvesting antenna size).
So, why do terrestrial microbes capture more light than they need, shading other cells even of their own species, that would be able to use the excess light? It might be to inhibit competition from other species, that at high light levels a phototroph captures light that would otherwise be used by competing phototrophs. Also a larger antenna size allows it to capture more light at lower light levels with lower cell densities (Ort et al., 2015. Redesigning photosynthesis to sustainably meet global food and bioenergy demand : 8530) (Negi et al., 2020. Light regulation of light ‐ harvesting antenna size substantially enhances photosynthetic efficiency and biomass yield in green algae : 15).
Reducing light antenna size has a trade off. A small antenna with fewer chlorophyll molecules increases efficiency at high light levels but if the cell density is low it reduces the efficiency at low light levels.
However modified cells have been designed that adjust the antenna size depending on the light intensity so that they achieve high efficiency both at low and high light levels compared to wild-type strains, doubling and even tripling the yields of the wild-type strains (Negi et al., 2020. Light regulation of light ‐ harvesting antenna size substantially enhances photosynthetic efficiency and biomass yield in green algae : 15)..
A Martian photosynthetic organism would experience large changes in light levels with a need to capture light during dust storms if possible, and also to capture as much as possible during conditions of bright sunlight, so it might already have an adjustable antenna size.
There are many other points in this complex process of oxygenic photosynthesis where efficiencies can be increased in principle.
Terrestrial photosynthesis rejects 50% of the incoming sunlight, mainly in the red part of the spectrum, leading to the distinctive “red edge”. The purple bacteria and lichens don’t have this “red edge” and Martian life would be likely to use red light like the purple bacteria, because of the high absorption of blue light by dust (Kiang, 2007, The Color of Life, on Earth and on Extrasolar Planets)
Oxygenic photosynthesis goes through two photosystems, 1 and 2, and both use the same frequencies of light. The efficiency could be doubled by using red light for one of the two systems (Blankenship et al., 2011. Comparing photosynthetic and photovoltaic efficiencies and recognizing the potential for improvement : 808).
Martian life might also be able to use the full range of the spectrum. Terrestrial seaweeds are dark brown in colour because they use accessory pigments like fucoxanthin to gather the blue-green component of light rejected by chlorophyll. These then transfer the energy to the chlorophyll and so to the photosynthetic reaction centers. They do this so that they can use sunlight at only 1% of surface levels so it helps to use the blue-green light that passes through seawater (Caron et al., 2001. Light harvesting in brown algae)
There is no need for terrestrial plants to do this because they already get more light than they can use for photosynthesis. However, a hypothetical Martian microbe with faster photosynthesis might find it useful to capture the full spectrum, especially in the low light levels on Mars. This is another way to double its theoretical efficiency compared to most terrestrial photosynthesis.
Oxygenic photosynthesis also uses the Calvin cycle. This has evolved only once. All the organisms with the capability for oxygenic photosynthesis belong to a single clade, all evolved from a single hypothetical ancestor. This is the least efficient of the six known pathways for carbon fixation, both in terms of energy, and in terms of the number of electrons needed for each mole of fixed CO2 (Bains et al., 2016. The cosmic zoo: the (near) inevitability of the evolution of complex, macroscopic life).
So, why is terrestrial oxygenic photosynthesis so inefficient? Perhaps it is just hard to evolve this form of carbon fixation? Bains et al suggest this may be a many pathways event. Perhaps oxygenic photosynthesis could evolve in many ways, but with very low probability of achieving all the necessary steps so terrestrial life only happened to evolve it once.
Bains et all suggest as a perhaps more plausible alternative, that it could be a "pulling up the ladder" event where once the niche was filled for a photosynthesizer not limited by the need for an electron donor such as sulfide, Fe(II) or hydrogen, it was hard for a new photosynthesizer to evolve again (Bains et al., 2016. The cosmic zoo: the (near) inevitability of the evolution of complex, macroscopic life).
Either explanation would let Martian photosynthesizers achieve a more efficient form of photosynthesis than we have today, by randomly arriving at more efficient photosynthesis, or they might have "pulled up the ladder" on a more efficient form of photosynthesis.
Photosynthesis using an alternative form of carbon fixation could have a faster kinetic rate. CO2 assimilation is often limited by the low catalysis rate of Rubisco. One proposed theoretical synthetic form of oxygenic photosynthesis could be two to three times faster than the Calvin–Benson cycle (Bar-Even et al., 2010. Design and analysis of synthetic carbon fixation pathways).
In short Martian photoautotrophs
- Would be likely to absorb red light and use it for photosynthesis, and may use the full range of visible light potentially doubling light to biomass conversion at low light levels compared to terrestrial blue-green algae, similarly to seaweed.
- May have adjustable light antenna size in order to cope with fluctuations of sunlight in the Martian solar storms so permitting high efficiency at high light levels, potentially tripling the efficiency.
- May have more efficient carbon fixation for photosynthesis than the Calvin cycle in terms of the electrons needed or the energy needed per mole of fixed CO 2 which could make it two or three times faster.
Each of these separately could increase biomass yields and it might have several of them combined which could increase the yield ten-fold or more.
A Martian photoautotroph would only need a small improvement in efficiency compared to terrestrial life to be competitive with our photoautotrophs in the oceans, and there seem to be possibilities for major increases in efficiency. This Martian photoautotroph then might replace the natural species in our oceans.
This could be harmless, even beneficial in some situations if it is compatible with terrestrial biology, for instance increase the productivity of oceans, and help with greening deserts. However differences in biology could make it inedible, accidentally toxic, etc., and if it outcompetes terrestrial algae it could make them extinct.
Enhanced Gaia - could Martian life be beneficial to Earth’s biosphere?
So far we’ve focused on situations where biosphere collisions are harmful, since the topic is planetary protection, so we need to focus on scenarios where there is indeed a need to protect Earth. However we should also recognize that the introduction of extraterrestrial life to our biosphere could also be beneficial, as Rummel mentioned in his foreword to “When Biospheres Collide” (Meltzer, 2012, When Biospheres Collide).
We have examples from multicellular life to show that invasive species aren’t always harmful. Schlaepfer et al did a survey of invasive species and in their table 1 they find many non native species that are actually beneficial. Some were deliberately introduced for their value for conservation, but many of the best examples were introduced unintentionally (Schlaepfer et al., 2011. The potential conservation value of non ‐ native species).
Schlaepfer doesn’t list any microbial examples. What could benign interactions with terrestrial life look like for Martian microbes? Here are a few suggestions:
- More efficient photosynthetic life from Mars could increase the rate of sequestration of CO2 in the sea and on land, improve soil organic content, and perhaps help with reduction of CO2 levels in the atmosphere
- More efficient photosynthesis could increase the productivity of oceans and help with greening deserts
- Most of the surface layers of our oceans are deserts, except near to the coasts, because of the limitation of nitrogen, phosphorus, iron and silica (needed for diatom shells) (Bristow et al., 2017. Nutrients that limit growth in the ocean) . If extraterrestrial life has different nutrient requirements, it may be able to inhabit these deserts and form the basis of an expanded food web.
- Martian microbes could be better at nitrogen fixation, phosphorus and iron mobilization, and so improve our soils, and help with crop yields as endophytes and exophytes – the plant equivalent of the human microbiome – these are fungi, bacteria and other microbes that co-exist with plants without harming them and often beneficially (Gouda et al., 2016 . Endophytes: a treasure house of bioactive compounds of medicinal importance).
- Martian life might aid digestion or enter into other beneficial forms of symbiosis with humans
- Martian life could produce beneficial bioactive molecules as part of the human microbiome. These could include molecules that are antiviral, antibacterial, antifungal, insecticides, molecules that kill cancer cells, immunosuppressants, and antioxidants - we get all of those from beneficial microbes that are already in our microbiome. (Borges et al., 2009. Endophytic fungi: natural products, enzymes and biotransformation reactions).
- Just as Martian microbes could enter the human microbiome, they could also enter plant microbiomes as endophytes and those interactions need not be harmful, many could be beneficial. (Afzal et al., 2019. Plant beneficial endophytic bacteria: Mechanisms, diversity, host range and genetic determinants)
- New forms of yeast could be of interest in the food industry (Sarmiento et al., 2015. Cold and hot extremozymes: industrial relevance and current trends) .
- Martian life could increase species richness by gene transfer to Earth microbes, leading to more biodiverse microbial populations.
- Martian extremophiles could colonize microhabitats in deserts and eroded landscapes barely habitable to terrestrial life, helping with reversal of desertification
- More efficient Martian microbes might be useful to generate biofuels from sunlight and water (Schenk et al., 2008. Second generation biofuels: high-efficiency microalgae for biodiesel production)
- Martian life might be accidentally toxic and control harmful microbes or insects
- It could add a new domain of life with almost entirely beneficial interactions similarly to the Archaea
- It could add to biodiversity with new forms of multicellular life based on a different biochemistry, or multicellular life in a different domain of life from the eukaryotes, with a more ancient common ancestor.
Even if introducing martian life is largely beneficial, it could still be harmful in some ecosystems or have mixed effects with some harms and some benefits
However even if introducing terrestrial life is largely beneficial we still need caution. There would be not just one encounter in one ecosystem. Martian conditions may well favour polyextremophiles able to survive in a wide range of conditions.
Chroococcidiopsis is perhaps our best analogue for a Martian cyanobacteria and it is a polyextremophile and found in many habitats throughout the world. Also the microbes would evolve eventually, and perhaps quickly, or change gene expression, and eventually find new habitats that they can colonize.
Maybe some of these encounters would be beneficial in some ecosystems, while other ecosystems are degraded, possibly even by the same interactions with the same microbe. Similarly for organisms, some organisms may be benefited and others harmed.
To take an example, even if what we find on Mars is just a new strain of Chroococcidiopsis, it could have toxins, protoxins or accidentally toxic semiochemicals. As an example of this, microalgae produce accidental hepatotoxins that can damage livers of cattle and dogs that eat algal mats, a common occurrence in the Great Lakes (Hoff et al, 2007. Ontario: Neurotoxic cyanobacterium (blue-green alga) toxicosis in Ontario). Such toxins wouldn’t harm humans, since we don’t eat the algal mats, but are harmful to other creatures. Similarly even something as harmless seeming as a new strain of chroococcidiopsis that developed in the very different conditions of Mars with the cold conditions, UV and ionizing radiation might produce accidental toxins that the terrestrial strains don’t produce.
The same Martian microbe may also have both harmful and beneficial effects on the same organism, or in the same ecosystem or in different ecosystems (as for the dandelion which as we saw is normally beneficial but harmful in Alaska (James River Association, 2021. Native, Non-Native, Invasive - and Dandelions!) ). Generally there might well be a mix of some beneficial and some harmful interactions.
Or the interactions could all be beneficial.
Amongst a million extra-terrestrial civilizations that return an unsterilized sample from a nearby biologically isolated biosphere with limited precautions and technological capabilities to contain it, we don’t know how many would find they have harmed the biosphere of their home world. It might be that
- it is never seriously harmful, it usually leads to an enhanced Gaia, and is almost always a beneficial process, all the way through to:
- most civilizations’ biospheres are seriously degraded.
There is no way to know.
Swansong Gaia hypothesis – that Mars would have far more CO2 without life – photosynthetic life itself keeps Mars barely habitable by growing and taking CO2 out of the atmosphere as Mars gets more habitable
This book introduces the Swansong Gaia Hypothesis in the sections:
and the hypothesis is summarized briefly in:
This book's Swansong Gaia hypothesis is that Mars gets larger CO2 emissions and infall from space than are needed to maintain the CO2 levels in the atmosphere in the past and present. It is expands on the idea of an abiotic pump based on carbonate formation(Nolan, 2008. Mars: A cosmic stepping stone. In MARS A Cosmic Stepping Stone : 137) and abiotic photosynthesis (Kahn, 1985. The evolution of CO2 on Mars) (Haberle et al., 2001. On the possibility of liquid water on present ‐ day Mars) which acts to remove carbon dioxide from the atmosphere whenever the combination of temperature and pressure goes significantly above the triple point of water. It expands this to a hypothesis that it was life itself that made Mars almost uninhabitable.
The main evidence for this hypothesis so far is the observation that the Martian atmosphere is remarkably close to the triple point of water. Part of this is due to equilibrium of the atmosphere with the vapor pressure of dry ice at the poles. This prevents any stable atmosphere between around 6 mbar and a little over 30 mbar in the current state of the Martian atmosphere with the carbon dioxide rapidly condensing out as dry ice at the poles.
However at times of higher axial tilt the dry ice sublimes completely and raises the atmospheric pressure to 12.5 millibars . If this is due to a biological pump then presumably the biological pump weakens to the point where it has minimal effect below 12.5 millibars due to the small amounts of liquid water available. For details see:
By this hypothesis, without life Mars might have continued with lakes, rivers, seas even through to the present. There are several bars of carbon dioxide locked up in the carbonates. But over a very wide range of emission scenarios for Martian volcanoes, life is able to remove nearly all the CO2 from the atmosphere to keep Mars just short of uninhabitable. This pump stops short of reducing e the levels all the way through to uninhabitability because the biological pump gets weaker as the planet gets colder because a colder planet has less liquid water for life to inhabit.
Text on Graphics: Swansong Gaia hypothesis.
Modern Mars looks sterile, but photosynthetic life might take CO2 out of the atmosphere when it gets warm enough for liquid water, keeping Mars barely habitable.
This would work with a wide range of CO2 emission scenariosEarly Mars with water and possibly early life
Image credits: NASA’s Goddard Space Flight Center from: (Steigerwald, 2019, New Insight into How Much Atmosphere Mars Lost )
As we saw, biotic photosynthesis would work similarly to abiotic photosynthesis, but would be a stronger feedback, and act faster to restore the atmosphere to its barely habitable state after a pulse of volcanic activity or impact of a large comet. It could also keep the surface barely habitable over a wider range of CO2 supply levels to the atmosphere.
Here photosynthesis would remove the CO2 mainly by carbonate formation. It would also produce organics which may be sequestered but it would also produce oxygen which might be used to return some or all of the organics to the atmosphere as carbon dioxide. If Mars has extensive lakes during the CO2 pulses, as it seems to have had sometimes in the past, from the Gale crater evidence, then there might be significant loss of carbon dioxide to buried organics. Clays would trap the organics in the sediments.
This is how it would work:
- Initially Mars has a thick atmosphere with a sea, rivers, surface water and warm conditions to encourage photosynthetic life. This either evolves on Mars, or is seeded from Earth
- Biotic and abiotic photosynthesis removes carbon dioxide from its atmosphere. Cyanobacteria precipitate carbonates and so speed up the abiotic process of carbon sequestration through direct formation of carbonates through extracellular and intracellular cyanobacterial calcification (Benzerara et al., 2014. Intracellular Ca-carbonate biomineralization is widespread in cyanobacteria). The carbonates, together with clays and other sediments would also trap organics from life which is another way to take CO2 out of the atmosphere. Without plate tectonics, Mars can’t return this to the atmosphere again
- Martian life might also have photosynthetic life that makes use of the calcite as shells simlarly to some diatoms and the coccolithophores (single cell shell forming marine organisms which formed deposits such as the White Cliffs of Dover).
- The sequestered carbon dioxide thins the Mars atmosphere reducing the greenhouse effect.
- This makes Mars less habitable for photosynthetic life.
This feedback loop continues until the CO2 removed is in equilibrium with the CO2 supplied to the atmosphere. At this point, Mars is so cold and dry that its photosynthetic life has minimal effect on its atmosphere.
As Mars warms up slightly due to a new pulse of CO2 introduced to a planet with minimal life on it:
- Life starts to spread and generates hydrogen and methane which adds to habitability by enhancing the CO2 greenhouse effect
- In the more habitable Mars life briefly becomes abundant and starts to take CO2 out of the atmosphere
- After a delay life makes Mars gradually uninhabitable again.
Mars does have continuous introduction of some CO2 into the atmosphere from volcanoes on a geological timescale, and it has other abiogenic sources of carbon dioxide, for instance from comets, so it would not lose all the CO2. Once the amount of life present is very low, CO2 builds up again. This would lead to an equilibrium, a homeostasis, where there is just enough life to remove nearly all the CO2 from the atmosphere as it is introduced by comets, volcanic eruptions, etc.
Mars is still geologically active with numerous young fissure fed flood lavas on the Elysium Planitia from 500 to 2.5 million years ago. In a preprint, Horvath et al suggest evidence for a possibly volcanic feature with a possible age range of only 53 to 210 thousand years. They suggest that the Cerberus Fossae region may still be active today (Horvath et al, 2020. Evidence for geologically recent explosive volcanism in Elysium Planitia).
In addition, measurements of carbonates in young Martian meteorites suggests carbonate formation is ongoing on Mars, with evidence of carbonate formation at 3.9 billion years ago (ALH84001), ~600 million years ago (Nakhla) and < 200 million years ago (EETA 79001) (Niles et al., 2010. Stable isotope measurements of Martian atmospheric CO ₂ at the Phoenix landing site). These meteorites are from rocks from at least 3 meters below the surface and probably 50 meters below the surface, as we saw above in the section NASA's central finding that any harmful life can get here better protected in meteorites is rebutted by BOTH of its main cites for it
There has to be a continuing input of CO2 into the system to form these carbonates.
There’s one possible weak biosignature in the meteorites. Life preferentially uses the lighter stable isotope of carbon, carbon 12 because it leads to lower energy costs than the heavier stable isotope carbon 13 (USGS, n.d., Resources on Isotopes), so life processes tend to separate out the two isotopes. The carbon 13 ratios (ratios) in the martian meteorites are highly variable, from -20% to +42%. Ratios on Earth fall into a similar wide range from -25% to +30%. Jull et al suggest fractionation by life as one of hypothetical explanation (Jull et al., 1995. Isotopic composition of carbonates in the SNC meteorites Allan Hills 84001 and Nakhla. Meteoritic's : 1667).
It’s a weak biosignature as the researchers mention another possible explanation, that the atmosphere is the source for the high carbon 13 ratio and the low carbon ratio could be due to magma that is low in carbon 13 (Jull et al., 1995. Isotopic composition of carbonates in the SNC meteorites Allan Hills 84001 and Nakhla. Meteoritics : 1667). This is a plausible explanation as the current ratio is 46%±4% as measured by Curiosity (Webster et al., 2013. Isotope ratios of H, C, and O in CO ₂ and H2O of the Martian atmosphere). The early Martian atmosphere may have had a composition of 10% to 20% (Shaheen et al., 2015. Carbonate formation events in ALH 84001 trace the evolution of the Martian atmosphere).
If there is life on Mars it would be likely to cause biogenic fractionation, but the potential for evolution of the ratios in the atmosphere and other geological sources of variation will make it hard to disentangle any biogenic signature component in variations in the carbon isotope ratios.
If this biological feedback loop is present, we would predict the current situation on Mars to have enough CO2 to be barely habitable, but not to have lost so much as to have no life at all. It would occur in such trace amounts that its effect on the atmosphere is only barely detectable, if at all.
The result is that Mars would look pretty much the same as Mars does to us now, with life not easily detectable from orbit. There is enough oxygen in the atmosphere to mask any seasonal fluctuations in oxygen produced by life in marginal habitats.
When there is enough CO2 for open water on Mars, the strength of this feedback effect could be amplified further by organisms similar to terrestrial forams in the early lakes and oceans, or other similar organisms.
On Earth forams evolved calcareous tests (shells) less than half a billion years ago during the Cambrian explosion (Boudaugher-Fadel et al., 2018. Evolution and geological significance of larger benthic foraminifera). However the Martian water was oxygen rich long before we have evidence of abundant oxygen on Earth.
So, it’s possible Mars evolved microorganisms with carbonate shells at an earlier stage of evolution. Planktic foraminifera produce as much as half the terrestrial particular carbonate flux to the ocean floor at a rate of around 2.9 gigatons per year (Jacob et al., 2017. Planktic foraminifera form their shells via metastable carbonate phases). So this process might cause a similar increase in the carbonate flux on Mars and be a significant increase over the abiotic processes.
Forams incorporate oxygen dissolved in the water to make their shells, rather than from the atmosphere. However, Martian lakes and seas could have oxygen rich surface layers, similarly to Gale crater lake (Hurowitz et al., 2017. Redox stratification of an ancient lake in Gale crater,) (Doyle, 2017, Ancient Lake On Mars Was Hospitable Enough To Support Life) (Lanza et al., 2014. High manganese concentrations in rocks at Gale crater, Mars) (NASA, 2017, A guide to Gale crater) (video) .
Terrestrial forams can be single cell secondary consumers, or kleptoplasts, ingesting chloroplasts from green algae to photosynthesize (Serôdio et al., 2014. Photophysiology of kleptoplasts : photosynthetic use of light by chloroplasts living in animal cells). The Martian analogues of forams could be either of those, or they might be themselves photosynthetic.
Then as we saw it could also involve coccolithophores.
The feedback process involving forams or similar creatures would work like this:
- Some of the dissolved carbon dioxide in the water gets incorporated in the shells of forams accelerating sequestration of carbonates in lakes and oceans
- This cools down the planet and makes the lakes and oceans less habitable until they freeze over and the feedback stops.
- At times when the Mars orbit has high eccentricity some of the ice would still melt when it is closest to the sun. This would lead to a situation where the lakes are ice covered for most of the year but have liquid water for a short while in summer, with the duration of ice free conditions based on an equilibrium between sequestration by the forams with carbonate shells, and the volcanic emissions of CO2.
Once photosynthetic life is no longer active in large numbers, and the forams no longer form shells, in large numbers, Mars is free to warm up, and its atmosphere thicken, due to changing eccentricity, or a period of increased volcanic emissions of greenhouse gases, but as it does so, the spread of life across the planet then takes more carbon dioxide from its atmosphere and cools it down again.
The reason there is any CO2 left may be because of the continuing low levels of volcanic emissions on timescales of millions of years. Another source of CO2 would be the infall of organics from comets and meteorites (Frantseva et al., 2018. Delivery of organics to Mars through asteroid and comet impacts) (Goetz et al., 2016. MOMA: the challenge to search for organics and biosignatures on Mars. International Journal of Astrobiology :247), These would combine with superoxygenated surface layers, and ionizing radiation that dissociates surface organics back to gases such as methane and carbon dioxide and water vapour. Some CO2 would also be delivered in comets.
In this way, this “Swansong Gaia” feedback loop continually keeps Mars only marginally habitable. Whenever it warms up and gets a bit more habitable this "Swansong Gaia" feedback loop would kick in making it less habitable again.
So far we have a simple model where there is only photosynthetic life removing CO2 from the atmosphere which cools it down reducing the amount of water available until the amount of life is minimal but not completely eliminated, just enough to stay in balance with the input of CO2 from volcanoes, and other sources like comets.
However if Mars has a biosphere it's likely to be much more complex than such a simple model would suggest. For one thing, all life depends on nitrogen, so, where does the photosynthetic life get its nitrogen from? Then there would also be interactions involving methane. Then there would be other interactions for instance involving carbon monoxide and hydrogen gas.
So let's look at the more complex possibilities here. Some of these interactions seem likely to strongly reinforce the Swansong Gaia tending to keep at a relatively stable minimally habitable state. Others could make it vary, sometimes weaker sometimes stronger.
There is no attempt here to do detailed modeling. It is just a sketch of how some of the cycles could work in a Swansong Gaia biosphere to help explain the observation of a Martian atmosphere close to the triple point of water. We also look at some of the methane spikes and the apparently seasonal oxygen cycle.
How the biological nitrogen cycle can reinforce Swansong Gaia if Martian microbes fix and release nitrogen similarly to microbes in terrestrial deserts
So far the Swansong Gaia hypothesis is based on the carbon cycle on Mars. But Mars would have a nitrogen cycle too, and nitrogen is essential for life. So how would its nitrogen cycle interact with the Swansong Gaia carbon cycle? We look at different scenarios depending on whether Martian life has nitrogen fixation, and denitrification which returns nitrogen to the atmosphere.
For this to work through to the present, Martian life needs to be able to do nitrogen fixation at low nitrogen levels.
If nitrogen fixation is impossible with low levels of nitrogen, the cycles described here will only apply to early Mars when there was more nitrogen in the atmosphere.
However as we saw there does seem to be a marginal possibility for nitrogen fixation in the present day Martian atmosphere see:
Nitrogen fixation scenario 1: Martian life never developed nitrogen fixation – weaker Swansong Gaia effect
In this scenario, life is limited to habitats and microhabitats with nitrate deposits or abiotic nitrogen. For this scenario see Possibility that past life in Jezero crater or even modern life never developed nitrogen fixation – or if it did, that nitrogen fixation was never taken up by microbes in oxygen rich surface layers
In this scenario, the Swansong Gaia effect that we looked at in the previous section would still exist but be weaker. As the planet warms there would be large amounts of water but most of this water would be inaccessible to life.
Photosynthetic life would still spread across the oceans and lakes, but it would be limited by the available nitrates, so it would take less CO2 from the atmosphere than if it was capable of nitrogen fixation
There would still be a Swansong Gaia feedback. Life would be more abundant when the planet warms in habitats with sufficient access to nitrates, but it would be a weaker feedback than if life has nitrogen fixation and photosynthetic life can spread throughout any wet habitats throughout the planet
Nitrogen fixation scenario 2: Martian life has nitrogen fixation and also denitrification to return nitrogen to the atmosphere, similarly to life on Earth – strong Swansong Gaia effect
If life on Mars does have nitrogen fixation, what happens will depend on whether Martian life is also capable of denitrification, biological pathways that can return the nitrogen from nitrates and ammonium back to the atmosphere.
Nitrogen in Earth's atmosphere is maintained at its high levels by denitrification. Capone et al. say that on a planet with oceans and continents, since nitrates are so readily soluble in water, without denitrification, nitrogen on the land would be substantially depleted, nitrogen would end up in the ocean and terrestrial life would be impossible (Capone wt al., 2006. Follow the nitrogen).
If life on Mars is as on Earth and returns nitrogen to the atmosphere through denitrification, then during a warming spell after a pulse of CO2, life would use denitrification of the nitrate deposits to produce the nitrogen needed for photosynthetic life to spread through the planet and to continue to remove the CO2 from the atmosphere for as long as it is produced.
In this scenario, as life spreads, responding to the CO2 pulse, the CO2 levels go down. As this happens then the deserts become colder and drier, in the thinner atmosphere, and as in terrestrial deserts, the colder and drier conditions lead to life returning less nitrogen to the atmosphere.
In this scenario over most of Mars it’s nitrogen rather than water that limits life, So in this scenario, it’s not impossible that Mars still has widespread liquid water, which as we saw is possible according to some ideas, for instance in the polar regions there might be large volumes of ice melt in cryoconite holes or the subglacial melt. .
Perhaps also below the surface in caves heated by geothermal processes. For a list of some of the potential habitats we might find on present day Mars see above::
- Potential for ultra low concentrations of life from microbial oases in Jezero crater itself
- Potential for distant microbial oases as sources of ultra low concentrations of viable spores in dust storms
- Potential for distant Mars habitat common in Antarctica: Fresh water drilled by sun warmed dust and prevented from evaporation by an ice lid
- Ice boulders thrown onto the surface in 2021 from a formerly habitable site on the flanks of Olympus Mons
In this scenario, there could be nitrogen fixing photosynthetic life on Mars in low concentrations throughout the planet. These habitats wouldn't be uninhabited, but have very low concentrations of life because of the limitations set by the low nitrogen levels in the atmosphere.
This is a scenario with many potential habitats but with life at low concentrations and hard to spot except in habitats with high levels of nitrogen already fixed, for instance as nitrates or organics from life.
Nitrogen fixation scenario 3: Martian life has nitrogen fixation but no denitrification –Swansong Gaia effect varies in effect depending on deliveries of nitrogen by comets
Now let’s look at another scenario, where Mars developed nitrogen fixation but not denitrification.
In this scenario, there is no way for life to return nitrogen to the atmosphere. Nitrogen builds up at times after large comet impacts or multiple comet impacts which deliver nitrogen to the planet.
If enough nitrogen has built up, then after a warming pulse of CO2, photosynthetic life spreads through the planet but it quickly uses up all of the easily accessible nitrogen in the atmosphere. This gets cycled through the biosphere for a while but eventually is removed from the biosphere as buried nitrates and organics.
In this scenario, after an increase in CO2 emissions by volcanoes, CO2 levels might rise first, then fall due to photosynthetic life, but rise again as CO2 continues to get added to the atmosphere and life can no longer remove it because it is limited by the reduced amount of nitrogen in the atmosphere.
During times of less CO2 emissions, life wouldn't use much nitrogen so the nitrogen could build up and be ready for life to use at the start of the next CO2 pulse.
In this scenario, the Swansong Gaia feedbacks vary. When there is a lot of nitrogen in the atmosphere, the feedbacks are stronger, and the climate stays close to the Swansong Gaia state and quickly returns to it. Then when there is a shortage of nitrogen the feedbacks are weaker and the climate is more variable.
In a closely related scenario, Mars has nitrogen fixation but levels of nitrogen fixation always use more nitrogen than is produced by denitrification and other sources such as comets.
Nitrogen fixation scenario 4: Martian life behaves like the life in terrestrial hyperarid deserts – nitrogen fixation and denitrification but denitrification stops in the driest conditions - strongest Swansong Gaia effect
Finally, if Mars life behaves like life in Mars analogue deserts a more complex picture of denitrification / nitrogen fixation arises (Shen et al., 2021. Nitrogen Cycling and Biosignatures in a Hyperarid Mars Analog Environment) which we’ll see actually reinforces the Swansong Gaia effect.
In the driest conditions in terrestrial Mars analogue deserts, the research of Shen et al suggests there is no biotic nitrogen fixation and no denitrification, just nitrate assimilation with the nitrates fixed abiotically from the atmosphere. They deduce this based on the isotope ratios for nitrogen and oxygen.
In wetter sites they detected more complex pathways and denitrification dominates. They found that some denitrification does occur in some hyperarid sites .
They suggest
“These results suggest that N cycling on the more recent dry Mars might be dominated by nitrate assimilation that cycles atmospheric nitrate and exchanges water O during intermittent wetting, resulting stable isotope biosignatures could shift away from martian atmospheric nitrate end member.
“Early wetter Mars could nurture putative life that metabolized nitrate with traceable paleoenvironmental isotopic markers similar to microbial denitrification and nitrification stored in deep subsurface.”
(Shen et al., 2021 . Nitrogen Cycling and Biosignatures in a Hyperarid Mars Analog Environment).
This suggests a scenario where as Mars becomes more habitable after a warming pulse, the wetter conditions in deserts first encourage microbial denitrification of any nitrate deposits, which by increasing the levels of nitrogen makes the biosphere more habitable for nitrogen fixing photosynthetic life, which would strengthen the Swansong Gaia effect, with the CO2 removed more rapidly the thicker the atmosphere.
As the CO2 is removed and the planet becomes drier, the balance between denitrification and nitrification shifts in the other direction. Over much of Mars, where nitrates are less available, life is limited by the nitrogen fixation before it reaches the point where it is limited by the availability of liquid water. So there would be very little or no biotic nitrogen fixation and most of the nitrogen for life comes from the remaining nitrates. However denitrification continues.
As CO2 levels get lower, the martian surface gets so dry that denitrification also stops.
However we still have the slow process of abiotic nitrogen fixation. Assuming no extra CO2 is added, to warm up the planet, in those hyperarid conditions without biotic nitrogen fixation or denitrification, nitrogen levels in the atmosphere continue to fall until it's too low for significant abiotic nitrogen fixation, and photosynthetic life is reduced still further.
At some point along this process, the amount of photosynthetic life is reduced so much that it can no longer remove significant CO2. At that point CO2 from volcanoes builds up again until denitrification produces enough nitrogen for photosynthetic life to flourish enough to take the CO2 out of the atmosphere.
This then could have different equilibrium points depending on the input of CO2 .
- If the CO2 input is high enough this would keep the planet at a warming level high enough to keep nitrogen fixation and denitrification in balance, and at a level where some nitrogen fixating photosynthetic life is possible throughout the planet.
- If the CO2 emissions from volcanoes are very low it may go down to a warming level with only denitrification, nitrogen fixation stopped almost completely and most life restricted to regions with already available nitrates.
- With even lower levels of CO2 from volcanoes there would be only a small amount even of denitrification in Swansong Gaia.
The first of these three scenarios leads to the nitrogen balance with the most habitable Swansong Mars of the three scenarios.
In this scenario, Mars is likely to have enough water availability to be more like the wetter parts of terrestrial Mars analogue hyper arid deserts where denitrification begins to dominate over nitrification. Perhaps biofilms in the brines found by Curiosity could be wet enough for denitrification to keep the nitrogen levels in the atmosphere from falling too low, or it might be due to life in the deep subsurface, or in the layers of fresh water in the polar ice that form due to the solid state greenhouse effect, or the flow like features, or in Martian caves or the lakes below glaciers. Antarctica has a rich diversity of cold adapted denitrifying bacteria (Cabezas et al., 2022 . Ubiquity and Diversity of Cold Adapted Denitrifying Bacteria Isolated From Diverse Antarctic Ecosystems).
. See:
There might be another way to achieve this balance. The nitrogen could be delivered by comets, possibly in large quantities in the form of ammonium salts, and the same balance would be reached of just enough nitrogen for some nitrogen fixation throughout the planet but instead of denitrification supplying the input of nitrogen, it’s the nitrogen delivered from comets that helps to sustain the levels of nitrogen needed to balance the nitrogen lost through nitrogen fixation (Poch et al., 2020. Ammonium salts are a reservoir of nitrogen on a cometary nucleus and possibly on some asteroid).
There could be other more complex scenarios than these with sometimes denitrification dominating and sometimes nitrogen fixation dominating, sometimes Swansong Gaia reinforced and sometimes weakened.
Methanogen based swansong Gaia in early Mars
First lets summarize again the situation for early Mars before moving on to look at how the methane cycles might work today. As we saw above in: Gaia, Swansong Gaia, or Anti-Gaia? How Mars's thin atmosphere near the triple point of water could be a weak biosignature of a Swansong Gaia stabilized through photosynthesis to keep Mars just barely habitable,. Sauterney suggests a hydrogen / methane cycle leading to a strong form of the anti-Gaia hypothesis for Mars. However from this new viewpoint of Swansong Gaia, their results look like a mechanism that could help form a self perpetuating Swansong Gaia.
Methanogens cool down the planet because methane is a weaker greenhouse gas than hydrogen because of the "collision-induced absorption effect where molecules of carbon dioxide and hydrogen interact with each other," (Pultarova, 2022, Ancient Mars microbes triggered climate change that made it hard for them to survive).
So on Mars microbes that convert hydrogen to methane (methanogens) are cooling. Sauterney's team simulated a Martian atmosphere with between 0.3% and 10% hydrogen and atmospheric pressure 0.5 to 3 atmospheres (Sauterney, 2022, Early Mars habitability and global cooling by H2-based methanogens : Supplementary Table 1).
This is the pathway they looked at with the hydrogen produced from volcanoes, with photolysis (reactions involving sunlight) converting some of it to / from methane and carbon monoxide and then the methanogens (MG) converting the hydrogen and carbon dioxide into biomass and methane. There's a side reaction with the carbon monoxide in the atmosphere converted to acetate by CO based acetogens, and then to methane plus biomass by methanogenic acetotrophs (microbes that consume acetate and convert it to methane).
They developed this model originally for early Earth where it helps make Earth warmer because methane is a warming gas in their simulated early terrestrial atmosphere of nitrogen at 1 bar, i.e. the same as modern atmospheric pressure). The methane warms the atmosphere slightly by 4°C (Sauterney, 2021, Co-evolution of primitive methane-cycling ecosystems and early Earth’s atmosphere and climate : Figure 4e).
H2-based methanogens (MG),
CO-based acetogens (AG)
methanogenic acetotrophs (AT)
from: (Sauterney, 2021, Co-evolution of primitive methane-cycling ecosystems and early Earth’s atmosphere and climate : Figure 1)
However on Mars with an atmosphere consisting of carbon dioxide and hydrogen only, their model biosphere, methanogens quickly take the hydrogen out of the atmosphere, rapidly cooling early Mars by 33 to 45°C until ice forms over the surface.
While hydrogen methanogens may have contributed to maintaining temperate conditions on Earth, they would have cooled the early Martian surface, with a reduction of the maximum possible temperature by 33 to 45 K
(Sauterney, 2022, Early Mars habitability and global cooling by H2-based methanogens : 6)
This could have made Mars almost uninhabitable early on, However some life would persist
At life- atmosphere steady state, the relatively high brines freezing point and relatively weak biological feedback balance out with a large ice coverage yet relatively warm ice-free surface, resulting in methanogenic life being limited to small ice-free regions but at or very close to the surface. With brines freezing at 252 K, Hellas and Isidis Planitiae and Jezero Crater are among the few regions that may remain free of ice, where the median minimum depth of Noachian methanogens would then be within the top few meters
(Sauterey, 2022, Early Mars habitability and global cooling by H2-based methanogens : 6)
As we saw before, this new idea of a Swansong Gaia, it could be an extra mechanism leading to an early forming barely habitable Swansong Gaia. As volcanoes add hydrogen to the atmosphere, the methanotrophs remove it and convert it to methane, cooling the atmosphere until it is barely habitable. So long as there is a continuing input of hydrogen the atmosphere never cools enough to be completely uninhabitable.
This simple picture for early Mars assumes there are no methanotrophs. Sauterey et al say that later on in the history of early Mars, biological consumption of methane (methanotrophs) could have lead to warming episodes. He suggests this as a possible explanation of the transient warming episodes that seemed to have happened in early Mars.
On Earth, the evolution of methanotrophy (biological consumption of methane) could have transiently offset the warming effect of methanogenesis; in contrast, methanotrophy could have driven a warming event on the Late Noachian/Hesperian Mars. The co-evolution of Martian surface conditions with a diversifying methane cycling biosphere therefore adds to mechanisms possibly explaining an early global cooling event as well as transient warming episodes that seem to have punctuated the early Martian climatic history
(Sauterey, 2022, Early Mars habitability and global cooling by H2-based methanogens : 6)
They explain in the earlier paper that this is sulfate based methanotrophy. If this evolves early the methanotrophs use the sulfuric acid as it is produced and come into an equilibrium with the methanogens, so that this just slightly reduces the warming of early Earth, if it evolves late then it can lead to a sudden cooling and glaciation of early Earth as the methanotrophs rapidly use up stocks of sulfuric acid from the volcanoes to remove all the methane from the atmosphere and convert it to hydrogen.
Our evolutionary sequences culminate with the evolution of anaerobic methanotrophy, which uses the oxidation of CH4 as primary source of energy, based on the consumption of H2SO4, the main oxidative species of the globally reduced early Earth
...
Slow evolution may delay methanotrophy after the response of the carbon cycle to methanogenesis. In this case, the oceanic stocks of H2SO4 are sufficient for methanotrophs to rapidly consume almost all of the atmospheric CH4 (Supplementary Fig. 6) and the planet is temporarily characterized by an atmospheric deficit in both CO2 and CH4. As a result, temperature plummets below the initial abiotic temperature.
In contrast, if evolution is fast enough and methanotrophs evolve before equilibration of the methanogenic biosphere with the planetary atmosphere and climate, atmospheric CH4 may be consumed during or after the warming period (Fig. 3) but before the deficit in pCO2 builds up; temperature then returns close to its initial value
(Sauterney, 2021, Co-evolution of primitive methane-cycling ecosystems and early Earth’s atmosphere and climate : Figure 4e)
A similar process on Mars however would have a warming effect
[Need to check details with the author. I'm not sure how this works, do the methanotrophs produce hydrogen on Mars? I can't currently find details for methanotrophs that produce hydrogen in anoxic conditions]
However the warming would be short lived as the methanogens would soon take the hydrogen out of the atmosphere again.
With modern Mars there is almost no hydrogen or methane in the atmosphere but may be large stocks of buried methane clathrates. Also there are possibly large quantities of buried organics and organic infall from space. Then there could be some hydrogen produced below the surface that methanogens could use.
So methane on modern Mars would be produced from hydrogen or from organics, or it could just be liberated from buried clathrates.
There are many reactions that can produce methane from organics. In this table the reaction Sauterey et al looked at is hydrogenotrophic methanogenesis, in the second row which converts hydrogen and carbon dioxide (dissolved in water as carbolic acid) to methane and water:
Table I From: (Kharitonov et al., 2021. Microbial Communities in Methane Cycle: Modern Molecular Methods Gain Insights into Their Global Ecology)
So we need to look for different cycles in present day Mars.
Warming from methanogens limited by Swansong Gaia feedback from photosynthesis which produces oxygen which turns much of the methane to CO2 and also fixes the CO2
We may also be able to explain trace levels of methane too (Yung et al, 2018) (Klusman et al, 2022), with another parallel methane cycle.
After an initial pulse of CO2, perhaps from a cometary impact or volcanic eruptions, methanogens produce more methane. This warms the planet, leads to more of the photosynthetic life and so leads to CO2 removed which acts to cool the planet to counteract the warming of the methane.
In addition photosynthesis would lead to high levels of oxygen in surface water and in the atmosphere, which would turn much of the methane to CO2 reducing its warming impact.
Methanogens could potentially make the planet more habitable if they were the only lifeform there. However, they would never be able to build up to large numbers in a sustained way, because whenever they warm the planet they stimulate so much photosynthetic life that they automatically, though indirectly, self limit themselves.
However, the methanogens might be able to spread rapidly in some initial pulse, for instance if most of the liquid water is below the ground at that point, out of reach of sunlight for photosynthesis.
That could give a period of warmer conditions and for lakes to form before the photosynthetic life spreads through the newly warmed planet.
The Swansong Gaia could be a result of a mix of methanogens that warm the planet and photosynthetic life that cools it. The methane is too reactive to remain long in such an oxygen rich biosphere, and so is unable to significantly counteract the effect of the removal of CO2 by the photosynthetic life forms.
Methanogens in the deep subsurface could be self limiting in another way, which could limit them even in absence of photosynthesis.
Self limiting microbe colonies can convert underground aquifers to calcite to maintain a subsurface barely habitable Swansong Gaia hydrology
As with the terrestrial Gaia there may be not just one cycle, but several interlocking and reinforcing cycles. There are many biological pathways that bacteria can use to form calcite (cement) for instance, with some of them used in self healing concrete (Rummel et al., 2017, Four Fallacies and an Oversight: Searching for Martian Life) (Dhami et al., 2013. Biomineralization of calcium carbonates and their engineered applications: a review) One such method may be of special interest to Mars. A consortium of methane oxidising and sulfate reducing bacteria can convert underground aquifers to calcite through anaerobic oxidation of methane (Rummel et al., 2017, Four Fallacies and an Oversight: Searching for Martian Life) (Drake et al., 2015. Extreme 13 C depletion of carbonates formed during oxidation of biogenic methane in fractured granite). Perhaps these conditions may occur in the Martian subsurface, for instance at the sources of the methane plumes if these originate in geothermally heated underground aquifers.
The methanotrophs might grow in a layer above the methanogens to catch the methane. Since they form calcite, this could block it off and prevent it reaching the surface. From time to time some of it would break through and this could explain the methane spikes.
In this case the methanotrophs render their subsurface habitat less habitable by converting some of the water to calcite and blocking off their own supply of methane. If this happens near the surface, the warmer conditions may act as a feedback to block off the methane more and so reduce the warming effect on the atmosphere.
This cycle could be combined with the photosynthesis to help reinforce Swansong Gaia.
Perhaps we can use emissions of methane from lake sediments in thermokarst lakes as a rough analogue. This is similarly episodic.
Methane-producing microbes survive on the nutrient-rich soils that comprise the lakebeds in many Arctic and subarctic regions. The microbes release methane at the bottom of the lake, and the gas then rises to the surface and escapes to the atmosphere. The amount of gas that reaches the surface is highly variable over time and at different locations.
(Orwig, 2014, Subarctic lakes belch more methane on brighter days, Earth magazine)
For our closest analogue perhaps we can use the thermokarst lakes in the high Tibetan plateau as an analogue for bubbling methane in a low pressure atmosphere .
The lakes with high emission fluxes were associated with high levels of methanogenic genes in the lake sediments. These microbes produce methane in situ which builds up and then emits. . Unlike many lakes where the methane comes from old deposits of organics that thawed at the end of the ice ages, these methane emissions come mainly from recently formed organics, perhaps from plants and the soil around the lakes. The lakes are small with a large perimeter to surface area ratio. 46% of the lakes emitted methane that formed after 1950 (Yang et al., 2023. Characteristics of methane emissions from alpine thermokarst lakes on the Tibetan Plateau).
Here is some of their data.
Each dot here is an emission spike for the methane, with the light green for emissions of gas bubbling up directly to the atmosphere and the dark green for diffusion through the water.
The emissions were measured for 120 lakes grouped in groups of 4 and measured every
(Yang et al., 2023. Characteristics of methane emissions from alpine thermokarst lakes on the Tibetan Plateau: Figure 4 from supplementary data)
The authors suggest Tibetan lakes are prone to bubbling rather diffusion because of the low atmospheric pressure of the Tibetan plateau at 60% of sea level pressure. They give three reasons for this:
- The lower atmospheric pressure makes methane less soluble in the water which makes it less favorable for diffusion through the water.
- The bubbles of methane form in vertical tubes in the lake floor and the lower air pressure will encourage those bubbles to expand vertically and so to escape more easily.
- The shallowness of the lakes leading to lower hydrostatic pressure.
This would seem likely to favour bubbling on Mars too.
Another cycle might involve methane or alkanes oxidised to siderite (iron carbonate). This is produced in prodigious amounts by some Fe(III)-reducing bacteria (Onstott at al., 2019. Paleo-rock-hosted life on Earth and the search on Mars: a review and strategy for exploration), which is a proposed metabolism for subsurface life on Mars (Parnell et al., 2016. Metalliferous biosignatures for deep subsurface microbial activity) (Onstott at al., 2019. Paleo-rock-hosted life on Earth and the search on Mars: a review and strategy for exploration), these could also form carbonates with other metals such as copper (Onstott at al., 2019. Paleo-rock-hosted life on Earth and the search on Mars: a review and strategy for exploration). As the methanogens warm the planet, more water would be available in subsurface layers suitable for Fe (III) reduction and the formation of siderite would help to cool it down again.
This is not meant to be an exhaustive list of cycles that could help perpetuate a Swansong Gaia. The idea here is to demonstrate the basic principle of a Swansong Gaia by suggesting various ways that life could maintain a planet in a barely habitable state for billions of years. Mars may also surprise us with something unexpected that has the same end effect of keeping it only barely habitable in a Swansong Gaia.
Could seasonal oxygen excess in spring and summer and deficit in winter be a possible signal of photosynthesis maintaining a Swansong Gaia homeostasis on Mars?
Could the seasonal oxygen observed by Curiosity be the result of low levels of oxygenic photosynthesis, the main process driving this hypothetical swansong Gaia?
The oxygen levels rose in spring and summer to levels 30% above those explainable by chemistry alone and dropped back to normal levels in the fall. Methane similarly rises by more than 60% of expected levels in summer, and also spikes randomly and unpredictably (Shekhtman, 2019, With Mars methane mystery unsolved, Curiosity serves scientists a new one: Oxygen). The authors write
Though Mars has the potential to generate significant O2 release due to abundances of oxidants in/at its surface, the mechanisms by which O2 could be quickly generated and then quickly destroyed are completely unknown. As with all surprising results, we hope that continued in situ, experimental, and theoretical results may shed light on this intriguing observation.
(Trainer et al., 2019. Seasonal variations in atmospheric composition as measured in Gale Crater : 3021)
The regions highlighted in yellow show the excess of oxygen in spring to summer and deficit in winter over the expected seasonal variation as measured by Curiosity.
Credits: Melissa Trainer/Dan Gallagher/NASA Goddard (Shekhtman, 2019, With Mars methane mystery unsolved, Curiosity serves scientists a new one: Oxygen)
There is a weak correlation suggesting less oxygen is generated when there is more dust in the air. This is something one would expect from photosynthesis.
Weak inverse correlation of oxygen to argon ratio with dust optical depth, less oxygen is produced when the atmosphere lets less light through. (Trainer et al., 2019. Seasonal variations in atmospheric composition as measured in Gale Crater : Supporting information : Figure 59).
Optical depth of 0.3 means 74% of the light is let through. Optical depth of 1.1 means 33% of the light is let through.
Also the dust didn’t correlate with other processes that could be relevant, the seasonal and interannual pressure variation, or temperature variation.
If photosynthesic life produced the oxygen, it increased it by around 10²⁰ molecules cm² if the atmospheric oxygen came from the surface directly below the atmosphere (Trainer et al., 2019. Seasonal variations in atmospheric composition as measured in Gale Crater : 3017) which works out at about 0.00016 moles of oxygen per square cm (using Avocados' number) or about 26.6 grams of oxygen per square meter.
Cockell studied a similar situation on an Earth analogue exoplanet with photosynthetic life growing inside the rocks, and estimated an output of 2.2 g O2/m²/year for terrestrial cryptoendoliths (Cockell et al., 2009. Cryptic photosynthesis—extrasolar planetary oxygen without a surface biological signature), or less than a tenth of the 26.6 g O2/m²/year found by Curiosity. Mars is not quite an Earth analogue, it has half the solar flux of Earth because it is further from the sun, however it has less of the light blocked out by the atmosphere which compensates enough to make photosynthesis on Mars roughly comparable.
However life on Mars could be more productive than this. In the same paper (Cockell et al., 2009. Cryptic photosynthesis—extrasolar planetary oxygen without a surface biological signature : table 1), the figure for mats living beneath soil surfaces in Yellowstone National Park is 55 g O2/m²/y. This figure is for a small patch of acidic gravel near to one of the Yellowstone geysers. This habitat was suggested as a model ecosystem for Mars in a 1995 paper (Rothschild., 1995. A “cryptic” microbial mat: A new model ecosystem for extant life on Mars) summarized in (Cockell et al., 2009. Cryptic photosynthesis—extrasolar planetary oxygen without a surface biological signature : table 1).
Desert crusts with a euphotic zone for photosynthesis only a few mm thick can be much more productive. Garcia‐Pichel et al recorded 950-2640 g O2/m²/y in a desert crust in Utah, and other results for desert crusts are similar (Garcia‐Pichel, et al., 1996. Microenvironments and microscale productivity of cyanobacterial desert crusts), summarized in (Cockell et al., 2009. Cryptic photosynthesis—extrasolar planetary oxygen without a surface biological signature : table 1).
Another possibility is a grit crust. This is a mix of lichens, fungi, algae and cyanobacteria. They coat pebbles and glue small rocks to each other. The optimal level of water for photosynthesis was 0.25 mm per day ((Jung et al, 2022, The grit crust: A poly-extremotolerant microbial community from the Atacama Desert as a model for astrobiology), summarized in (Lee, 2020, Newfound desert soil community lives on sips of fog). Perhaps lichens and cyanobacteria could form such a crust using the brines detected by Curiosity? I couldn’t find figures for oxygen production from a grit crust.
Rothschild found a much higher figure in an intertidal cryptic microbial mat of 13,000 g O2/m²/y (Rothschild et al., 2002. Photosynthesis below the surface in a cryptic microbial mat). If Martian life exist and is as productive as that mat it can produce the measured signal of 26.6 grams of oxygen per square meter using much less than a hundredth of the light flux perhaps because it is only biologically active for a hundredth of the year, for instance in biofilms that retain water through to day time like the ones we looked at in:
Or perhaps covering less than a hundredth of the surface or some combination such as biologically active for a tenth of the year and occurring as a cryptic mat over a tenth of the surface,
We see that 26.6 g O2/m²/y is too high a figure to explain as life inside the rocks themselves in Martian conditions. It could be due to microbial mats below the surface or a more productive desert crust.
Such a fast metabolism would be a challenge in the very cold brine layers. However, perhaps if a biofilm can retain the water through to the much warmer daytime temperatures, it can make such figures plausible, also, if life is abundant on Mars, present at both Viking lander sites for instance.
There’s another way such high levels of oxygen production could be more plausible. Terrestrial photosynthesis is far from optimal in its use of energy at low light levels. Martian life has lower light levels and often obscured further by the dust. It could have evolved a faster form of photosynthesis than the Calvin cycle. Then if it had a variable antenna size to cope with dust storm conditions, this would let it use light more efficiently at all light levels. It could also improve on most terrestrial photosynthesis by capturing nearly the full spectrum of sunlight (like seaweeds). Combining all these possibilities, Martian life could potentially achieve roughly a ten-fold increase in efficiency compared to terrestrial photosynthesis.
On Mars there would be evolutionary pressure for more efficient photosynthesis to make better use of the light in dust storms, and also competition to make best use of the limited opportunities to take up the CO2 rapidly as habitable conditions become less abundant in a cooling Mars with a thinner atmosphere. That could be combined with photosynthesis that became more efficient by chance if the low efficiency of terrestrial photosynthesis is indeed due to "pulling up the ladder" on evolution of photosynthesis as Barns suggested (Bains et al., 2016. The cosmic zoo: the (near) inevitability of the evolution of complex, macroscopic life). We discuss these possibilities above.
Martian life could also be adapted to the colder conditions on Mars with a faster metabolism, using chaotropic agents such as the perchlorates which can speed up reactions at low temperatures.
Trainer who is the lead author of the paper on the Curiosity oxygen results, interviewed by Scientific American, put it like this:
On Earth, photosynthesis and respiration by living things cause tiny fluctuations in our planet’s otherwise steady oxygen concentration. We shouldn’t expect this on Mars, though. “That’s far out,” Telling says: Mars appears too inhospitable for a critical mass of life capable of sustaining either process. “It’s almost certainly going to be a nonbiological chemical reaction.”
Trainer herself does not rule out a biological explanation, but nevertheless underscores its unlikeliness. “People in the community like to say that it will be the explanation of last resort, because that would be so monumental,” she says. There are abiotic mechanisms aplenty, both known and unknown, to rule out first before leaping to any more sensational claims.
(Andrew, 2019, NASA’s Curiosity Rover Finds Unexplained Oxygen on Mars)
It would be a surprise to see the signal of a Swansong Gaia so easily as this. Martian life might only inhabit the RSLs and other limited habitats, or be limited by the presence of nitrates, or it might not have developed photosynthesis. If it does occur in these brines it might have a very slow metabolism.
Oxygenic photosynthetic life on Mars could be expected to generate a signal like this, but perhaps a weaker one, easily masked by the naturally occurring oxygen in the atmosphere. This level of oxygen would require a highly productive cryptic near surface microbial mat or desert crust or similar. However, it seems not impossible that biology explains all or most of the signal especially if life on Mars has evolved ten times more efficient photosynthesis than in terrestrial deserts, to make best use of the low light levels on Mars.
Potential limits on biomass of a Swansong Gaia on Mars based on free CO and H2 in the atmosphere do not rule out Swansong Gaia
Sholes et al have suggested a limit on the amount of life there could be on Mars based on the CO and H2 in the atmosphere. The CO is produced photochemically from the carbon dioxide, and so they can work out how much there should be and compare it with the amount there would be if life was able to take advantage of it.
They argue that these sources of energy are easily available to Martian microbes using ancient metabolic pathways of terrestrial life. They argue that such pathways involving simple reactions should evolve easily on Mars. On the assumption that microbes on Mars would use these gases they work out a maximum Martian biomass of 2×10¹¹ kg or 10²⁷ cells (Sholes et al., 2019. A maximum subsurface biomass on Mars from untapped free energy: CO and H ₂ as potential antibiosignatures). The calculation is based on a minimal basal power requirement for life of 3×10⁻²³ kJ s⁻¹cell⁻¹. However the minimal amount to avoid racemization of cells is 10⁻²⁴ kJ s⁻¹cell⁻¹ so in principle 30 times more cells would be possible.
Note that this use of CO as an antibiosignature has been challenged. Life could generate CO indirectly. The CO could be produced by photochemical processing of methane into CO in the atmosphere, with the methane perhaps produced by methanogens originally, in a model motivated by an Archaean Earth (Schwieterman et al., 2019. Rethinking CO Antibiosignatures in the Search for Life Beyond the Solar System : 3)
However, if we can use the Sholes et al estimate, it works out at a limit on biomass of around 1.4 gram per square meter of the Martian surface of 144.8 million square kilometers or an average of 700 million cells per square centimeter for life that subsists off CO and H2 in the atmosphere.
Their figure of around 1.4 g/m² of biomass or 7 × 10⁸ cells / cm² is not unreasonable as an approximate limit to the amount of surface life on Mars, especially since the biomass would also be localized (very little probably in the southern uplands) and patchy.
Terrestrial cell counts at the cold-arid limit of life are often of the order of about 1000 cells / gm (Goordial et al., 2016. Nearing the cold-arid limits of microbial life in permafrost of an upper dry valley) which is well below 7 × 10⁸ cells per cm². Even a global habitable layer to a depth of 20 centimeters with ten times this cell density would contribute cells, 0.1% of their limit and would not contribute enough biomass to have any noticeable effects on the CO globally.
Curiosity was able to monitor CO, but the measurements were hard to do. The signal is 15% ionized CO and 85% CO2 fragmenting into CO⁺. It behaves much like a passive tracer gas in the atmosphere, tracking the signal from argon. They spotted a significant increase after sol 1000. This may be suspect and involve either contamination or instrument errors (Trainer et al., 2019. Seasonal variations in atmospheric composition as measured in Gale Crater : 3013). However, it is possible that future investigations do find signatures of life that uses the CO, if this varies seasonally.
Dry ice processes to maintain the Swansong state and timescale for the biological pump to reduce habitability to a barely habitable Swansong state after a pulse of CO2 and implications for restoration ecology or terraforming
[ADDCITES] [MID EDIT]
The south pole of Mars is a high plateau, far higher in altitude than the north pole which was the floor of an ancient ocean now dried up. Because of that Mars has a north polar cap of ordinary ice but the south polar cap is mainly dry ice which freezes out at a much lower temperature. I'll use the words "evaporate" and "freeze out" (or "condense") instead of the more technical "sublimate" and "deposit" for the transition of dry ice directly to carbon dioxide gas and back again. I'll use "axial tilt" for "obliquity".
First we need to know about how the tilt of Mars' axis affects the temperatures at the poles. Without a heavy Moon its axis can change its tilt far more than for Earth (its obliquity). When the axis is less tilted dry ice and water vapor condense out more and it has larger polar ice caps. When it is more tilted the polar regions don't get so cold and the ice caps are small. At very high angles the polar ice caps may disappear altogether and be replaced by ice in equatorial regions. At angles between 30° and 50° the water ice caps are small and the dry ice at the south pole all evaporates and the atmosphere is at its thickest.
N.B. this illustration may be out of date, the models show no ice cap between 30 and 50 degrees and then an ice cap at the south pole again at above 50 degrees.
(NASA, 2011, Changes in tilt of Mars' axis)
Stamenković et al calculate that when the axial tilt is small as in the lower illustration, which could last for millennia, the water would all freeze out and without the protection of the water vapor, any CO2 splits into CO and O with the oxygen not condensable at those temperatures leading to a highly oxidising environment, however the CO2 would also freeze out so it would be necessary to model the process in detail to find out how much of the CO2 splits before it freezes out (Stamenković et al., 2018, O2 solubility in martian near-surface environments and implications for aerobic life : Supplementary information : 2.2.5.2.2). Those are also the warmest conditions.
When the obliquity is high as in the top right illustration, it is possible that the south polar ice cap of dry ice sublimes out and the atmospheric pressure rises to 12 millibars (Stamenković et al., 2018, O2 solubility in martian near-surface environments and implications for aerobic life : Supplementary information : Table S4). The global annual average temperature falls by 10.3 C at a 90 degree tilt compared to a tilt of 5 degrees while the minimum annual average (at the south pole) increases by 30.1 C if it remains at 6 millibars. However, if the dry ice in the south polar ice cap evaporates it is warmer when at a 90 degree tilt and the average temperature falls by only 6.9 C and the minimum temperature rises by 3.1 C.
It varies from
- 5 degrees tilt: global annual average 205.7 K (-67.45 C) and minimum annual average (at south pole) 144.4 K (-128.75 C)
- 25 degrees tilt (current obliquity) global average 203.703 K (-69.447) and minimum 161.8 K
- 90 degrees tilt: global annual average 195.4, (-77.75 C) [or 198.8 (-74.35 C) if the southern ice cap has evaporated] and minimum 177.7 K (-95.45 C) [or 179,5 K (-93.65 C)]
Even when the axis is almost vertical , when there is almost no water vapour left in the atmosphere, they are still conditions where some of the possible habitats for surface life may continue (if they exist today). For instance since there are small ice caps at all axial tilts, ice on sun facing slopes would still melt due to the solid state greenhouse effect,. There would be subglacial melt, and also the cryoconite holes where sun warmed dust drills into the ice and forms miniature greenhouses capped by translucent ice, also meteorite impacts would continue to throw up ice boulders from the subsurface in warmer regions of Mars.
Then when the tilt is high, the polar regions are warmer, which is relevant to the potential fresh water habitats due to the solid state greenhouse effect at the poles, subglacial melt and cryoconite holes.
Meanwhile at all axial tilts, there can be life in any subsurface caves heated by geothermal processes and open to the surface and in fumaroles and at all axial tilts there is some atmosphere left, possibly increasing to 12 millibars at high axial tilts if the south polar ice cap of dry ice evaporates completely. For some of the many potential surface and near surface habitats and microhabitats that have been proposed for Mars see:
In this situation the atmosphere is kept in equilibrium by the vapor pressure of dry ice. For as long as there are substantial amounts of dry ice at the south pole, then the pressure of the atmosphere is dependent only on the varying temperature of the dry ice sheet and the partial vapor pressure of dry ice at that temperature and since carbon dioxide mixes very well then it will soon reach close to an equilibrium throughout the atmosphere, of course with seasonal variations.
We would expect any biological pump that maintains the Swansong Gaia to be most active when the atmosphere is thickest and liquid water able to form more easily and that also is when the dry ice cap is gone. At that point the atmospheric pressure could be sensitive to the biological pump.
It is also possible that the atmosphere is out of equilibrium and thinner than it needs to be. Radar measurements found that the dry ice at the poles is capped by water ice which may be preventing it from evaporating.
Bierson et al suggested that at the current axial tilt of 25 degrees the dry ice is trapped by this water ice.They calculate that 10 meters thickness would be enough to trap the dry ice and the measurements suggest 15 to 60 meters thickness.
Our modeled results indicate that approximately 10 m of water ice is enough to stabilize the CO 2 ice even with a large thermal wave (Figure S2)
(Bierson et al, 2016, Stratigraphy and evolution of the buried CO2 deposit in the Martian south polar cap : 4175)
Our measurements of the dielectric properties of the bounding layers are consistent with nearly pure water ice between 15 and 60 m thick. Modeling described in section 4 finds that these thicknesses are sufficient for reducing the sublimation rate of CO2 ice to near-zero values.
(Bierson et al, 2016, Stratigraphy and evolution of the buried CO2 deposit in the Martian south polar cap : 4178)
Though the paper doesn't look into this, this leads to an intriguing possibility that the south pole may have more dry ice that could perhaps be liberated by breaking through the ice sheet somehow, if this cap is the reason the atmosphere is as thin as it is.
This shows the trapped layers of dry ice:
Colourized radiograph of the south polar cap dry ice reserves trapped by capping layers of water ice, from (Buhler et al., 2021, Obliquity-Driven CO2 Exchange Between Mars' Atmosphere, Regolith, and Polar Cap : Figure 1)
Bierson et al. did a detailed model of the evolution of the Martian atmosphere over the last million years based on radar evidence of accumulated dry ice at the poles, and minimum ages for the observed dry ice reservoirs capped with ice. They found that for much of the time the dry ice does evaporate completely but most of it is currently blocked from evaporating by the capped ice.
The first row in this figure shows the tilt of Mars' axis, which we can work out projecting backwards based on its current tilt and the dynamics of the solar system over short timescales like this. At present its axis is at an angle of about 25 degrees. In the past million years it has varied between 35 degrees and 15 degrees.
We are especially interested in what happens to the atmospheric pressure in the second row.
Text on graphic:
At plateaus all dry ice has evaporated, Warming liberates 12.5 millibars through to here, Warming now liberates only 6.7 millibars
During these dips the south pole is too cold for a runaway greenhouse effect. Dry ice freezes out at the south pole in dips.
Model runs without insulating caps of ice covering the dry ice, Model runs with caps, lowest ice cap formsThis ice cap is thick enough to prevent warmed dry ice below from breaking through (e.g. as dry ice geysers)
From: (Bierson et al, 2016, Stratigraphy and evolution of the buried CO2 deposit in the Martian south polar cap : figure 4)
The current conditions are unusual in their model. Most often, Mars either doesn't have any dry ice cap, or when it forms, it is temporary for a few tens of thousands of years. It has a long term equilibrium with the highest level of 12.5 millibars of pressure, with seasonal variation from 11 to 13.5 millibars (Bierson et al, 2016, Stratigraphy and evolution of the buried CO2 deposit in the Martian south polar cap : 4176), with a few dips down to 3 millibars, and for a few millennia down to less than 2 millibars at around 800,000 years ago.
However, in the last 300,000 years Mars's tilt hasn't varied so much, only by a few degrees above and below 25° tilt. In their model, the dry ice got trapped at the poles in three layers of sequestration each capped by a layer of ice that prevents it evaporating. Bierson et al. calculate that a cap of 10 meters of water ice is enough to prevent the dry ice evaporating (Bierson et al, 2016, Stratigraphy and evolution of the buried CO2 deposit in the Martian south polar cap : 4176). It's not yet clear in detail what controls depositing a cap of water ice instead of dry ice at the poles though it may relate to the position in Mars' orbit where Mars is closest to the sun relative to the position in its orbit of the equinoxes (longitude of perihelion) at the time of the accumulation of the dry ice or water ice (Bierson et al, 2016, Stratigraphy and evolution of the buried CO2 deposit in the Martian south polar cap : 4178).
However other authors, Buhler et al see the capping layers as temporary. They melt and reform over the dry ice. This is shown first in a presentation from 2019 (Buhler et al, 2019, "The Origin of Mars’ South Polar Massive CO2 Ice Deposit from Co-Evolution with Mars’ Atmosphere")
Buhler et al estimate that the first capping layer formed at the previous dip, 400,000 rather than 300,000 years before the present, and the second capping layer at 200,000 before the present, with a slight adjustment in depth at 100,000 years before the present. (Buhler, 2023, A 510,000 Year Record of Mars' Climate)
From: (Buhler, 2023, A 510,000 Year Record of Mars' Climate : figure 2)
With the ice capping layers now shown like this:
From: (Buhler, 2023, A 510,000 Year Record of Mars' Climate : figure 3)
So they don't see the current atmosphere as trapped under ice. Instead the ice remains at the poles because there isn't currently enough sunlight to liberate it.
We can compare them side by side, Buhler on the left with water ice layers that don't trap the dry ice, and Bierson on the right with layers that do trap the dry ice.
Notice how in the Bierson et al graphic on the right, the atmospheric pressure rises only part way, and then stops as the axial tilt increases, because it is trapped by the water ice. This means there is more dry ice that would be liberated if the water ice could be broken through in some way.Text on graphic: South pole dry ice is gone at 12.5 millibars
Water ice layers now trap all except 6.7 millibars
Bierson et al., 2016
Water ice layers don't trap the dry ice. Buhler et al, 2023.
From: (Bierson et al, 2016, Stratigraphy and evolution of the buried CO2 deposit in the Martian south polar cap : figure 4) and : (Buhler, 2023, A 510,000 Year Record of Mars' Climate : figure 2)
From both these studies, it is clear there is only around 12 millibars of atmosphere in the form of dry ice, available for a warming greenhouse effect, at least for some way above the current warming levels, because if there is more available, it would have been triggered by the extra warming of the extra sunlight at the south pole for high levels of axial tilt.
So at least for the start of any attempt at warming to thicken the atmosphere beyond 12 millibars, we already see there is no extra dry ice available to be liberated. Buhler et al found that some of the dry ice from the poles gets taken up by the regolith which may have as much as 100 millibars of dry ice in the top few hundred meters, and some of that could be liberated after the dry ice is all gone, leading to an estimated range of the maximum atmospheric pressure at around 40 degrees axial tilt of 7.6 to 14.5 millibars (Buhler et al., 2021, Obliquity-Driven CO2 Exchange Between Mars' Atmosphere, Regolith, and Polar Cap : table 1). See below:
If Bierson et al are right and the dry ice is trapped by those ice layers capping the dry ice not even that much can be achieved by natural processes. From their figure, the current warming which started around 40,000 years ago only liberated around a tenth of a millibar.
However Bierson et al's model is good news for terraformers and those who want to attempt restoration ecology (also called ecopoesis), since the south pole seems to be warm enough to at least release more dry ice than it has at present. Perhaps we could liberate it just by drilling through the ice. First the surface water ice cap needs to be melted or drilled through. Then as the dry ice sublimates, the next layer below that and then the third layer also, before it can go all the way to 12 millibars.
Bierson don't discuss the longer timescale. But if the ice accumulation is due to the longitude of the perihelion at the time of the accumulation of the dry ice or water ice (Bierson et al, 2016, Stratigraphy and evolution of the buried CO2 deposit in the Martian south polar cap : 4178). these conditions must occur frequently. It also seems a remarkable coincidence to spot Mars in this state if it is the first time in 3 billion years that its atmosphere got into a state like this.
So if Bierson et al. are right, there is likely to be some way in the future for Mars to liberate those now buried layers to restore the conditions before they were buried. If they are trapped, perhaps they would be released after a strong enough warming pulse of emissions from the glaciers?
However, from Buhler et al.'s results it seems the capping layers are just temporary and may dissipate by themselves whenever the axial tilt gets high enough to expose them again, and their model shows the dry ice continuing to evaporate in the present day as the axial tilt increases.
Could there be alternating episodes of runaway warming and runaway atmospheric collapse in early Mars - and could we trigger a runaway warming today?
[PRELIMINARY MATERIAL - PENDING INFORMATION FROM THE AUTHORS OF THE PAPERS]
[SUGGESTIVE OF A NEW FLASH FLOODING BIOLOGICAL OR CHEMO-PHYSICAL FEEDBACK HYPOTHESIS??]
In 1991 without the detailed information available about the south polar cap we have today, McKay et al. worked backwards from the pressure of of Mars of 6 millibars to calculate the temperature of the dry ice at the south pole, to be in equilibrium with the 6 millibars atmospheric pressure.
That led to this figure. The dashed line shows the CO2 vapor pressure, i.e. pressure of CO2 needed in the atmosphere above dry ice to keep it stable, neither evaporating or condensing.
From this figure we read that at point A dry ice would be stable at around 6 millibars and a temperature of between 135 and 140 K. The solid line shows the temperature that the dry ice would reach with the current axial tilt depending on the pressure in the atmosphere.
McKay et al assume that point A is at the correct temperature for a vapor pressure of 6 millibars as they didn't have good direct measurements in 1991 and by modeling the atmosphere including the greenhouse effect based on this assumption they were able to plot this diagram.
The other temperatures for the south pole for different atmospheric pressures are shown with the solid line and the vapor pressure to keep dry ice in equilibrium is shown by the dashed line on the same graph.
Text on graphic: This feedback loop keeps Mars' atmosphere in equilibrium at a low pressure, in this case 6 millibars, if the south pole is cold enough.
In the orange region the dry ice is too cold to sustain the atmosphere as vapor over dry ice and it freezes out until it reaches point A.
Plot of polar temperature on Mars and the CO2 vapor pressure on the same graph.(Marinova et al., 2005. Radiative-convective model of warming Mars with artificial greenhouse gases : figure 1) adapted from (McKay et al., 1991, Making Mars habitable : figure 3) which has a longer discussion in (McKay et al., 1991, Making Mars habitable : 492). This in turn is a medication accounting for the greenhouse effect of equation 20 of (Gierasch et al., 1973, Atmospheric Pressure Variation and the Climate of Mars : 1505)
Based on this diagram it seemed likely the Martian atmosphere has collapsed out as dry ice in balance with CO2. If more CO2 is either added from volcanoes or is liberated from the dry ice cap to push the pressure up to a point between A and B we see that the temperature line is below the vapor pressure line. This means that the temperature at the south pole will be too cold to sustain any higher level of vapor pressure above the dry ice and so the carbon dioxide will quickly condense out again pushing it back to point A.
If McKay et al. had found that the atmospheric pressure was higher, say 12 millibars (as it would be at higher axial tilt) then they'd have put point A further to the right and the distance between A and B would be less needing less warming for a runaway. If they'd found the pressure was lower, say 2 millibars, then they would have put point A further to the left and point B further to the right needing more warming to trigger a runaway.
This is the basic assumption I'm making about how their model works [will check to confirm]. Assuming this is correct, then we can get some interesting conclusions combining their ideas with the latest research.
If we go back to McKay et al.'s model, and increase the sunlight at the poles, it shouldn't take much extra sunlight to eliminate this low pressure point of stability and the feedback that causes the atmosphere to collapse.
Their model is based on a more complex equation summarized in equation 1 of (McKay et al., 1991, Making Mars habitable : 492). So this would need to be recalculated for different atmospheric pressures.
However for a first approximation (pending more detailed investigation by someone, I will try contacting the authors of these two papers for more information) but for now, let's just shift their theoretical polar temperature curve up until the first point where there would be no low pressure point of stability for any amount of CO2.
This shows how the model would fit with Buhler's idea of a capping layer preventing the dry ice from evaporating (Bierson et al, 2016, Stratigraphy and evolution of the buried CO2 deposit in the Martian south polar cap : 4175). In this case the polar dry ice cap is already warm enough for a runaway greenhouse effect, but it is prevented from doing this by the water ice cap.
Text on graphic:Yellow curve assumes a warmer south pole - just shifting the curve up as a first approximation.
If Buhler et al. are right and the dry ice is capped by water ice, drilling through the
water ice might trigger a runaway without extra warmingThis point may be below the curve if the dry ice is trapped below water ice and not in equilibrium (arrow to point A).
[NOT MODELLING, JUST SHIFTING THE TEMPERATURE PRESSURE CURVE FROM McKay et al FOR A FIRST APPROXIMATION]
(Marinova et al., 2005. Radiative-convective model of warming Mars with artificial greenhouse gases : figure 1) adapted from (McKay et al., 1991, Making Mars habitable : figure 3)
Interestingly their theoretical curve meets the vapour pressure curve at close to 11 or 12 millibars which is remarkably close to the maximum amount of dry ice easily available to evaporate.
Text on graphic: As a first approximation just by shifting up the curve (in reality it is a complex model) it seems no amount of runaway warming or sudden collapse of the atmosphere is possible when the dry ice available falls to below ~11 millibars.
At around 11 millibars there is no low temperature point of stability for atmospheric collapse at any axial tilt.
Plot of polar temperature on Mars and the CO2 vapor pressure on the same graph. This time the theoretical polar temperature curve is shifted up enough to achieve a runaway greenhouse. Interestingly this happens at about 11 millibars, not far from the current estimated dry ice reserves of 12.5 millibars.
[NOT MODELLING, JUST SHIFTING THE TEMPERATURE PRESSURE CURVE FROM McKay et al FOR A FIRST APPROXIMATION](Marinova et al., 2005. Radiative-convective model of warming Mars with artificial greenhouse gases : figure 1) adapted from (McKay et al., 1991, Making Mars habitable : figure 3)
This also shows the minimum amount of CO2 needed to permit McKay et al.'s scenario of two points A and B to happen at all, at any amount of axial tilt. I.e. the amount of CO2 Mars needs to enable any such scenario with a point of stability at A for an atmospheric collapse when it falls below point B, and in the other direction, enough CO2 available for a runaway warming to get to above point B when warmed enough, whether by greenhouse gases or changing axial tilt.
The sudden runaways would seem to stop at around 11 millibars. There would need to be more than 11 millibars equivalent of CO2 available to have any potential for a runaway collapse or inflation even by a few millibars.
This would also mean that if there is more than 11 millibars of CO2 easily available, then when the tilt is enough for the atmosphere to reach 11 millibars naturally, it will always go on to do a runaway and lose all the rest of its easily lost dry ice to the atmosphere, as it would no longer have McKay et al.'s two points A and B. Before 11 millibars it still has those points A and B and so can't do even a small runaway.
We can also look for the first point in a thinning atmosphere where the atmosphere would collapse to the poles. Here we just use the data that the atmosphere freezes out at around 1.5 millibars for the highest axial tilt in the last million years in (Bierson et al, 2016, Stratigraphy and evolution of the buried CO2 deposit in the Martian south polar cap : figure 4)
As a first approximation shifting the temperature curve down, the atmosphere would be able to collapse down to 1.5 millibars (for 15 degrees axial tilt) at around 90 millibars so the first runaway collapse might happen at around 100 millibars or so.
Plot of polar temperature on Mars and the CO2 vapor pressure on the same graph. This time the theoretical polar temperature curve is shifted down to meet the curve at around 1.5 millibars for the stable equilibrium. The unstable end point, the atmospheric pressure for dry ice to form for the first time and the atmosphere to collapse is at around 90 millibars.
[NOT MODELLING, JUST SHIFTING THE TEMPERATURE PRESSURE CURVE FROM McKay et al FOR A FIRST APPROXIMATION](Marinova et al., 2005. Radiative-convective model of warming Mars with artificial greenhouse gases : figure 1) adapted from (McKay et al., 1991, Making Mars habitable : figure 3)
As a first approximation it looks as if as the atmosphere gets thinner that it would first collapse at around 9 kPA or about 90 millibars. It would certainly happen at some point above the over 30 millibars of McKay et al.
We will find later that this is an underestimate. The atmosphere can collapse at far higher pressures up to 600 millibars when the atmosphere becomes too thin to transport heat to the poles fast enough to prevent the dry ice condensing out.
So, if the atmosphere can be much thicker, why is the present day atmospheric pressure between collapse at around 12 millibars which seems likely to be close to the lowest pressure possible for a runaway greenhouse between collapses?
It would be a remarkable coincidence if this was because of a biological pump that happens to be exactly in balance with emissions from volcanoes and infall from space at this pressure. Could there be some process operating to minimize the amount of any potential runaway?
A way for a Swansong Gaia to minimize atmospheric collapses and inflations: potential for a stronger biological pump during runaway warming in the early Mars atmosphere
Suppose the maximum pressure of the Mars atmosphere was much higher between collapses, say, 30 millibars. We'd not notice any difference at an axial tilt below 30°. As the axis tilts further the atmospheric pressure would just increase gradually in equilibrium with the vapour pressure of carbon dioxide over the south polar dry ice cap, until it reached a pressure of perhaps 11 or 12 millibars, the point at which point a runaway greenhouse can't be avoided at any temperature.
Then it would suddenly all evaporate away in a runaway greenhouse until all the dry ice was evaporated. It would then reach its maximum pressure, say, 30 millibars, and would stay at that level until the axis tilt was reduced enough to reduce light levels at the poles until the first dry ice forms making it unstable at 30 millibars when it would suddenly collapse back down to 6 millibars.
When the atmosphere rapidly thickens, any remaining ice at the poles or in mountainous regions would rapidly melt, and that would lead to flash flooding. It might last for a century or centuries, of the ice draining away through rivers and streams, forming temporary lakes, until it sinks into the desert sands or evaporates into the warmer air at lower latitudes and lower altitudes.
This shows how it would work in an early Mars with a somewhat thicker atmosphere:
Text on graphic: Sudden collapse
Runaway warming with sudden flooding.
Strong biological pump.
CO₂ levels loweredEarly Mars at 30 millibars of atmosphere (as it starts to thin).
When south pole light levels are low enough for the first dry ice to form, it collapses quickly to 6 millibars vapor pressure over dry ice in a runaway cooling, by (McKay et al, 1991)Light levels increase when Mars' axis tilts over.
This triggers runaway warming (at ~12 millibars?)
We expect sudden widespread melt and floodingAdapted by simply shifting the 12 millibars levels up to 30 millibars from: (Bierson et al, 2016, Stratigraphy and evolution of the buried CO2 deposit in the Martian south polar cap : figure 4)
We also expect the dips to be asymmetrical, with the atmosphere only collapsing when it gets very cold at the poles, with a near vertical fall, and then rising slowly to around 12 millibars before suddenly all evaporating again in a runaway effect.
But we don't see this. Instead the dips in atmospheric pressure in (Bierson et al, 2016, Stratigraphy and evolution of the buried CO2 deposit in the Martian south polar cap : figure 4) are symmetrical with no visually distinguishable difference between the two sides of the dips.
Those symmetrical dips also suggest that 12.5 millibars is either below or not far above the lowest pressure where a runaway greenhouse effect starts. If they were above the point where a runaway greenhouse effect can occur, they would be asymmetrical, as we saw, with a dip starting at, say, 30 millibars, and then ending at, say, 6 millibars at the next runaway effect, depending on the dry ice available.
This may just be a coincidence like the coincidence that the Moon is at the exact distance right now to cover the sun as seen from Earth, with the eclipse varying between annular and varying lengths of time of totality. But it is quite a remarkable coincidence.
Is there some interaction between the dry ice effect and a Swansong Gaia that might favor this point somehow? Or is there some chemical or physical process that explains it?
Perhaps either the collapses or the runaways themselves trigger a pump, either physical, chemical, biological or all three, that gets as the amount of the runaway gets less each time, stopping at the point where there are no runaway warming or sudden collapses possible.
If, for instance, 11 millibars is the pressure at which a runaway greenhouse occurs, the current level of 12.5 millibars would mean that after the dry ice warms to the point where it can enter a runaway greenhouse, any runaway can only liberate 1.5 millibars, small enough so that the sudden cooling collapses at the start and the runaway warming episodes at the end of the dips might not be noticeable. This is what one might expect if for some reason feedbacks in Swansong Gaia are acting to reduce the amount of each runaway greenhouse effect as much as possible.
So could there be such a mechanism? One obvious candidate is if it involves flash floods in some way. When the atmosphere rapidly warms up in a century or so, it might well lead to flash floods of the ice stored at high altitudes and over much of the polar region just below the surface as well as the ice caps.
As Mars gradually lost its carbon dioxide atmosphere there must have been a first point when it collapsed, and presumably that would happen when the south pole is coldest and it collapses to only 1.5 millibars or possibly less. Suppose this happened at 90 millibars, then it would be like this:
Text on graphic: Sudden collapse
Runaway warming with sudden flooding.
Each time the runaway warming restores a little less CO₂ than was lost.As Mars loses its atmosphere it would suddenly collapse at some point
First sudden collapses only when the axial tilt is so small that poles get so little sunlight that after collapse the dry ice will have a vapor pressure of only 1.5 millibars - maybe this collapse starts at 90 millibars? [need to check with modelers.
Above 30 millibars for sure]By 12.5 millibars it is no longer able to do sudden collapses or runaway warming at any axial tilt.
Why don't we see an atmosphere at some state between 12.5 and 90 millibars?
Perhaps because the sudden warming itself creates especially habitable conditions and a brief strong biological pump?
Adapted by simply shifting the 12 millibars level before and after the lowest dip up to 90 millibars from: (Bierson et al, 2016, Stratigraphy and evolution of the buried CO2 deposit in the Martian south polar cap : figure 4)
Though as we saw, other research suggests it happens far earlier at 600 millibars which we go into in detail below:
How chemical weathering or biological pumps could be enhanced during the episodes of flash flooding on Mars however they were caused
We do know that Mars has had many episodes of flash flooding, whether they were caused by this hypothesis of a sudden runaway warming and collapse or in some other way.
There are many deep river networks on Mars that show that large rivers flowed in the past.
River network in Osuga Valles likely caused by sudden flooding rather than steadily flowing rivers from gradual seepage
(Seybold et al, 2018, “Branching geometry of valley networks on Mars and Earth and its implications for early Martian climate.”) press release (uchicago news, 2018, Rain or snow likely formed Martian rivers)
This is an example of a river course likely formed by flash floods from craters that filled with water until the crater wall breaks
This river likely formed when the wall of a flooding crater was breached leading to a flash flood.
(Goudge, 2017, The importance of lake breach floods for valley incision on early Mars) press release (Kortsha, 2017, Mars’ Surface Shaped by Fast and Furious Floods from Overflowing Craters)
Let's go back to the time of early Mars to when the atmosphere is 90 millibars as for this diagram:
Text on graphic: Sudden collapse
Runaway warming with sudden flooding.
Each time the runaway warming restores a little less CO₂ than was lost.As Mars loses its atmosphere it would suddenly collapse at some point
First sudden collapses only when the axial tilt is so small that poles get so little sunlight that after collapse the dry ice will have a vapor pressure of only 1.5 millibars - maybe this collapse starts at 90 millibars? [need to check with modelers.
Above 30 millibars for sure]By 12.5 millibars it is no longer able to do sudden collapses or runaway warming at any axial tilt.
Why don't we see an atmosphere at some state between 12.5 and 90 millibars?
Perhaps because the sudden warming itself creates especially habitable conditions and a brief strong biological pump?
Adapted by simply shifting the 12 millibars level before and after the lowest dip up to 90 millibars from: (Bierson et al, 2016, Stratigraphy and evolution of the buried CO2 deposit in the Martian south polar cap : figure 4)
At the start of a dip, as the dry ice freezes out, in a few centuries falling from say 90 millibars to 1.5 millibars, rain, sleet and snow would also fall.
There is much more ice at the north pole because it is at a lower elevation. As Mars gets colder it would favour snow at the north pole, dry ice snow at the south pole, but wherever the water ice snow falls, it would have more and more dry ice mixed in as the process continues. The snow would tend to favour high altitudes and the polar regions. Even at the start when not much dry ice is mixed in with the snow yet, even when it is falling as rain, the rain would be very carbon dioxide rich water.
So then in the sudden runaway at 1.5 millibars or 2 millibars all that carbon dioxide rich ice melts. The dry ice would evaporate very fast, but the ice might take tens of millennia probably, maybe even hundreds of thousands of years because of the high thermal inertia of ice, just as for the very long timescales to melt the ice caps in Greenland and Antarctica.
These would spread out to make large shallow lakes in the many craters on Mars. Then, because of the higher vapor pressure of the warmer water compared to solid ice, many of these lakes would quickly evaporate, especially the first water to spread in the initially dry atmosphere formed by the runaway greenhouse effect of the dry ice.
The floods of water would also sink into the desert sands which would get wet and then the wet desert areas in the equatorial regions especially would dry out in the warmth of the sun and fall again as snow or rain on the mountains and in the polar regions and so they would replenish the reservoirs of ice ready for the next dip. During the atmospheric collapse the snow and ice would form once more in the polar regions and at high altitudes.
So in this way there would be a period of several millennia, possibly tens of thousands of years of flash flooding after the runaway greenhouse as the climate exits the dip, with the water rushing through river valleys and spreading out into large shallow lakes, ideal conditions for stromatolites, algal mats and algal blooms, as well as martian equivalents of the forams and coccolithophores with their microscopic calcite shells, if such form there.
See above:
Forams on Earth don't just occur in the sea, they can also be found in fresh water and in soils (Holzmann et al, 2021, Review: Freshwater and Soil Foraminifera – A Story of Long-Forgotten Relatives). The coccolithophores which formed the great cliffs of Dover can also be found in fresh water as well as in polar habitats even though they are best known as a tropical or subtropical genus (Heimdall, 1997, Identifying Marine Phytoplankton : Chapter 6, Modern Coccolithophorids - extract publicly avaialble via ScienceDirect). So there are many ways these shallow lakes could encourage carbon sequestration for as long as they last.
The carbon dioxide rich acidic water would react with alkaline rocks such as granite carving out caves and forming carbonates. Mars should have huge caves far larger than on Earth in the low gravity and it would carve those out in sedimentary rocks and also in igneous rocks (rocks that solidify out of lava) such as granite.
Granite heart shaped rock (NASA, 2014, Rockin' Valentines!).
Geologists were surprised to find granite on Mars because it requires rock to remain molten for long periods for the lighter granite to separate out of the melt, but they now think that these conditions may occur in some of the very long lived volcanoes on Mars (Wray, et al., 2013, Prolonged magmatic activity on Mars inferred from the detection of felsic rocks."), press release: (NASA, 2013, Evidence found for granite on Mars)
Terrestrial granite caves are particularly biologically active and often have rock encrustations, "speleotherms", enriched with organics from life, including organic rich opal stalagmites and stalactites.
Water seeping through granite caves forms mineral deposits called speleotherms and this is enhanced with biological activity which can end up with large amounts of organics including carbon trapped in the stalagmites and stalactites and other structures. The opal stalagmites in granite caves often have carbon content of more than 50% (Romani et al., 2010, Speleothems of Granite Caves : Table 1).
There are speleotherms in basalt caves too, the normal igneous rock covering much of Mars (Ford, 2018, A geochemical study of speleothems and cave waters in basaltic caves at Lava Beds National Monument, Northern California, USA). Also Mars has large areas of sedimentary rocks such as sandstone (NASA, 2016, Knobbly Textured Sandstone on Mount Sharp, Mars). There are of course many caves in sedimentary rocks and they also have their microbial communities and speleotherms (Aubrecht et al., 2007, Anatomy of biologically mediated opal speleothems in the World's largest sandstone cave: Cueva Charles Brewer, Chimantá Plateau, Venezuela)
The caves could be far larger in the low gravity of Mars. It's hard to see most caves from satellites overhead. However we do know that Mars has lava-tube caves in basalt from satellite observations and they can be up to hundreds of meters in diameter.
The mouth of this cave in Mars' Arsia Mons region is at least 50 meters across, bigger than similar caves on Earth and the tube inside is likely at least this big.
(NASA, 2021, HiRISE Spots the Mouth of a Martian Lava Tube ).
Lava tubes on Earth range from 10 to 30 meters in diameter. The caves are typically much wider than they are high and the height ranges from from 40 to 400 m on Mars and 500 to 900 meters on the Moon. (Sauro et al., 2021, Lava tubes on Earth, Moon and Mars: A review on their size and morphology revealed by comparative planetology : 13) ESA story: (ESA, 2020, Take refuge in a cave on the Moon)
The cave entrances themselves are also good habitats for photosynthetic life, as on Earth and more so on Mars where the extra shielding from UV is useful (Viudez-Moreiras, 2021, The ultraviolet radiation environment and shielding in pit craters and cave skylights on Mars)
So those sudden flash floods during runaway greenhouse on Mars could trap a lot of carbon dioxide as speleotherms in likely vast underground caves both through chemical weathering and also by life growing in the water and getting trapped in the speleotherms.
Mars likely has the full range of cave types Earth has including also caves not found here such as ones formed by the action of dry ice. Caves on Earth are often very biologically active. See above:
This is just to sketch out an idea and of course needs closer examination.
The chemical and physical processes which could react with alkaline rocks and deposit carbonates in caves and crevices and cracks could be worked out without any knowledge of any martian biosphere. The biological processes require considerable speculation at this stage. We can look at scenarios using terrestrial analogues but the details may need to wait for whatever we find out about Mars' biosphere if any.
[END OF MATERIAL PENDING MORE INFORMATION]
Estimated 100 millibars of dry ice absorbed in the regolith - is this involved in any feedback?
Could there be a physical feedback that could freeze out the atmosphere into dry ice? A fair bit of the dry ice would freeze out in the regolith and caves during the runaway collapse episodes. There seems to be a significant amount of dry ice in the regolith, Around 100 millibars (Buhler et al., 2021, Obliquity-Driven CO2 Exchange Between Mars' Atmosphere, Regolith, and Polar Cap : table 1). We will look at this in the next section:
A lot of this would be restored to the atmosphere during the runaway greenhouse as the floods warm the regolith and the caves. But some of it would remain frozen out even after the runaway warming and this could be another effect that would tend to make the dips a little asymmetrical rising not quite so high as they fall.
In present day Mars the dry ice in the regolith is stable and as the axial tilt changes, dry ice freezes out at the south pole and evaporates again for as long as the south pole still has any dry ice left. The south polar dry ice cap vanishes however between 30°–50° tilt. Buhler et al found that when Mars is tilted over a long way, above 50° tilt, the amount of sunlight at the south pole decreases again and the dry ice cap forms once more.
Buhler et al. found that for as long as the dry ice cap exists, around 40% of the evaporating dry ice ends up in the regolith rather than in the atmosphere. This subtracts from the expected final atmosphere.
When there is no dry ice left, the dry ice starts to evaporate from the regolith into the atmosphere, which can add to the atmospheric pressure.
Buhler et al calculate that there's around 100 millibars of dry ice available (68% confidence interval of 52 to 166 millibars). (Buhler et al., 2021, Obliquity-Driven CO2 Exchange Between Mars' Atmosphere, Regolith, and Polar Cap : table 1). Based on the figures they use there, they calculate 320 trillion tons for 86 millibars 3.7 trillion tons to a millibar.
However most of it moves back and forth between the dry ice and the regolith. The maximum atmospheric pressure when the dry ice cap vanishes works out as between 7.6 and 14.5 millibars depending on how much dry ice can be absorbed in the regolith (Buhler et al., 2021, Obliquity-Driven CO2 Exchange Between Mars' Atmosphere, Regolith, and Polar Cap : table 1)
Text on graphic: Wilde range of scenarios for dry ice liberated from the regolith when the dry ice cap vanishes
Between 30°and 50° axial tilt, the dry ice at the south pole vanishes.
Dry ice reappears above 50 degrees.
A lot of the dry ice from the south pole ends up in the regolith when the south polar ice cap evaporates.
Annotations added to (Buhler et al., 2021, Obliquity-Driven CO2 Exchange Between Mars' Atmosphere, Regolith, and Polar Cap : Figure 3D)
Up through to when the polar dry ice vanishes, some of the dry ice from the south pole is absorbed in the regolith. But once it is gone the regolith switches to a source and may liberate all that absorbed dry ice and even significantly more, depending on how much is already absorbed in it at present.
It's likely that most of this is absorbed in the top few hundred meters of regolith.
Text on graphic: Most likely Regolith absorption curve
(Illustrative curve added by hand) annotations added to: (Buhler et al., 2021, Obliquity-Driven CO2 Exchange Between Mars' Atmosphere, Regolith, and Polar Cap : Figure 4D)
We can see that the dry ice is most concentrated in the top 200 meters of the regolith though small amounts are likely present down to a kilometers depth or more.
Text on graphic: Axial tilt (degrees)
Atmosphere (multiples of 10 trillion tons)
Dry ice cap (multiples of 100 trillion tons) m
Maximum surface pressure range 7.6 to 14.5 millibarsDry ice in the regolith (loose rock and dirt) (multiples of 100 trillion tons)
Mars has enough dry ice trapped at its south pole to double its atmosphere to 12.5 millibars. and enough in the regolith to increase it to a tenth of Earth's atmospheric pressure
Modeled history of the dry ice reservoirs in the regolith for various estimates of the absorptive capacity of the dry ice, from (Buhler et al., 2021, Obliquity-Driven CO2 Exchange Between Mars' Atmosphere, Regolith, and Polar Cap : Figure 6)
So that could be a chemical and biological pump feedback that leads to the system evolving to minimize the amount of the greenhouse runaway because each runaway generates flash floods which then help sequester large amounts of CO2 until enough is removed from the atmosphere of Mars over millions and then billions of years to stop those flash floods almost completely. That could explain why we don't see any runaway today.
Although most of the ancient atmosphere ended up in the carbonates, several bars, we have perhaps a tenth of a bar, a tenth of Earth's atmospheric pressure as dry ice in the regolith. If the Martian atmosphere started to collapse at, say, 90 millibars a lot of that early atmosphere might have ended up in the regolith,
However it turns out that it probably first collapsed at a much earlier stage so we look at that next.
The martian atmosphere could have started to collapse at 600 millibars 3.6 billion years ago
Looking at earlier in the history of Mars, Buhler suggested periodic collapses could have happened at an earlier stage at 0.6 bars or 600 millibars (Buhler, 2022, Martian Global-Scale Flooding Triggered by Atmospheric Collapse). The atmosphere collapses whenever the axial tilt falls below ~30°. It can do that because the thing atmosphere can't transport heat to the poles fast enough to prevent the dry ice freezing out.,
Buhler’s model is an explanation for the enigmatic intense valley network period between 3.6 and 3.5 billion years ago, and explains why flash flooding would increase at this time, when most models say it should decrease.
In his model the collapsing atmosphere causes the melt water and flash floods, rather than the runway warming during the inflating atmosphere periods. He suggests that as Mars cools down during the atmospheric collapse, it forms thick dry ice layers on top of the water ice caps at the poles.
This dry ice from the collapsing atmosphere insulates the polar ice caps (because dry ice is a very good insulator). It's then the internal heat of Mars which melts the polar ice sheets from the base upwards, and it then floods Mars. .
Once below ~0.6 bar, the atmosphere began to periodically collapse and reinflate. Collapse occurred whenever Mars’ sinusoidally evolving obliquity (spin-axis tilt) fell below ~30° because a <~0.6 bar inflated atmosphere was too thin to transport sufficient heat to prevent runaway deposition of the atmosphere into polar CO2 ice sheets. The decline below ~0.6 bar likely happened ~3.6 billion years ago, at which time an extensive H2O ice cap covered Mars’ south pole
(Buhler, 2022, Martian Global-Scale Flooding Triggered by Atmospheric Collapse)
This is from a preprint of NASA funded research also written up as a conference paper (Buhler, 2022, Mars' Noachian-Hesperian Intensive Fluvial Activity Driven by Atmospheric Collapse)
Back then the poles were covered in thick water ice, 2-4 kilometers thick, almost thick enough for the base of the ice cap to melt due to internal heat from Mars, but not quite. The collapsing atmosphere adds a 660 meter thick layer of CO2 forms on top of it (maximum thickness before the dry ice melts at the base of the dry ice cap). This extra dry ice layer has three effects. It insulates the water ice, it may form clathrates at the interface between the dry ice and water ice which are also thermally insulating, and the weight adds sheer stresses on the water ice. This then melts the ice completely in a few hundred thousand years, which then floods much of early Mars.
The atmosphere re-inflates when the tilt falls back below 30 degrees.
There could have been one or several of these meltwater pulses each lasting 10,000 to 10 million years within a 100 million year time period during which time the atmospheric pressure fell from 600 millibars to 200 millibars.
Transition from atmospheric collapse onset (~0.6 bar) to inflated atmospheric pressures at which collapse-induced melting was insufficient to breach Argyre (~0.2 bar) likely occurred in ~10 8 years.
[He explains that Argyre is a paleolake near the south pole that likely filled and emptied several times and the research was done to try to explain how that happened]
...
Intense polar meltwater pulses driven by periodic atmospheric collapse are an almost inescapable consequence of Mars’ transition from a stable, thick atmosphere to a collapse-prone, thin atmosphere near the Noachian-Hesperian boundary, given the presence of a south polar H2O ice cap. One or several pulses lasting 10 4 – 10 7 years within a ~10 8 -year timespan are consistent with the development of valley networks from intense periodic erosion during > 10 5 years near the Noachian-Hesperian boundary(Buhler, 2022, Martian Global-Scale Flooding Triggered by Atmospheric Collapse : 2)
Those flash floods would also seem a good candidate for a strong biological or physiochemical pump in Early Mars. However that flash flooding mechanism isn't relevant any more today though the runaway collapse / inflation should still happen.
We don't have such thick layers of dry ice any more.
So once it is down to 200 millibars it might transition to the cycle I sketched based on Chris McKay's two points A and B where there's still some flooding but during the inflation rather than collapse stage of the cycle. See above:
Then the biological pump and weathering may have removed half of those 200 millibars and the rest is still there as dry ice.
Flash flooding continued to around 2 billion years ago
This flash flooding atmospheric collapse / inflation may have ended 2 billion years ago. This seems the most recent evidence of rivers flowing on Mars, from 2 to 2.5 billion years ago though without an explanation of how they formed:
This indicates that surface waters forming chloride deposits continued through the Hesperian and into the Amazonian, making them among the latest-formed, large-scale deposits of water-related minerals on Mars. Collectively, data suggest formation from meltwater from episodic ice/snow deposits continuing until 2.0–2.5 Ga.
...
The chlorides are often found in small depressions, including perched above much deeper craters where no chloride is observed. They are also often found within channels. For these reasons, we think that the water source came from surface runoff, rather than deep groundwater welling up to the surface. The small amounts of water required are most likely from occasional melting of ice.
(Leask, 2021, Evidence for Deposition of Chloride on Mars From Small-Volume Surface Water Events Into the Late Hesperian-Early Amazonian)
It's probably been much like it is today ever since.
Modern weaker pump without the atmosphere collapse and reinflation
Those flash floods have likely ended now, but the biological pump won't have stopped altogether (if there is life there). We still have water flowing when the axial tilt is high enough, at above 35 ° (Dickson et al., 2023, Gullies on Mars could have formed by melting of water ice during periods of high obliquity) press release (Planetary news contributor, 2023, Gullies on Mars Could Have Formed by Melting of Water Ice).
Then there's the Chinese rover discovery of cemented sand dunes that suggests recent liquid water from thawed frost / snow even in equatorial regions probably at high axial tilt (Qin et al., Modern water at low latitudes on Mars: Potential evidence from dune surfaces). That could have led to some carbonates organics sequestered too.
Then at times Mars is more active. For instance the volcanic eruption on the flanks of Arsia Mons 210 million years ago, which likely lead to 100 cubic kilometers of subsurface melt in three lakes, which would have stayed melted for centuries to millennia insulated by surface ice (Scanlon, 2014, A habitable environment on Martin volcano?). Those could have done a bit of CO2 sequestration too.
Also small amounts of CO2 may be sequestered even when it's as dry as it is today, especially if the oxygen variation that Curiosity found is due to photosynthesis. See:
So the flash flooding wouldn't be the only process. It might be that over the last few hundred million or couple of billion years the main process is the biological pump during times when the axial tilt is between 30 ° and 50°, but flash flooding would be the major process early on leading to the situation we find today.
Also if Mars had a pulse of CO2 emissions from volcanoes or comets to bulk the atmosphere up to above the 11 millibars (or whatever the current maximum is), between the atmospheric collapses, the flash flooding would start up again until it is back to current conditions again.
If this is the reason, then perhaps without the biological pump the atmosphere would still be doing its collapses and reinflations whenever the tilt is between 30° and 50°. It would be similar to our present day Mars during those collapses, but have a much thicker atmosphere when the axial tilt is between 30° and 50°.
Without the flash flooding, the biological pump would be weaker, leading to an equilibrium with the CO2 from volcanoes and infall at a higher pressure, so perhaps the atmosphere might still be a little thicker between 30° and 50° with repeated small runaway inflations and collapses.
So then, the combination of the flash flooding and the biological pump is what keeps the atmosphere so thin even at those higher axial tilts in modern Mars.
The 11 millibars (or whatever it is) in McKay et al's graph shifted doesn't seem to depend on whether it is the modern sun or a fainter young sun, only on the vapour pressure of dry ice, if so the flash flooding would have stabilized it at 11 millibars even 2 billion years ago. If so, Buhler's 3 millibars is presumably due to some difference in the situation for collapse of a thicker atmosphere.
If this is why Mars has a thin atmosphere so close to the triple point of water, the life must still persist on Mars because the strong biological pump still has to work during flash floods whenever the atmosphere temporarily thickens after a volcanic eruption, or the atmosphere would gradually start to get thicker again. Which would happen rarely today, but frequently back when it first got down to 11 millibars when Mars was more geologically active.
This means life needs to persist to the present through the very cold conditions of a near-vertical axial tilt when the atmosphere goes down to 1.5 millibars.However there are many ways the life could persist even in a thinner atmosphere than today. Some of the surface habitats I cover in the book would work even at very low atmospheric pressure, especially the subglacial melt and the cryoconite holes at the poles.
See the section of my draft book:
So there could be life on the surface continuously in rare oases even through to the very low levels of CO2. Or life might retreat underground into caves during the times of very low axial tilt as astrobiologists have often suggested. Or it could persist in dormant states.
Either way, the biological pump doesn't need to have much or any activity today because it would only activate strongly after a pulse of CO2 emissions from volcanoes or after a comet impact.
First steps at calculating how long it would take to remove the atmosphere based on chemical weathering or a biological pump
At any rate, whether due to a stronger biological feedback during flash floods, or just a biological pump that removes carbon dioxide slowly over a long period of time, if the thin atmosphere at 12 millibars or so is due to the Swansong Gaia this suggests the biological pump weakens to the point it almost stops operating at 12 millibars, getting stronger in wetter conditions above that point to sequester current level of CO2. emissions from volcanoes and infall from space whenever it goes above that point possibly reinforced by sudden floods or by rapid spread of life during the floods after runaway greenhouse effects.
Most of the time the atmosphere would be at 12 millibars or less as for the last million years. There would be occasional pulses of carbon dioxide due to volcanic eruptions, none of which were large enough to add significantly to those 12 millibars in the last million years. However from time to time Mars is far more active. When that happens, if there is a biological pump (either operating when the atmosphere is thicker or during flash floods) it could help to rapidly reduce them down to the current 12 millibars at which point the pump is so weak it almost stops operating.
Then, when Mars' axis is less tilted, the atmosphere starts to freeze out as dry ice. At that point the atmospheric pressure is regulated by the dry ice feedback rather than the Swansong Gaia.
Bierson et al find that the lowest atmospheric pressure goes down to only 2 millibars, which is reached briefly for a few millennia is only (Bierson et al, 2016, Stratigraphy and evolution of the buried CO2 deposit in the Martian south polar cap : figure 4). A couple of millennia is a is short enough period of time for life to survive in dormant states or as viable spores, however even at those low pressures, there would still be liquid water due to the solid state greenhouse effect only a few tens of centimeters below the surface of water ice at the poles if Mars does have optically translucent ice similar to the ice in Antarctica. It might also have microhabitats where salt overlays ice, and temporary microhabitats when ice boulders are thrown onto the surface after meteorite collisions, and in caves or fumaroles.
See again:
Let's look at how long it takes to remove 0.1 bars from the atmosphere of early Mars, using photosynthesis, carbonate formation, abiotic photosynthesis, etc. There is bound to be considerable uncertainty, with lower light levels, possibility of more efficient photosynthesis, possibly any water covered in ice and so on.
[WORK IN PROGRESS, DIFFICULT CALCULATION TO DO]
Pollakc et al in 1987 estimate ten million years to remove 1 bar of CO2 atmosphere in a warm wet early Mars by chemical weathering but with considerable uncertainty.They were reasonably confident the figure is not larger than a billion years (Pollack et al., 1987, The Case or a Wet, Warm Climate on Early Mars : 212).
The terrestrial pump is about 2 gigatons a year.
(Riebeek, 2011, The Carbon Cycle)
A later estimate from 2017 is that terrestrial forams remove 2.9 gigatons a year of the terrestrial carbon dioxide (Jacob et al., 2017. Planktic foraminifera form their shells via metastable carbonate phases).
There is a great deal of evidence for the shorelines of an early Martian northern ocean and Earth's ocean (Caredenas et al, 2021, Paleogeographic Reconstructions of an Ocean Margin on Mars Based on Deltaic Sedimentology at Aeolis Dorsa) (Chan et al., 2018, New Evidence of an Ancient Martian Ocean From the Global Distribution of Valley Networks)
This is an artist's impression which shows where the northern ocean would be, nestled in the vast Northern low lying plains.
(ESA, 2015, Artist's impression of Mars four billion years ago)
For a first order estimate let's just compare the areas of the oceans, assuming the early Mars oceans had a similar pump. Earth's oceans cover 360 million square kilometers (NOAA, n.d., How much of Earth's oceans have been explored). The surface area of Mars is 144.37 million km2 (Center for Planetary Sciences, An Introduction to Mars)
I can't find a surface area for the early Mars ocean [TOADD] so for now, assuming it covers a third of the surface of Mars which is about right. Then assuming the carbon sequestered by forams is proportional to the size of the ocean, we can scale the terrestrial 2.9 gigatons as 2.9*(144.37/360)*1/3 = 0.38 gigatons a year.
The Martian atmosphere is ~2.5 x 1016 kg for a total of 6.36 millibars at its mean radius (NASA, n.d. Mars Fact Sheet). So 1 bar corresponds to (1000/6.36) * 2.5 x 1016 / (103 x 109) gigatons = 3.93 million gigatons of CO2
So at 0.38 gigatons a year that comes to a period of 10 million years approximately for forams to remove 0.1 bars. similarly to Pollack's calculation for early Mars for chemical weathering. It would likely be slower than this on Mars because of the low light levels, ice, etc., but it could be higher if Mars has developed more efficient photosynthesis.
Both the forams and chemical weathering would take about a million years to remove a tenth of a bar, or 10,000 years to remove 1 millibar. However there is considerable uncertainty here.
For present day Mars, we need figures for sequestration by stromatolites and algal mats in shallow lakes that may form. Then we also need to look at sequestration of organics in sediments and in the stromatolites and algal mats as well as the carbonates.
Then we also need to look at the possibility of enhanced biological pumps after runaway warming during flash floods through sedimentary rocks and other rocks such as granite forming speleotherms.
It may well be less than 10,000 years to remove a millibar but there's too much uncertainty to calculate it.
Testing the Swansong Gaia hypothesis through looking for evidence of cycles on Mars that maintain its atmosphere near the triple point of water
If the hypothesis is correct our missions to Mars may detect life actively removing CO2, such as photosynthetic life on Mars but in low quantities so that the life is only barely detectable.
The other side of this is greater knowledge of the CO2 emissions from volcanoes and modelling of the abiotic processes that remove CO2 from the atmosphere. We might get proof that the atmosphere would be thicker without the presence of biology, again through modelling.
We could look for microfossils on Mars and study any recent carbonate deposits and organics in other ways to try to determine if they were the result of biology or abiotic processes, The percentage of carbon sequestered due to biology versus abiotic processes could be fed in to inform modelling to deduce how much of the homeostasis is due to biology and how much to abiotic processes. This would include analysing the carbonates in the dust to narrow down the estimates of how much CO2 is sequestered in them, and to determine how much is due to life processes.
From the previous section it seems that Swansong Gaia may only operate strongly to regulate the temperature rarely during flash floods, though it would continue to sequester carbon dioxide at low levels even with the atmosphere regulated at 6 millibars through the dry ice effect.
We could also look for past life on Mars. At times when the atmosphere is thicker we should find more evidence of life, and could calculate that this was sufficient to significantly accelerate the reduction of CO2 to current levels.
So, the method of confirmation would be similar to the Gaia hypothesis, we would need to find evidence sufficient to show that Martian life has been involved in a self maintaining homeostasis that constantly restores the atmospheric pressure to 6 millibars whenever it significantly departs above it.
Feasibility of terraforming Mars or ecopoesis (making Mars more habitable for native martian life)
This section continues discussions in
It uses the material from the discussion above in
Chris McKay proposes terraforming Mars using artificial greenhouse gases. He presents two possible scenarios, a Mars with a CO2 rich atmosphere with almost no oxygen and warmed with greenhouse gases for plants which he calculates can be achieved in a century, and one with a breathable atmosphere for humans which requires far longer timescales of order 100,000 years.
At the end of this video he looks at a third scenario, if what we find there is a second genesis. In this scenario we restore whatever conditions are optimal for native martian life. That relates to the scenario in this book of humans in orbit around Mars and on its moons exploring Mars via telepresence. They might not just exploit it and explore it from orbit.
Perhaps even if Mars has mirror life, and it is never safe for humans to land there and return to Earth, still, we might want to do restoration ecology, to "marsform" Mars to coin a word instead of terraform it. Make it more suitable for the current martian biosphere so that it can grow and flourish.
Video: Making Mars Habitable
The next section looks into the potential for terraforming Mars through to a breathable atmosphere, and comes up with similar timescale of around 100,000 years for an oxygen rich atmosphere, assuming we can find the carbon dioxide to convert to oxygen and enough nitrogen for a buffer - though with considerable variation around that figure, and there does seem potential to speed it up with megaengineering using millions of square kilometers of managed algal ponds which can achieve a relatively high efficiency of conversion of sunlight to biomass.:
But first let's look at the potential for a carbon dioxide rich atmosphere, perhaps mixed with oxygen, which might be more suitable for marsforming.
McKay et al and Marinova et al. proposed using greenhouse gases to warm up Mars enough for a runaway greenhouse effect (Marinova et al., 2005. Radiative-convective model of warming Mars with artificial greenhouse gases )(McKay et al., 1991, Making Mars habitable) .
However, we also found from modern radar measurements of the south pole that the total dry ice reserve available for the runaway greenhouse effect is around 12 millibars. All the dry ice at the poles evaporates at 30 degrees. Beyond that point some dry ice is liberated from the regolith but not much. Taking account of absorption in dry ice in the regolith the thickest the atmosphere gets is between 7.6 and 14.5 millibars.
Bierson et al. for instance give a figure of 12.5 millibars from (Bierson et al, 2016, Stratigraphy and evolution of the buried CO2 deposit in the Martian south polar cap : 4176). This is well below the lowest end of the range considered by McKay et al in 2001, of 100 millibars (10 kPa) for making Mars habitable for humans.
The range of estimates are from 10 to .100 kPa. Even this lower value is probably enough to move the climate state up above point B with a little assistance from other greenhouse gases, and enough for habitability with major assistance from other greenhouse gases.
(McKay et al., 2001, The Physics, Biology, and Environmental Ethics of Making Mars Habitable : 101)
it's not clear that the temperature does need to be raised to achieve a runaway. From Bierson et al (Bierson et al, 2016, Stratigraphy and evolution of the buried CO2 deposit in the Martian south polar cap : figure 4) the ice may be trapped . But Buhler et al. think the ice caps may not be much of a barrier.
If the dry ice is trapped, it may be more a matter of drilling holes in the capping layers to let the dry ice out at warmer times of the martian year at the south pole perhaps together with a little artificial warming.
Text on graphic: Mars' south pole has enough dry ice to double its atmospheric pressure to ~12 millibars but covered in a capping layer of water ice at least 10 meters thick.
We may be able to restore this atmosphere in less than a century as "ecopoesis" either by drilling through the capping layer, or by warming the dry ice with extra greenhouse gases or mirrors in space
If we restore conditions Mars had 300,000 years ago it may have dormant life from then to reawaken.
Artist’s impression by Ron Miller of the martial CO2 geysers that form in spring in the polar regions (Miller, 2006, PIA08660: Sand-Laden Jets (Artist's Concept), JPL).
That is more optimistic for terraforming or ecopoesis as far as 12.5 millibars (or whatever the figure is). It's possible that we could shift the atmosphere to 12.5 millibars today and so make it more habitable for native martian life and also marginally more useful for humans on the surface too if we find that it is safe to send human astronauts there.
So, we could liberate those 12.5 millibars , that much is maybe not that much of an obstacle with the level of access to technology that any terraformer needs for the types of projects described in the literature.
The low pressure of 12.5 millibars would mean that humans need full pressure spacesuits because our lungs can't function below the Armstrong limit of 63 millibars. So it wouldn't be enough to remove the need for spacesuits. No matter how much oxygen we get into our lungs our lungs can't absorb enough of it from the air below 63 millibars and so we quickly go unconscious. Above 63 millibars our lungs can make use of the oxygen. However we would need a closed cycle not an open cycle breathing apparatus. That is because carbon dioxide is toxic to humans at not much above current CO2 levels in the terrestrial atmosphere. We couldn't survive for long in a 63 millibars carbon dioxide atmosphere using only open cycle oxygen supplies. So 12.5 millibars compared to 63 millibars is about a pressurized spacesuit compared to a closed but unpressurized breathing system.
Liberating only 12.5 millibars of carbon dioxide available would be enough to make Mars significantly more habitable for microbes and could help restore the conditions that occurred on Mars most of the time from 300,000 to a million years ago. So that could count as restoration ecology in the sense of restoring conditions that occurred recently enough so that there may be life still there that could quickly make use of it and spread over the surface.
There might be some liquid water such as the Chinese rover found, and enough water to form gullies. See above:
Terraforming to anything like habitable conditions for trees and terrestrial plants would require much more even than McKay et al's "major assistance from other greenhouse gases" (McKay et al., 2001, The Physics, Biology, and Environmental Ethics of Making Mars Habitable : 101) to keep Mars warm since that was for an atmosphere of 100 millibars after the runaway greenhouse nd this is only 12 millibars.
Marinova et al proposed using supergreenhouse gases. These can increase the temperature much more for smaller amounts of the gases but there is an issue finding the minerals needed to make the gases. Marinova et al. found an optimal mix of 15% C2F6, 62.5% C3F8 and 22.5% SF6 (Marinova et al., 2005. Radiative-convective model of warming Mars with artificial greenhouse gases : section 5.3). 0.1 Pascals of these gases is enough to raise the temperature by 12 C (Marinova et al., 2005. Radiative-convective model of warming Mars with artificial greenhouse gases : figure 7). They proposed 0.2 Pascals to push the atmosphere up to 30 millibars which their modeling suggested was needed for a runaway greenhouse. With only 12.5 millibars available (an extra 6 millibars) these gases would be used instead to warm the planet over its current temperature.
However there may be issues locating the amounts of fluorine needed even for this modest warming by 12 C using supergreenhouse gases. The Martian atmosphere is ~2.5 x 1016 kg for a total of 6.36 millibars at its mean radius (NASA, n.d. Mars Fact Sheet). 0.1 Pascals is 0.001 millibars. Marinova et al's 0.1 Pascals corresponds to (0.001/6.36) * 2.5 x 1016 / (103 x 109) gigatons = 3.9 billion tons of the mix. I make that mix 100 * 137.75 / 171.05 = 80.5% Fluorine by weight. So, it's about 3.14 billion tons of fluorine.
For those calculations I used the atomic masses rounded to the nearest whole number: Carbon 12, Sulfur 16, Fluorine 19, Calcium 20.
The world reserves of fluorite ore are about 71 billion tons containing about 5 billion tons of fluorspar equivalent in phosphate rocks (USGS, 2022, Fluorspar). Fluorspar is CaF2 so that's 3.27 billion tons of fluorine in fluorspar ore. So at 3.14 billion tons of fluorine, it requires a little under the total fluorine in all Earth's fluorite reserves To put this in perspective, a cubic kilometer of water weighs a billion metric tons. Fluorspar is 3.18 times denser than water (Merck, n.d., fluorspar). So the world fluorspar reserves correspond around 1.6 cubic kilometers of fluorspar if refined to produce pure fluorspar, and this project needs a similar amount, a little under 1.6 cubic kilometers of fluorspar.
We don't know how much Mars has by way of fluorspar or phosphatic shales. These depend on the hydrological cycle on Earth. The fluorine is concentrated in phosphatic shales in the sea as a result of weathering, and dust falling on the sea and then the phosphorus combining with fluorine carbon and oxide as carbonate fluorapatetite (Filippelli, 2011, Phosphate rock formation and marine phosphorus geochemistry: The deep time perspective). This has the formula Ca5(PO4,CO3)3(F,O) (mindat.org, n.d., carbonate rich fluorapatetite).
From this data about how fluorite ore forms, It is likely rarer on Mars with a less active hydrological cycle and without continental drift the larger deposits would likely be mainly in the floor of the northern ocean
On the face of it as a smaller planet and with a much smaller ocean for a shorter period of time it should have less, because fluorspar and phosphatic shales (which is the main reserve of fluorine on Earth) form as a result of hydrological processes.
However it is possible to argue the other way, on Earth tectonic processes must recycle a lot of the fluorine. So that could be a reason for larger deposits in the basin of the northern sea on Mars. It would seem optimistic for Mars to have more fluorine than Earth though. 0.1 Pascals would seem to be at the high end of what might be possible if we can use all of the martian fluorine deposits. If they are similar to Earth's they'd only be a fraction of that, reasoning that it is a smaller ocean that was present for a shorter period of time and may have been ice covered for much of the time without much of a hydrological cycle.
For significant warming using fluorine based manufactured greenhouse gases, it seems likely that much of it needs to come from elsewhere, for instance the asteroid belt.
However asteroids haven't had those hydrological cycles that concentrated fluorine in the phosphatic shales. The asteroids most rich in fluorine are the EH asteroids with around 200 ppm fluorine (Kogo et al, 2018, Fluorine in the Earth and the solar system, where does it come from and can it be found? : Figure 1). At that concentration it would be necessary to mine and refine 15.7 trillion tons of the most fluorine rich asteroids to obtain the fluorine for every 0.1 pascals of warming gases for each warming by 12 C.
Such large amounts of fluorine would be more likely to be available if we can obtain it as a byproduct from a large industrial base already doing asteroid mining for other minerals. In short, unless some process on Mars has accumulated very large amounts of fluorine, it seems a megatechnology project to obtain the fluorine for the greenhouse gases for this method of terraforming, requiring large scale asteroid mining.
So, given the issues with sourcing the warming gases, and the low levels of fluorspar likely to be available, it is probably not going to be easy to terraform Mars in this way,
It may be better for prospective terraformers may need to consider other methods, for instance chemical or biological methods to return the carbonates to the atmosphere and perhaps biologically produced gases.
Methane and hydrogen sulfide are the obvious candidates for biologically produced greenhouse gases. They have a far weaker warming effect, but would be much easier to produce since they only require hydrogen, carbon and sulphur all of which are very abundant. However, they would have a short residence time in the current oxidising atmosphere. It might need creativity to find a warming gas that can be produced biologically or chemically in sufficient quantities in the current martian conditions, and that would persist in the current oxidising conditions on Mars especially since we would still want oxidising conditions for a biosphere even with plant life and trees only.
To get a thick atmosphere, and also as a warming gas, we also need a way to liberate the carbon dioxide from carbonates, perhaps using lime boring microbes as we saw in:
We don't need to worry about it freezing out rapidly as dry ice at 12 millibars at the current axial tilt, but there could be a slow dry ice sequestration enough to go from Buhler's 600 millibars to 11 millibars in 10,000 years.
Also this carbon dioxide needs to be liberated quickly enough to overcome any biological pump that may start to remove it from the atmosphere again as carbonates once the atmosphere is thick enough for a strong biological pump. Ideally we need to establish a biological pump in the opposite direction as strong as or stronger than the biological pump removing the carbon dioxide, to take the place of tectonic processes as a way to keep returning the carbon dioxide to the atmosphere indefinitely.
From all this, it seems possible that we could liberate ~12millibars by simply breaking through the capping layers of water ice at the poles or by greenhouse gases. That would be enough for partial ecopoesis.
For full ecopoesis if we want to restore the conditions of early Mars, or for terraforming, we might need to look at alternative warming gases since though the supergreenhouse gases identified by Marinova et al are very warming there is unlikely to be enough fluorspar to do the job on Mars. It might be enough to start with some lime boring microbes to liberate more carbon dioxide to thicken the atmosphere further with a modicum of planetary engineering / ecosynthesis.
There may well be enough CO2 to get all the way to above Buhler's 600 millibars and restore a habitable environment if we can liberate it from the carbonates
If we do achieve this, a sudden warming even for a few tens of millibars might well cause a miniature version of the flash floods that caused the great valley networks we see on Mars. Perhaps for as long as thousands or tens of thousands of years Mars might have rivers of water from the melting ice caps and buried ice in the mountainous regions, which might then spread to form shallow lakes in the craters, some of which might persist for a long time in the lower lying craters, especially in Hellas basin. Those would let us study directly the carbon sequestration in Swansong Gaia if it does happen. If it is biologically safe to land humans on the surface of Mars, these lakes and rivers could be of value to prospective colonists. Also it might give us an opportunity to kickstart some kind of a process to liberate carbonates into carbon dioxide and start a biological pump the other way to return carbon dioxide to the atmosphere. If humans can’t land there, the lakes and rivers would help kick-start the restoration ecology biosphere.
However, all this would need to be looked at carefully and we need always to bear in mind the potential that a mistake in deliberate ecoengineering could lead to a biosphere that is less rather than more habitable. For some examples to show the complexity of the situations see above:
Timescale to convert 130 millibars of CO2 to oxygen artificially for terraforming, millennia to tens or hundreds of thousands of years
This section continues the discussion above in
We saw that the carbon dioxide can be removed over timescales of millennia if Mars has a biological pump as efficient as Earth for sequestering carbonates. 70 ppm could be removed in 400 years if a warmer Mars had a pump as efficient as in the terrestrial oceans. It would likely take far longer however.
It may be enough of an issue so that a terraformer would need to allow for returning carbonates to the atmosphere as carbon dioxide as we discussed at the end of:
This may be a good place to work out the timescale for converting carbon dioxide to oxygen for a breathable atmosphere. Terraformers would also need to find a supply of a buffer gas most likely nitrogen which Mars m might or might not have in the form of nitrates (McKay et al., 1991, Making Mars habitable).but here we'll focus on the oxygen.
This section uses modern figures to rework the calculation of McKay et al from 1991, based on the assumption that all the organics are permanently sequestered. Normally nearly as much carbon dioxide is returned to the atmosphere as is sequestered on Earth.
McKay et al get a figure of 100,000 years to achieve a breathable atmosphere in this way (McKay et al., 1991, Making Mars habitable : 495-496). Their breathable atmosphere has a minimum of 130 millibars of oxygen (set by hypoxia) (McKay et al., 1991, Making Mars habitable : table 1).
To convert 130 millibars of carbon dioxide to oxygen, takes
If this is done artificially, algal ponds have very high productivity. A 2020 study found that 2.35 GtCO2 per year can be sequestrated as biomass in 100,000 km2 of open algal ponds (Zhoo, 2020, Macro assessment of microalgae-based CO2 sequestration: Environmental and energy effects). Much more can be sequestered in closed systems that pipe artificial light generated using LCD via light tubes into a few litres of algae.
The Martian atmosphere is ~2.5 x 1016 kg for a total of 6.36 millibars at its mean radius (NASA, n.d. Mars Fact Sheet). So 130 millibars correspond to (130/6.36) * 2.5 x 1016 / (103 x 109) gigatons = 511,000 gigatons of CO2
130
The surface area of Mars is 144.37 million km2 (Center for Planetary Sciences, n.d. An Introduction to Mars). However the polar regions would be far less habitable even in a terraformed Mars. As an approximation lets assume 50 million square kilometers in the equatorial regions are covered in algal ponds, and kept warm enough somehow to be as productive as Earth's algal ponds.
It is certainly a megaengineering project. The amount of CO2 we need to remove is about ten tons per square meter of for those fifty million square kilometers of algal ponds. Or if we can cover the whole of Mars including the polar regions, we need to remove 3.5 tons per square meter. 100 tons of biomass fixes around 183 tons of CO2 (Christie, 2007, Biodiesel from microalgae beats bioethanol : 127) . So for the fifty million square kilometers of algal ponds, we need to bury around 5.6 tons per square meter of algal dry biomass. Or just short of two tons per square meter if it can be spread over the whole surface of Mars. This gives an idea of the scale of the undertaking, we would need to cover the surface with an average of several meters thickness of dried algae in the process of converting the carbon dioxide to oxygen to get a breathable atmosphere (coal has a density of around a ton per cubic meter,and compressed algal dry biomass would be less dense than that)..
Based on 2.35 gigatons for 100,000 km2, they could sequester 500*2.35 gigatons per year, this gives a timescale a bit over four centuries (435 years) to convert 130 millibars of CO2 to oxygen.
On the other hand if we rely on nature to do it without intervention it would take too long.
The total carbon sequestered every year on Earth by land plants is around 121.7 gigatons a year, this is called the terrestrial gross primary productivity (GPP) much of which is almost immediately returned to the atmosphere through respiration. About 48 to 69 gigatons a year is sequestered as biomass (Gough, 2011, Terrestrial Primary Production: Fuel for Life) .
Most of this is returned to the atmosphere again. That is for a land area of 148.94 million square kilometers (Physicalgeography.net, n.d. Introduction to Oceans) (NEED BETTER CITE) so, that same 50 square kilometers would convert around 16 to 23 gigatons a year. Or as CO2, 59 to 85 gigatons a year.
Taking the lower value and assuming it could all be buried, and if the equatorial regions of Mars could be as productive as the average for the land area of Earth that works out at 511,000/59 = 8,666 years to convert 130 ppm of CO2 to oxygen. Those figures would be reduced because of lower light levels on Mars. But if the photosynthesis is more efficient either through engineering or using native martian life that is more efficient at photosynthesis, they would be increased.
However, it is unrealistic to bury all the plant biomass. So it would take a lot more than 8,666 years.
If we look instead at soil sequestration in various soils, then some soils lose carbon, but looking at the management practices that are reported to sequester carbon, they range from half a ton or less to over 2 tons for carbon sequestered per hectare per year (Minasny et al, 2017, Soil carbon 4 per mille : table 1) . At 100 hectares to a square kilometer that's 5 gigatons a year which gives a timescale of around 100,000 years to convert 130 ppm of CO2 to oxygen using 50 million square kilometers of well managed forestry or other systems capable of sequestering one ton per hectare, longer if less can be sequestered per year. That doesn't take account of issues of soil saturation, whether it could continue to sequester the carbon for that long.
These figures are of a similar order to McKay et al's 100,000 years. But if we could have large scale algal farms covering much of the equatorial regions of Mars and artificially buried the organics, we could have a breathable atmosphere in millennia. It could be even faster with bioreactors and artificial sunlight piped in with light-tubes. The removed carbon dioxide would need to be replaced with artificial greenhouse gases strong enough to take their place warming the biosphere.
Terraforming Mars to a carbon dioxide atmosphere suitable for plants and trees is easier, though still a major megatechnology project, if Mars has enough carbon dioxide for the project in the form of dry ice and if it can be warmed enough with artificial greenhouse gases to push it up to well above 30 ppm (McKay et al., 1991, Making Mars habitable). See:
From these calculations, it seems that a future very major megatechnology project could achieve a breathable atmosphere for humans in millennia, assuming there is enough dry ice there for photosynthesis to use to make the oxygen, or the carbon dioxide is liberated from the carbonates and assuming enough nitrogen for a buffer gas, using millions of square kilometers of algal ponds on the surface of Mars. It is far faster to achieve the same breathable atmosphere by paraterraforming or by using space colonies spinning slowly for artificial gravity. That can be achieved on a timescale of decades and with current levels of technology as we saw,
⸽ How terrestrial life that do well in Mars simulation chambers show a reciprocal plausibility of martian life able to live on Earth
[FIXCITES (HTML markup)]
We now have a wide range of candidate terrestrial microbes and even higher organisms such as lichens that could potentially survive and even grow in various candidate microhabitats on Mars. This shows that it’s biologically possible for a species of microbe or even higher life to be adapted to both planets.
()
This is not so surprising as almost all the most challenging martian conditions are duplicated somewhere on Earth in Mars analogue sites (Preston et al., 2013, CAFE-Concepts for Activities in the Field for Exploration - TN2: The Catalogue of Planetary Analogues) (Fairén et al., 2010, Astrobiology through the ages of Mars: the study of terrestrial analogues to understand the habitability of Mars). Only its ionizing radiation levels and the vacuum of space, the extreme cold, and the near vacuum of the martian atmosphere are not duplicated here, of the ones that are severe stressors,. But many terrestrial microbes turn to be pre-adapted to ionizing radiation and vacuum, probably partly because of desiccation resistance, and many go into a state of vitrification at low temperatures. Some mosses and algae can survive immersion in liquid nitrogen at -193°C and even liquid helium at only 0.65 degrees above absolute zero (Lenne et al., 2010, Freeze avoidance: a dehydrating moss gathers no ice), so would be able to survive the very low temperatures on Mars.
Some of these candidates have been tested in terrestrial Mars simulation chambers. Others have been tested in the BIOMEC experiment on the exterior of the ISS for their ability to survive in Mars simulation conditions complete with ionizing radiation. I will quote just the summary for each category from (Sielaff, et al., 2019, Habitability of Mars: How Welcoming Are the Surface and Subsurface to Life on the Red Planet?)
- “Methanogens: The results from laboratory studies show it might be possible for some methanogens to inhabit the subsurface of Mars due to their tolerance to low pressure, desiccation, and perchlorate salts.”
- “Bacteria: The described studies show that the organisms with the highest potential for survival of martian conditions are likely to be spore-forming bacteria which show resistance to multiple extreme physicochemical factors. It is important to determine if vegetative cells of spore-formers and nonspore-formers could withstand long-term simulated martian conditions. The microorganisms isolated from various Earth environments show this potential, but more research is needed on studying the limits of life for bacteria in the context of Mars habitability.”
- “Fungi: Based on the study results, the fungi studies survived exposure to simulated martian conditions in various capacities. Their resistance to radiation might be an important advantage over other microbial forms with regard to survival under martian conditions.”
- “Lichens: Although Mars would present a harsh environment for sustaining life on its surface due in part to the high amount of radiation, … an environment protective against high levels of radiation could be present on the martian surface, which may allow for the survival and proliferation of photosynthetic organisms [such as iron, salts, snows and crystalline rocks] These environments may allow for photosynthetic growth by lichens and other photosynthetic organisms on the surface, while allowing for a protective niche from the harsh environment.”
- “Mosses: These results showed that bryophytes have a high potential for survival in martian conditions, although more research is needed. Even though UV exposure did not inhibit photosynthesis completely, it would be necessary to determine if bryophytes can conduct photosynthetic activity for extended periods under these conditions.”
Some individual species or genera of special interest:
- Chroococcidiopsis - UV and radioresistant can form a single species ecosystem, and only requires CO2, sunlight and trace elements to survive (Billi, et al, 2011, Damage escape and repair in dried Chroococcidiopsis spp. from hot and cold deserts exposed to simulated space and martian conditions)
- tested in BIOMEX (De Vera et al., 2019, Limits of life and the habitability of Mars: the ESA space experiment BIOMEX on the ISS)
- sometimes found in human microbiome in the upper throat behind the nose (Ventero et al., 2022, Nasopharyngeal Microbiota as an early severity biomarker in COVID-19 hospitalised patients) and human breast milk (Lackey et al., 2019. What's normal? Microbiomes in human milk and infant feces are related to each other but vary geographically: the INSPIRE study).
- one strain Chroococcidiopsis indica produces a potential neurotoxin BMAA which can cause Lou Gerig syndrome, the disease Steven Hawking had (Cox et al., 2005, Diverse taxa of cyanobacteria produce β-N-methylamino-L-alanine, a neurotoxic amino acid : fig 2)
- Methanogenic archaea such as Methanosarcina soligelidi (Maus et al., 2020. Methanogenic archaea can produce methane in deliquescence-driven Mars analog environments) (Serôdio et al., 2014. Photophysiology of kleptoplasts : photosynthetic use of light by chloroplasts living in animal cells)
- Alkalilimnicola ehrlichii MLHE-1 (Euryarchaeota)
- able to use CO in Mars simulation conditions, in salty brines in conditions similar to those of the Recurrent Slope Lineae for the water potential and temperature range, and could grow in oxygen free conditions if nitrates are present, and unaffected by magnesium perchlorate and low atmospheric pressure (10 mbar) (King, 2015. Carbon monoxide as a metabolic energy source for extremely halophilic microbes: implications for microbial activity in Mars regolith)
- Halorubrum str. BV (Proteobacteria)
- also did well in similar conditions to Alkalilimnicola ehrlichii, simulating the RSLs (King, 2015. Carbon monoxide as a metabolic energy source for extremely halophilic microbes: implications for microbial activity in Mars regolith)
- rock inhabiting black fungi, Cryomyces antarcticus (an extremophile fungi, one of several from Antarctic dry deserts) and Knufia perforans,
- adapted and recovered metabolic activity during exposure to a simulated Mars environment for 7 days using only night time humidity of the air; no chemical signs of stress (Pacelli et al., 2017. BIOMEX experiment: ultrastructural alterations, molecular damage and survival of the fungus Cryomyces antarcticus after the experiment verification tests)
- Cryomyces antarcticus was tested in BIOMEX (De Vera et al., 2019, Limits of life and the habitability of Mars: the ESA space experiment BIOMEX on the ISS)
- Rock inhabiting black fungus Exophiala jeanselmei MA 2853
- also adapted and recovered metabolic activity during exposure to a simulated Mars environment for 7 days using only night time humidity of the air; no chemical signs of stress (Zakharova et al., 2014. Protein patterns of black fungi under simulated Mars-like conditions)
- close relatives found in the human microbiome which are occasionally pathogenic (Zakharova et al., 2014. Protein patterns of black fungi under simulated Mars-like conditions)
- occasionally an opportunistic pathogen itself (Wu et al., 2022. Cutaneous Phaeohyphomycosis of the Right Hand Caused by Exophiala jeanselmei) and close relatives are sometimes fatal (Zeng et al., 2007. Spectrum of clinically relevant Exophiala species in the United States).
- Lichens such as Xanthoria elegans, Pleopsidium chlorophanum (de Vera et al., 2014, Adaptation of an Antarctic lichen to martian niche conditions can occur within 34 days) and Rhizocarpon geographicum
- Xanthoria elegans and Rhizocarpon geographicum tested in BIOMEX (De Vera et al., 2019, Limits of life and the habitability of Mars: the ESA space experiment BIOMEX on the ISS)
- for more on Pleopsidium chlorophanum, see next section
The illustration of an alpine lichen that may be able to flourish on Mars shows the reciprocal plausibility of even multicellular martian life adapting to terrestrial conditions - Mosses Grimmia sessitana collected in the alps (Huwe et al., 2019. Mosses in low Earth orbit: implications for the limits of life and the habitability of Mars)
- tested in BIOMEX (De Vera et al., 2019, Limits of life and the habitability of Mars: the ESA space experiment BIOMEX on the ISS)
The illustration of an alpine lichen that may be able to flourish on Mars shows the reciprocal plausibility of even multicellular martian life adapting to terrestrial conditions
Not just microbes, even higher life from Mars could be adapted to live on Earth. One of our best candidates for a lichen to survive on Mars is Pleopsidium chlorophanum (gold cobblestone lichen), an alpine lichen that also grows in Antarctica in Mars analogue conditions and also grows in warmer alpine locations in places such as Europe and California.
Caption: Pleopsidium chlorophanum showing its different growing habits.
The photograph to the left shows its semi-endolithic growth in Antarctic conditions. You can see that it has fragmented the granite, and that pieces of the granite are partly covering it, possibly helping to protect from UV light. Photograph credit DLR taken at an altitude of 1492 m above sea level at "Black Ridge" in North Victoria Land, Antarctica (de Vera et al., 2014, Adaptation of an Antarctic lichen to martian niche conditions can occur within 34 days :figure 1).
The image to the right shows its more usual growing habit in California, above Lake Isabella, in the Kern River area (Sharnoff, S, 1989, Pleopsidium chlorophanum)
Summarizing details about it from (de Vera et al., 2014, Adaptation of an Antarctic lichen to martian niche conditions can occur within 34 days) this lichen is able to cope with high UV, low temperatures and dryness in cracks, probably adaptive behaviour to protect it from UV light and desiccation. It remains metabolically active in temperatures down to -20 °C, and can absorb small amounts of liquid water from the atmosphere in an environment where it is only surrounded by ice and snow. The relative humidity in the lichen's niche microhabitat varies from 57% to 79% as the temperature varies from -6 °C to -8 °C and externally it varies from 23% to 46% as the external temperature varies from 8 °C to -8 °C.
In their 34 day Mars simulation chamber experiment the temperature varied between -50 °C and +21 °C, and the relative humidity varied between 0.1% and 75% (because warmer air has lower humidity for the same water content).
The atmosphere approximates conditions encountered in the equatorial and lower attitude regions of Mars.
When exposed to full UV levels the fungus component of the lichen Pleopsidium chlorophanum died, and it wasn't clear if the algae component was still photosynthesizing,
However, when partially shaded from the UV light, as it is in its natural habitats in Antarctica, both fungus and algae survived, and the algae remained photosynthetically active throughout. Also new growth of the lichen was observed. Photosynthetic activity continued to increase for the duration of the experiment, showing that the lichen adapted to the Mars conditions.
This is remarkable as the fungus is an aerobe, growing in an atmosphere with no appreciable amount of oxygen and 95% CO2. It seems that the algae provides it with enough oxygen to survive.
The lichen was grown in Sulfatic Mars Regolith Simulant, without ice. Photosynthetic activity was strongly correlated with the beginning and the end of the simulated martian day, when atmospheric water vapour could condense on the soil and be absorbed by it, and could probably also form cold brines with the salts in the simulated martian regolith.
The pressure used for the experiment was 700 - 800 Pa, above the triple point of pure water at 600 Pa and consistent with the conditions measured by Curiosity in Gale crater.
The experimenters concluded some lichens and cyanobacteria can probably adapt to Mars conditions, taking advantage of the night time humidity, and that it is possible that life from early Mars could have adapted to these conditions and still survive today in microniches on the surface (de Vera et al., 2014, Adaptation of an Antarctic lichen to martian niche conditions can occur within 34 days).
If lichens like Pleopsidium chlorophanum on Earth can grow in Mars simulation conditions, then it’s biologically possible the other way around that any lichens on Mars may be able to grow on Earth.
So, not only microbial life, also higher life from Mars could potentially be able to colonize Earth.
Mars surface temperatures can reach 35°C in the shade in summer, which may favour optimal growth at high temperatures if life can retain water to those higher temperatures
FIXCITES
We saw that Planococcus Halocryophilus Or1 which grows in permafrost soils is also able to grow right up to human blood temperature (Mykytczuk et al., 2013. Bacterial growth at− 15 C; molecular insights from the permafrost bacterium Planococcus halocryophilus Or1). Could Martian life grow at such high temperatures?
The issue of optimal temperatures for growth is more acute for human pathogens. Any life that can live in or on the human body needs to be able to survive at or near body temperatures, although a pathogen adjusted to cooler conditions can harm us if it contaminates food or is an allergen or toxin in the air.
By definition, psychrophiles (cold loving microbes) have optimal growth at 15°C or below and psychrotrophs (cold tolerant microbes also known as psychrotolerant) are able to grow below 15°C but prefer to grow at higher temperatures, doing well at around 20°C (typical room temperature in the US) (Moyer et al., 2007. Psychrophiles and psychrotrophs).
In a likely scenario, most Martian life in Jezero crater has optimal growth temperatures at 15°C or below, psychrophiles. However this doesn’t rule out the possibility of life on Mars that has optimal growth temperatures much higher.
If martian life does have biofilms, which can retain water through to day time, there could be evolutionary pressure for species in these biofilms to acclimatize to grow rapidly for the short time it experiences very warm near surface conditions in summer, which as we saw could reach 15 °C (Martín-Torres et al., 2015. Transient liquid water and water activity at Gale crater on Mars). on the same day that surface brines have enough humidity for life at below -70°C in the early morning through to 6 am (Martín-Torres et al., 2015. Transient liquid water and water activity at Gale crater on Mars : fig 3b).
The Spirit rover measured temperatures of up to about 35°C in summer (though it had no way to detect if there were any surface brines) and down to about -90°C in winter (NASA, 2007, Extreme Planet Takes Its Toll). That is the temperature in the shade, so may be similar to the temperature experienced by any native life in the dust.
Graphic from (NASA, 2007, Extreme Planet Takes Its Toll), added the text for the maximum and minimum temperature as described in the source.
Whether or not martian life is adapted to grow at the temperatures of human blood, it may well be able to survive such temperatures.
Some species of Martian surface life may be pre-adapted to hotter, even hydrothermal conditions from geologically recent Mars and emerge by reacclimatizing, adapting, or species sorting in warmer conditions
[FIXCITES]
In another scenario, Mars has rare species of Martian surface life that inherited capabilities to adapt to warmer conditions than it encounters on the surface in Jezero crater today, for instance from species adapted to hydrothermal conditions. This commonly happens on Earth. These might emerge through species sorting – persist in small numbers in surface biofilms and spread and adapt rapidly when they encounter far warmer condition
Smith et al. list three ways microbes can adapt to warmer conditions (Smith et al., 2022).
- acclimatize by up or down regulating genes, and altering the fatty acid composition of cell walls, this can happen in minutes to days
- adapt by selection of the genetic variation a population has naturally, some combination of traits that works well at higher temperatures, or by mutation, or by recombination.
- With archaea, adaptation can also involve taking up genetic traits from other archaea through gene transfer agents, or in the other direction if they can transferring some of their capabilities to terrestrial life, which can happen overnight in seawater as we saw in the discussion of the ESF study
- Respond with species sorting – supposing we return a fragment of biofilm from Mars, it would likely include a diverse species mix and typically a terrestrial microbiome has a some rare species in it with capabilities the other microbes don’t have.
Smith et al. tested species sorting with six samples taken from a long term field experiment site, Nash’s field, in Berkshire, UK in 2016. They kept each soil sample hydrated at a constant temperature in the laboratory at 4, 10, 21, 30, 40, and 50°C for 4 weeks to simulate species sorting though they didn’t supplement them with any additional nutrients (Smith et al., 2022).
The highest temperature they tested, 50°C, is likely well above the most extreme temperatures Berkshire soils experienced in thousands of years. The UK reached 40°C for the first time in recorded history in summer 2020. According to one model, before global warming the UK likely encountered this temperature only every 2,000 to 10,000 years (Christidis et al., 2020: figure 6d).
Smith et al. took microbes from each soil sample and incubated them in agar plates until colonies formed. They found that if they incubated the sample at the same temperature it was sorted in the constant temperature phase, the new community had microbes much better adapted to the conditions. This was especially striking at 50°C.
When the soil was first incubated at 50 °C, the dominant microbes had optimal temperatures ranging from below 20 to above 35°C.
After sorting, by keeping the soil hydrated at 50°C for 4 weeks, the dominant species that emerged when incubated at 50°C had optimal temperatures ranging from below 50°C to well above 55°C (Smith et al., 2016) (reading from the diagram).
diagram).
from (Smith et al., 2016: Fig 2)
From these experiments it seems species sorting could give a community of Martian microbes a far faster way to adapt to terrestrial conditions than evolution or gene expression - provided it has at least a few rare species able to live at higher temperatures in the surface communities. It may have those from times in the past, for instance if it survived in geothermal vent refuges near the surface.
The paradox of abundant spores of heat adapted geobacillus spores in cold places on Earth, and potential for Mars to have produced such spores in the recent geological past, perhaps even today
FIXCITES
Mars had warmer conditions in the geologically relatively recent past. As recently as 210 million years ago, a volcanic eruption on the flanks of Arsia Mons melted enough ice for two lakes of 40 cubic kilometers each and a third one of 20 cubic kilometers of subsurface melt, which would have stayed melted for centuries to millennia insulated by surface ice (Scanlon, 2014, A habitable environment on Martin volcano?).
These would likely have subsurface high temperature habitable regions from volcano / ice interactions such as hydrothermal pools, and tuyas, a flat topped volcano beneath ice with liquid water forming around it (Glenister et al., 2021).
Mars has been geologically active even more recently, only the last few million years. The rootless cones (volcanic cones without a magma chamber below them) show evidence of steam explosions and hydrothermal systems that may have remained above 0°C for up to 1,300 years (Hamilton et al., 2010), with some of them possibly active as recently as less than 20 million years ago (Stacey, 2019), and there is evidence of explosive volcanism 53 to 210 thousand years ago by crater counting, which suggests some potential for present day subsurface hydrothermal activity (Horvath et al., 2021).
Mars may even have undetected hydrothermal pools in caves even today which vent to the surface from time to time, or did so in the recent past. If so, there may be rare species in surface microbial communities grow best in very warm conditions as with the microbes found by Smith et al. in their species sorting experiment. In this scenario, these thermophiles likely don’t do well in the present Martian surface conditions. They are in low numbers, outcompeted by other microbes, but they persist in small numbers, or may only persist as numerous viable spores, ready to grow when they meet the right conditions.
This exact scenario happens on Earth with the genus geobacillus. It’s been called the geobacillus paradox. These microbes do best in hydrothermal vents but they are found in surprisingly large numbers almost everywhere researchers look, including in cool soils and cool ocean floors (Zeigler, 2013). We can add more cold climate examples to Zeigler’s review, as since then, geobacillus spores have also been collected from the air over the McMurdo dry valleys in Antarctica (Bottos et al., 2014) and the soil of raised beaches in G King George Island, the largest of the South Shetland islands 120 km off the south coast of Antarctica (Zhang et al., 2018)
Geobacillus spores are probably so common because their spores are very durable. This would let them accumulate to such large numbers over long periods of time. Zeigler argues that they seem to have lifespans of at least millennia, possibly more. They are highly resistant to ionizing radiation and UV, perhaps as much so as radiodurans. They are also just the right size to get transported in the atmosphere and dust storms. It’s possible that they get released in large numbers on rare favourable conditions (Zeigler, 2013).
This seems a possible scenario for present day Mars if it has hydrothermal systems active recently, perhaps in caves or even as active fumaroles (openings that vent steam and volcanic gases), that vent to the surface, with some of the spores spreading in the dust. These vents may release large numbers of spores from time to time, but only rarely, during times of volcanic activity when the hydrothermal systems become more productive or activate. Then the spores from those occasional periods of activity could spread in the dust storms, protected from UV and ionizing radiation and accumulate in the dirt. In this scenario these spores from hydrothermal systems or fumaroles on mars could still be viable, even if they aren’t able to replicate in the current martian surface conditions.
Could there be active fumaroles even today? Fumaroles might be hard to spot on Mars as the humid air might form ice towers in colder regions of Mars, like the ice fumaroles on mount Erebus in Antarctica. These ice towers would hide most of the thermal signature. In their terrestrial analogues, life lives in dark humid caves inside the tower, with a humidity of 80% to 100%, and most microbes use chemical redox gradients for energy. Hoffmann suggested looking for circular hot spots a few degrees warmer than the surroundings and up to 100 meters in diameter. The towers would be up to 30 meters high in the low Martian gravity. The terrestrial towers often collapse and reform over a timescale of decades (Cousins et al., 2011), (Hoffmann et al., 2003).
Fumarole ice chimney on mount Erberus (Turner, 2012). Hoffmann et al. suggest its Mars analogue could be up to 30 meters high and up to 100 meters in diameter in Martian gravity and may be hard to spot because the ice would mask its thermal signature from orbit (Hoffmann et al., 2003).
Mars might have undetected fumaroles today or it may have ones that were active in the geologically recent past that might spread spores in the dust storms, similarly to the geobacillus spores on Earth. This gives a possible way for Perseverance’s samples to contain spores adapted to high temperatures in hydrothermal conditions. They might also have been produced by rootless cones (Hamilton et al., 2010) in the geologically recent past less than 20 million years ago (Stacey, 2019) or perhaps even at the times of explosive volcanism 53,000 to 210,000 years ago (Horvath et al., 2021).
In this scenario, if we return a fragment of a biofilm, say, it may have a diverse species mix just from the life best adapted to current conditions in Jezero crater, perhaps some with optimal growth temperatures well above 0°C if they can retain water through to daytime in Jezero crater.
But then, as for the field in Berkshire, there may be some that are able to do well at significantly higher temperatures than any they encounter in Jezero crater today. Those then may be like the NRC study’s example (SSB, 2009: 46) of Epsilonbacteraeota (Waite et al., 2017), found in natural hydrothermal vents, which may not be many steps away from pathogenic strains such as Helicobacter (Cornelius et al., 2012) as we saw above in
All this suggests a scenario where a martian sample might already have heat adapted strains able to grow on or in our bodies at up to human body temperature, not that different from Planococcus Halocryophilus Or1 (Mykytczuk et al., 2013), or the strains of chroococcidiopsis found in the nasopharyngeal microbiota (Ventero et al., 2022), and in human milk from Gambia (Lackey et al., 2019), also black fungi such as the pathogenic close relatives of the black fungus Exophiala jeanselmei MA 2853 which responded well to exposure to Mars simulation conditions (Zakharova et al., 2014)
⸽ A new size limit review could look at recent research into the very tiny ribocells
The next size limit review may examine new research into extremely small early life cells such as ribocells with enzymes made from fragments of RNA instead of proteins (Kun, 2021. Maintenance of Genetic Information in the First Ribocell). Steven Benner and Paul Davies say the small diameter structures in the martian meteorite ALH84001 are consistent with RNA world cells (Benner et al., 2012, Towards a Theory of Life : 37).
Panel 4 for the 1999 workshop estimated one of the structures in the Mars meteorite ALH84001 was consistent with a minimum volume early life RNA world cell 14 nm in diameter and 120 nanometers in length, if there is an efficient mechanism for packing its RNA (SSB, 1999, Size limits of very small microorganisms: proceedings of a workshop : 117). New research into ribocells might lead to a review panel to reconsider this suggestion.
A new size limit review could look at prions because of the recent discovery of prions in all three of the domains of life
[At the time of the last public comment I assumed that prions are harmless as they aren't mentioned in any of the recent Mars sample return reviews - but from my preliminary literature survey this might need to be reviewed again by experts as a result of the recent discovery of prions in all three of the domains of microbial life, most recently in the archaea. This wasn't known when prions were last assessed in the planetary protection literature in 1997]
The next size limit review might also need to look at prions. These are misfolded proteins that can cause similar proteins to misfold and so can propagate .
This a new issue raised by the Biological safety report (Craven et al., 2021, Biological safety : 6) that was previously mentioned in the 1997 report but not considered since then (SSB, 2009, Assessment of planetary protection requirements for Mars sample return missions : 60). The 1997 report said:
Subcellular disease agents, such as viruses and prions, are biologically part of their host organisms, and an extraterrestrial source of such agents is extremely unlikely.
(SSB, 1997, Mars Sample Return: Issues and Recommendations (1997) : 21)
Biological safety report said most likely Martian prion-like assemblies are harmless to Earth organisms - but that we need to be able to sterilize them - so which is it?
The Biological safety report argues in the same way that most likely Martian prions are harmless to Earth organisms, unless the terrestrial host was similar to Martian hosts and they argue that Earth is unlikely to have similar hosts
It is most likely that any hypothetical Martian prion or similar protein assembly (if such a prion exists and is present in the cached samples) would be incapable of propagation owing to the lack of available hosts, unless these protein assemblies were essentially similar to human, animal, plant or other Earth analogues, and due to the presence of differing environmental conditions on Earth.
(Craven et al., 2021, Biological safety : 4).
However they also recommend that samples are sterilized sufficiently to inactivate prions before they are released because if it can, then it can inactivate similar chemistries found on Mars
Likewise, it is not reasonable to focus other efforts specifically on the prions that cause transmissible spongiform encephalopathies such as Creutzfeldt-Jakob Disease in humans (as they are entirely based on misfolded human or animal proteins), but it is reasonable to focus on inactivation methods that affect protein assemblies similar to prions and the means of fully denaturing (inactivating) those protein polypeptides
(Craven et al., 2021, Biological safety : 11).
If it is indeed necessary to sterilize prions, or at least "protein assemblies similar to prions" to make samples safe for distribution, it may be necessary to contain prions.
So which is it, do we need to contain prions or not? Though they don't mention this in the report, and may not know about it, there is a new factor here not considered in previous reports. We now know that microbes get prion diseases too.
A potential reason to contain prions: the last universal common ancestor of modern life may have had prions and a protein from one species of bacteria can act as a prion in another
The biological safety report didn’t consider prions that infect microbes so we should look at them briefly. Yeasts often get prions and indeed most of them are beneficial (Wickner et al, 2020, How do yeast cells contend with prions? : table 1) but two of them are harmful and yeasts protect against them with various mechanisms (Wickner et al, 2020, How do yeast cells contend with prions? : figure 2). These are all ones that arise spontaneously in yeast cells. We might find yeasts on Mars especially if it has related life. Some fungi especially black yeasts do well in Mars simulation experiments (Pacelli et al., 2017, BIOMEX experiment: ultrastructural alterations, molecular damage and survival of the fungus Cryomyces antarcticus after the experiment verification tests).
Bacteria can also get prions and in a discovery from 2017, short fragments from a protein Rho from Clostridium botulinum, the microbe that causes botulism, can act as inheritable prions that change the functionality of the very distantly related bacteria E. coli. The shortest of the fragments was only 68 amino acids long (Yuan, 2017, A bacterial global regulator forms a prion).
So that also is an example of a prion that comes from an unrelated bacteria°C. botulinum infecting e. coli
Prions were also found in Archaea in 2021, which means they are now found in all three of the domains of life, Eukarya, Bacteria and Archaea and were likely present in the Last Universal Common Ancestor of all terrestrial life. (Zajkowski et al., 2020, The hunt for ancient prions: archaeal prion-like domains form amyloid-based epigenetic elements). This would seem to suggest a high chance of prions on Mars.
Prions are often beneficial to bacteria, so distantly related martian bacteria might benefit terrestrial bacteria, but would this benefit or harm the host?
The roles of prions are still being unraveled. Prions seem to be rarely harmful in bacteria and frequently beneficial and may help bacteria to cope with stress (Schramm, 2020, Protein aggregation in bacteria : conclusion). However making the bacteria better able to cope with stress could make them more infectious in hosts.
From these results, prions from an unrelated bacteria from Mars could in principle make a terrestrial microbe fitter - would that make it more harmful? The prions might be small, with an example of 68 amino acids. It might be necessary to look closer at this, to look at whether prions from Mars could
- make terrestrial microbes more infectious, or
- harm terrestrial microbes
It seems the Biological Safety Report was right to raise the issue of prions again, for the first time since 1997 and to recommend sterilization levels that would destroy them, at least until we know more. However they didn’t look at prions of microbes, a rapidly developing field. A review would need to look at what effects microbial prions could have on other organisms and the biosphere, if any.
This may very well conclude that there are no issues. However, given how small prions are and hard to sterilize, it does seem to be an issue to look at, in a thorough study of the minimum size requirements.
⸽ The life detection lab above GEO achieves no realistic risk to Earth's biosphere, so is resilient to any future change in recommended levels of assurance
Whether we decide to aim for no appreciable risk as a civilization, it's a clear benefit of any alternative that achieves it.
The ESF recommended an update of the size limit AND the level of assurance. From the comments on the PEIS and comments by Carl Sagan, Carl Woese and Gill Levin, it is clear that many in the public and scientists would support no appreciable risk rather than low risk as a level of risk assurance to aim for.
If this becomes our decision as a civilization, even most ground based telerobotic facilities would not be sufficient and the life detection lab above GEO becomes a clear winner. There may be potential for a ground based facility at this level of no appreciable risk but it would seem likely to be far more expensive at current levels of technology.
We can achieve this with any solution that sterilizes all samples returned to Earth or doesn't return them to Earth at all, and in the forward direction any solution that is able to provide thoroughly sterilized landers on Mars.
Worst case scenarios introduce novel ethical and legal questions – is a 1 in a million level of risk acceptable for a scenario that could adversely affect the biosphere of Earth in the very worst case?
The very worst case scenarios for martian life such as mirror life also introduce novel ethical and legal questions about levels of risk we are prepared to take for outcomes that could be exceptionally severe in the worst case.
The ESF settled on a 1 in a million figure. Expanding on the brief history they give, Kelly has traced the 1 in a million figure back to a 1 in 100 million figure in a 1961 article, introduced by Mantel et al. for the purpose of discussion (Mantel et al., 1961. “Safety” testing of carcinogenic agents). When asked why he chose this figure he replied "We just pulled it out of a hat" (Kelly et al., 1991. The Myth of 10^-6 as a Definition of Acceptable Risk: Or," in Hot Pursuit of Superfund's Holy Grail"). The FDA adopted this in 1973 but it became 1 in a million when the final rule was issued. Graham (Graham, 1993. Risk in perspective: The legacy of one in a million) says in practice, EPA's air office tries to reduce the risk to as many people as possible to 1 in a million and the maximally exposed individual to 1 in 10,000. In other situations, EPA recommends a range of risk levels from 1 in 100,000 to 1 in 10 million, and sometimes approves at a level of 1 in 10,000.
This is an ad hoc ethical decision by regulators about levels of acceptable risk, which got accepted more widely by legislators and the general public.
The ESF chose this figure on the basis of this historical usage, that it has become the "gold standard" although it is almost impossible to justify this as preferable to any other figure.
While it is almost impossible to find a justification for it, it appears that the 10-6 [one in a million] value has been accepted and is now considered by regulators as being the ‘gold standard’ to be met to demonstrate excellence in risk management
(Ammann et al., 2012, Mars Sample Return backward contamination–Strategic advice and requirements : 27)
It also doesn’t take account of human error. There are many examples, such as a SARS outbreak in 2003 in Taiwan which happened because a technician skipped the standard procedure after a spill, because it would make him late for a conference (Demaneuf, 2020. The Good, the Bad and the Ugly: a review of SARS Lab Escapes)
Other escapes could happen from equipment failure. During the Apollo sample returns, two technicians had to go into isolation after a leak was found in a sample handling glove for Apollo 11 (Meltzer, 2012, When Biospheres Collide : 485), and 11 technicians in a similar incident for Apollo 12 (Meltzer, 2012, When Biospheres Collide : 241).
All these issues such as the level of assurance and how to take account of human error needs especially close scrutiny once we assess a potential for novel and unprecedented large scale harm. We might also want to consider other issues such as accidents, a fire at the facility, even a plane crash into the facility, or criminal actions.
Researchers into synthetic life recommend containment requirements several orders of magnitude more stringent than a BSL-4 for their research
Synthetic biology already permits the creation of inheritable synthetic life such as life with hachimoji DNA, inheritable DNA with four extra bases for a total of 8 instead of the 4 used by terrestrial life (Hoshika et al., 2019. Hachimoji DNA and RNA: A genetic system with eight building blocks). Experimenters make sure this is safe by designing the four extra nucleotides so that they depend on chemicals only available in the laboratory.
Some synthetic biologists have suggested that a safety mechanism to contain synthetic life should be many orders of magnitude safer than any contemporary biosafety device. Schmidt puts it like this
… maybe [1 in 100,000,000,000,000,000,000] is more than enough …
The probability also needs to reflect the potential impact, in our case the establishment of an XNA ecosystem in the environment, and how threatening we believe this is.
The most important aspect, however, is that the new safety mechanism should be several orders of magnitude safer than any contemporary biosafety mechanism.
(Schmidt, 2010. Xenobiology: a new form of life as the ultimate biosafety tool)[In this quote XNA refers to life based on a different informational polymer from DNA. Similar remarks would apply to mirror life]
We can’t rely on the same risk-benefit calculus for release of SARS and for release of mirror life, without legislative / executive / public involvement to decide if this is what we should do.
Synthetic biologists are able to design the life they make with large numbers of multiply redundant precautions to make sure it can’t survive in the wild, with the precautions also designed so it can't evolve to escape the built in restrictions (Torres et al., 2016, Synthetic biology approaches to biological containment: preemptively tackling potential risks)
This is something we can do with engineered synthetic biology because we make it by modifying terrestrial life in particular ways. However any exotic life like mirror life from Mars isn't engineered by anyone to be unable to live on Earth. As we saw it may well have evolved in ways that make it capable of living on Earth.
Extraterrestrial life has the same issue as synthetic life, a potential for irreversible changes to how Earth's biosphere functions. This could lead to many in the wider scientific and general public calling for a higher level of assurance than the "gold standard" of 1 in a million, to levels of assurance similar to those we have for synthetic biology experiments.
The ESF in 2012 ruled out the prohibitory precautionary principle, because it was asked to find a way to return unsterilized samples, but a study with a broader mandate might require no appreciable risk for unprecedented harm
Finally, based on the 2012 ESF recommendation to review the size limit and level of assurance, it needs to review: new research on the potential for non terrestrial biology such as ribocells and update the size limit and level of assurance.
On that last point, updating the level of assurance, it needs to consider Carl Sagan's statement
“I, myself, would love to be involved in the first manned expedition to Mars. But an exhaustive program of unmanned biological exploration of Mars is necessary first.
“The likelihood that such pathogens exist is probably small, but we cannot take even a small risk with a billion lives.”
[quote from: (Sagan, 1973, The Cosmic Connection - an Extraterrestrial Perspective)
Based on that and extensive discussion with the general public, and ethicists, it needs to:
- examine whether or not to adopt the prohibitory version of the precautionary principle, based on wishes of the public rather than priorities of space agencies
There are many variations on this principle and the ESF study used the best available technology version. It compared two versions:
- Best Available Technology Precautionary Principle: Activities that present an uncertain potential for significant harm should be subject to best technology available requirements to minimise the risk of harm unless the proponent of the activity shows that they present no appreciable risk of harm.
- Prohibitory Precautionary Principle: Activities that present an uncertain potential for significant harm should be prohibited unless the proponent of the activity shows that they present no appreciable risk of harm
(Ammann et al., 2012, Mars Sample Return backward contamination – Strategic advice and requirements : 25)
The ESF ruled out the Prohibitory Precautionary Principle on the basis that it would simply lead to cancellation of the mission (Ammann et al., 2012, Mars Sample Return backward contamination – Strategic advice and requirements : 25)
It is not possible to demonstrate that the return of a Mars sample presents no appreciable risk of harm. Therefore, if applied, the Prohibitory Precautionary Principle approach would simply lead to the cancellation of the MSR mission.
They did this as experts mandated to find the safest way to conduct the mission. They didn’t have a mandate to recommend that the mission is cancelled.
However, elsewhere in the paper by Stewart which these experts use as their main source, he says stronger versions such as the prohibitory principle are often justified and appropriate, since society places very high value on the environment and its protection:
In critiquing strong versions of PP [the Precautionary Principle], this essay does not argue that stringent preventive environmental regulation should never be adopted. … As society places a very high value on the environment and its protection, stringent preventive regulation of uncertain environmental risks is often justified and appropriate.
(Stewart, 2022. Environmental regulatory decision making under uncertainty : 15).
NASA clarified that the Mars Sample Return mission has no campaign level probability target for containment but this is not mentioned in the text of the PEIS
NASA's employee Chester Everline put it like this:
Given that sample return missions of the type proposed for MSR have never been attempted before, is it even feasible to do enough testing to assure that a 99.9999% target can be achieved
NASA replied:
With regards to the assurance case (HS- 017), no outcome in science and engineering processes can be predicted with 100% certainty.
(NASA, 2023, Mars Sample Return FINAL PEIS : B-38)
This shows a basic lack of understanding of risk assurance. Some outcomes CAN be predicted with 100% certainty. If the samples aren't returned at all, we can predict with 100% certainty that there will be no harm to Earth's biosphere. Similarly if you sterilize all the samples returned to Earth, then with an appropriate level of sterilization we can achieve 100% certainty - or at least "no appreciable risk".
Chester Everline then raised an issue with NASA's probability target for containment. This is only for the capsule landing in the Utah sands, not for lab leaks in the Sample Receiving Facility because the PEIS had already postponed discussion of that to tier 2.
NASA doesn't set a target probability. Instead it says it will try to calculate the potential for pathogenic effects from release of small amounts of the samples.
To assess the risk associated with the return of samples, NASA has identified multiple vectors (specific pathways) that could result in the release of Mars material into Earth’s biosphere. However, a final quantitative estimate of the likelihood of release for any one vector or group of vectors based on the MSR Campaign design and mission plans is not complete, and the assessment of each of these vectors is ongoing.
Because it is currently thought the potential for pathogenic effects from the release of small amounts of Mars samples is regarded as being very low, the analysis of Health and Safety in Section 3.4 focuses on the design mitigations and protocols utilized to minimize the potential risk associated with Mars sample release during landing and recovery.
Should further refinement of mission and design elements result in the potential for substantive impacts outside the scope of those analyzed in this PEIS, then supplemental National Environmental Policy Act (NEPA) analysis may be required.
(NASA, 2023. Mars Sample Return final PEIS :3-4)
They don't define what is meant by "very low" or "substantive" in terms of a probability and don't say what level of risk they consider acceptable. Then if there is potential for a substantive impact they would just do supplemental analysis. They give no details of who is doing this analysis or what their qualifications are.
NASA applies a 1 in a million chance of containment to three different pathways by which material could escape from Mars and reach Earth. If those pathways are independent the overall probability is (1-1/million)^3 or 0.999997. That's 99.9997% containment or about one chance in 333,000 of release.
Chester Everline asked NASA to clarify what they were using, an overall target of 1 in a million or 1 in 333,000 99.9997% assurance), or some other overall target probability?
He also says that the passage is confusingly written.
Chester Everline
It is unclear, from a risk management/environmental impact perspective, what a stringent probability target is. It is explicitly stated (in the last paragraph on page S- 11 and its continuation at the top pf page S-12,), that the MSR Campaign:
- has established stringent probability targets to drive robust containment engineering
- selected a target value equivalent to a 99.9999 percent probability of successful containment
- applies these targets to each of three material vectors or pathways along which Mars material may reach Earth.
With respect to the three material vectors or pathways, if each pathway has a 99.9999 percent probability of successful containment, then the probability the MSR Campaign achieves successful containment is 0.999999 cubed or 99.9997% if the probabilities for each pathway are independent. If this is the intent it should be explicitly stated and if the intent is that the entire campaign must have at least a 99.9999 percent containment probability that should be explicitly stated.
(NASA, 2023, Mars Sample Return FINAL PEIS : B-38>)
NASA's team's reply doesn't resolve the confusion he identified, but rather, adds to the confusion:
The comment correctly identifies that the target value is applied, as stated in the PEIS, "to each of three material vectors" (pp. S-11 and 3-16) and identifies how an overall likelihood might be calculated.
The use of high probability targets for containment assurance is stringent in the sense that each serves to limit the likelihood of releasing unsterilized material, consistent with NASA's required approach, where "preventing harmful biological contamination of Earth's biosphere is the highest priority" (NPR 8715.24).
NASA allows but does not require restricted sample return missions to achieve compliance through demonstration of a specific likelihood threshold (Section 5.4.2, NASA-STD-8719.27).
To demonstrate compliance with NASA planetary protection standards the MSR Program has developed and continues to refine an Assurance Case, which provides quantitative data on the performance of the as-flown systems in terms of containment likelihood as described in the PEIS (pp. 3-16 and 3-17).(NASA, 2023. Mars Sample Return final PEIS : B-38)
So they say that
- by stringent containment they mean they applied stringent containment to each of the three pathways.
- they don't have an overall containment target.
- NASA doesn't require missions to have a specific likelihood threshold.
From this it's clear NASA is attempting risk assurance here without a specific threshold for containment for the overall mission. It is just setting containment thresholds for some particular components of the mission without any overall policy to guide them and not considering any other pathways.
NASA have made no changes to the confusing passage in their final PEIS, the passage. The first sentence is most naturally understood as a 1 in a million chance of successful containment for the entire mission.
The MSR Campaign selected a target value equivalent to a 99.9999 percent probability of successful containment. These targets are applied to each of three material vectors Mars Sample Return Campaign Programmatic EIS S-12 or pathways along which Mars material may reach Earth: 1) free particle transport; 2) approach, entry, and descent; and 3) landing.
(NASA, 2023. Mars Sample Return final PEIS : S-11 - S-12)
[It is not clear to the reader that this is just a probability target that they use for some basic events such as these three pathways without any overall campaign wide mission target]
So in short, NASA did clarify in their comment replies to Chester Everline that there is no overall likelihood target for their Mars sample return mission, however this clarification hasn't been reflected in the text of the PEIS itself.
Even though NASA have in-house expertise in risk assurance, the NASA Mars PEIS team were not able to give the responses an expert on risk assurance would expect. This is another failing of the NEPA requirement to ensure that the disciplines of the preparers were appropriate for the issues identified in the scoping process.:
The disciplines of the preparers shall be appropriate to the scope and issues identified in the scoping process
§ 1502.6
See Supplementary information under :
Also as we saw the only study to recommend an updated target is the ESF study which required 100% containment at 0.05 microns to contain ultramicrobacteria.
- Why NASA can't rely on HEPA filters for the ESF recommendation to contain 100% of ultramicrobacteria
The ESF study said the size limit and level of assurance needs to be reviewed at regular intervals and NASA certainly should have done a review of these parameters before developing a mission plan by their recommendations.
Instead NASA decided that the ESF study didn't need to be mentioned based on their assessment (not based on consulting any experts on biocontainment) that a HEPA filter can achieve 100% containment at 0.05 microns. It didn't contest the ESF recommendations and didn't say anything in response to the need for a regular review of the requirements.
JPL risk assurance expert Chester Everline's suggestion to defer returning the samples until the risk is well understood
Chester Everline mentions the idea of doing in situ studies first on Mars. This is another way to archive 100% certainty that there will be no harm to Earth's biosphere:
Thus there is a trade here, involving sending scientific instruments with limited capability to Mars - which has essentially unlimited material to sample and analyze, or returning about a half kilogram of Mars material to Earth (where there is an abundance of analytical science instruments and skilled scientists to operate them) with the understanding that ultimately more samples will likely be needed.
Even if such possibilities have already been considered and discarded as infeasible, adding a paragraph to the MSR PEIS summarizing this and citing the appropriate references would assuage concerns that the MSR PEIS has too narrow a focus.
(NASA, 2023. Mars Sample Return final PEIS : B-37)
Chester Everline said that they should rest their case on the Mars meteorite argument - which we know is false. He says if the Mars meteorite argument is not valid the risk from the Mars Sample Return mission should be considered unacceptable.
A possible consequence of unsuccessful containment is an ecological catastrophe. Although such an occurrence is unlikely, NASA should at least be clear regarding what level of risk it is willing to assume (for the biosphere of the entire planet)
…
Rigorously demonstrating compliance with a one-in-a-million loss of containment probability will be extremely challenging, and deploying such a containment system may not be technically achievable ...
…If the MSR [Mars Sample Return] Campaign can convincingly demonstrate that material returned to Earth by MSR will be subjected to more severe conditions than those transported by natural processes, then MSR poses no greater risk to Earth than we would expect from the next Mars meteorite.
[I.e. if they can convincingly demonstrate that samples are subjected to more severe conditions for life in the sample tubes than in a Mars meteorite ejected from Mars and impacting Earth]
However, if this cannot be convincingly demonstrated the MSR Campaign should seriously consider not returning samples using the technology described in the PEIS [Provisional Environmental Impact Statement] (i.e., transition to a deferred return campaign option).
[AS WE SAW THIS ARGUMENT WAS SHOWN TO BE INVALID MANY YEARS AGO (SSB, 2009, Assessment of planetary protection requirements for Mars sample return missions : 47)]A better statement of options should include the possibility of delaying the return of Mars samples until the risks associated with their return are better understood
…
If the MSR Campaign is unable to convincingly demonstrate that the risk it poses to Earth is [...] less than an analog natural hazard [i.e. Mars meteorites] the risk from MSR should be considered unacceptable. If the MSR Campaign can convincingly demonstrate the risk it poses to Earth is less than an analog natural hazard, then returning samples using MSR is analogous to having another Mars meteorite impact earth. The MSR assurance case should focus on this.(Everline, 2022, Comment posted December 20th)
[explanatory comment added in square brackets][removed redundant "not" in the red text marked as [...] ]
So, in situ study on Mars is a way to achieve zero risk while we continue to find out about Mars, and we can do life detection on Mars. It is a solution. It would safe NASA the cost of this mission in the 2020s and 2030s, replaced by in situ landers and rovers on Mars.
NASA could then return samples from Mars at a later date once we understand conditions there better. Instead of designing a way to contain them that can contain any conceivable alien biology we might find on Mars, we could then contain them based on what we know is there.
NASA's team replies that deferred entry is already covered by "no action":
Finally, sending scientific instruments with limited capability to Mars or deferring the mission is described by the No Action Alternative.
(NASA, 2023. Mars Sample Return final PEIS : B-37)
That is a mistaken reply. The "no action" alternative doesn't mention deferring the mission.
Under the No Action Alternative, the MSR Campaign as described in this PEIS would not be undertaken. As a result, investigation of Mars as a planetary system would be limited due to the cost and complexity of sending instruments into space or to Mars for in situ analyses. By not undertaking the MSR Campaign, scientists would not have access to the full breadth and depth of analytical science instruments available in Earth laboratories.
(NASA, 2023. Mars Sample Return final PEIS : 2-24)
A deferred mission alternative would say specifically that the mission is deferred until some specific criteria can be met. E.g.:
Deferred mission alternative
The mission is deferred until in situ studies on Mars establish that samples can be returned with a risk of harm of less than 1 in [target probability] and meanwhile in situ studies are prioritized
This remains a reasonable alternative. There is. a NEPA requirement to include a summary that identifies all the alternatives submitted by public commenters and Chester Everline's alterative of a deferred sample return would seem to qualify.
The final environmental impact statement shall include a summary that identifies all alternatives, information, and analyses submitted by State, Tribal, and local governments and other public commenters for consideration by the lead and cooperating agencies in developing the final environmental impact statement.
§ 1502.17
The Council on Environmental Quality also says an agency has to consider alternatives that don't fully meet all its goals.
An agency also has to look at alternatives that don't meet the applicants listed goals but better meet the policies and requirements set forth in NEPA and the agency's statutory authority and goals
(CEQ, 2022, National Environmental Policy Act Implementing Regulations Revisions - a rule by the CEQ on 4/20/2022)
It would seem that Chester's proposed alternative should have been one of those listed.
See Supplementary information under :
- Fails NEPA requirements to consider reasonable alternatives that don't fully meet the agencies listed goals but better meet NASA and NEPA policies and requirements
- NASA also required all alternatives to test unsterilized samples for present day and past life in a terrestrial laboratory based on out of date information about in situ capabilities
How we could use Carl Sagan's "we cannot take even a small risk with a billion lives" as a basis to decide to use the prohibitory version of the precautionary principle
We saw that a possible outcome of the update of the level of assurance could be a decision to adopt the prohibitory version of the precautionary principle for a Mars sample return because of the very high value that society places on the integrity of Earth's biosphere and ecosystems and on human health.
Stewart in the paper cited by the European Science Foundation suggests there may be situations where prohibition may be needed, since society places very high value on the environment and its protection (Stewart, 2022. Environmental regulatory decision making under uncertainty : 15).
The ESF said such a decision would simply lead to cancellation of the Mars Sample Return mission (Ammann et al., 2012, Mars Sample Return backward contamination – Strategic advice and requirements : 25)
It is not possible to demonstrate that the return of a Mars sample presents no appreciable risk of harm. Therefore, if applied, the Prohibitory Precautionary Principle approach would simply lead to the cancellation of the MSR mission.
See above:
However with the miniature lab above GEO suggestion we have a way to conduct the mission with no appreciable risk. Indeed we can go better. With sufficient levels of sterilization we can achieve no realistic risk.
The decision on the necessary level of assurance for a Mars sample return isn't a NASA’s decision NASA can make for themselves alone. We are all stakeholders here. Carl Sagan said we can’t take even a small risk with a billion lives
Carl Sagan:
Because of the danger of back-contamination of Earth, I firmly believe that manned landings on Mars should be postponed until the beginning of the next century, after a vigorous program of unmanned Martian exobiology and terrestrial epidemiology.
…. I, myself, would love to be involved in the first manned expedition to Mars. But an exhaustive program of unmanned biological exploration of Mars is necessary first. The likelihood that such pathogens exist is probably small, but we cannot take even a small risk with a billion lives.
(Sagan, 1973, The Cosmic Connection - an Extraterrestrial Perspective)
In the terminology of the precautionary principle, Sagan was saying that we use the prohibitory version, that we shouldn’t take even a small risk.
One possible outcome of public debate on this topic is to formalize Woese, Levin and Sagan’s ethical views on this topic into legislation. The general public, and legislators, could decide that if an action has potential for unprecedented levels of harm to human health or the environment, the prohibitory version of the principle should always be used.
Perhaps it might be formulated something like this (for illustrative purposes only not a proposal):
If it is impossible to show that there is no appreciable risk of unprecedented levels of harm to public health or the environment, the Prohibitory version of the Precautionary Principle must always be used
Unprecedented here means unprecedented in human history - e.g. the effects on our biosphere of returning mirror life able to metabolize terrestrial organics and convert ordinary into mirror organics on a large scale throughout the biosphere - or returning a novel fungal genus similar to aspergillus that human immune systems are not adapted to protect us from.
This wouldn’t be meant as an exclusive principle - there might be other situations where the prohibitory principle is appropriate. But whenever there is appreciable risk of unprecedented harm, the prohibitory version would always be used even when in the opinion of experts the level of risk is likely very low.
Ethical decisions about acceptable levels of risk can't be decided using the methods of engineering or science
This decision is something that needs global public debate.
NASA are likely to set a higher priority to completing the mission assigned to them than the general public. However they aren’t the only stakeholders. We are all potentially affected in the worst case. It needs to be opened out to larger debate.
This is something we can’t decide on the basis of science or engineering. It is an ethical and legislative choice. As Richard Randolph put it
The risk of back contamination is not zero. There is always some risk. In this case, the problem of risk - even extremely low risk - is exacerbated because the consequences of back contamination could be quite severe. Without being overly dramatic, the consequences might well include the extinction of species and the destruction of whole ecosystems. Humans could also be threatened with death or a significant decrease in life prospects
In this situation, what is an ethically acceptable level of risk, even if it is quite low? This is not a technical question for scientists and engineers. Rather it is a moral question concerning accepting risk
(Randolph, 2009, Chapter 15, God’s preferential option for life: a Christian perspective on astrobiology : 292)
Most public comments share Sagan's priority that NASA can't take even a small risk of large-scale harm -
Carl Sagan’s strong focus on protecting Earth is not an unusual concern in the general public. Several dozen distinct members of the public expressed views on NASA's draft PEIS that suggest they would be in support of a similar approach to planetary protection that places a very high value on Earth’s biosphere. 49 out of 63 made comments that make it clear they would agree with Sagan’s view. Of those, ten commenters specifically mention unprecedented harm.
These are links to seven of the nine commenters who specifically mention unprecedented harm are:
- stop mission, unprecedented harm [Richard Spotts] – test first, unprecedented harm [Evelyn B] - unprecedented harm [Logan Greger]
- stop mission, unprecedented harm [Gabor Bihari] – do not return - unprecedented harm (William Agger) - unprecedented harm, test first [Emily Whitehead]
- unprecedented harm, return to space station [Paul Marks]
Then there are the detailed comments from Thomas Dehel presenting the views of Gil Levin, (Dehel, 2022, Comment posted December 13th), DiGregario presenting the views of Carl Woes (DiGregorio, 2022, Comment posted December 5th) and the author of this book presenting the views of Carl Sagan (Walker, 2022, Comment posted December 20th).
It also shows that these were by far the most prevalent concerns of members of the public who felt concerned enough to comment on the plans. From this data it’s clear that anyone who plans a sample return mission needs to have an answer to these concerns about large-scale harm.
There were 170 comments on the first round of requests for public comments and they had a similar percentage with variations on stop mission, test first, sterilize first, with several raising unprecedented harm issues [will add more on this later]
These are links to all the comments submitted to the PEIS in the second round of public comments. The ones that would surely agree with Sagan are highlighted in bold. The link text briefly summarizes the comment.
Many are short and succinct but clear in what they intend to say.
- stop mission, unprecedented harm - test first - study in separate module attached to ISS - test first - stop mission
- stop mission - test first, unprecedented harm - test first - Study in space or not at all, keep Earth 100% safe, our efforts to contain the samples may seem lax a generation from now - need clarity about security measures
- test first keep Earth 100% safe stop mission - off topic - alternative design - stop mission, unprecedented harm - unprecedented harm
- alternative design - Test first- test first - Don’t return - ISS first
- test first - test first - test first - test first - don’t return until 100% safe
- very dangerous - test first - test first - test first- Don’t return unless 100% safe - or sterilize first test first
- unknown risk, test first- extra precautions for EES reentry - sterilize first - sterilize first - sterilize first do not return
- sterilize first do not return - sterilize in space station first - do not return - unprecedented harm - send to Russia first - test first
- issues with disinfection of earth entry site - support PEIS - study in situ or space lab or sterilize first - off topic (future missions need to be designed for reuse) - fully support, suggests more samples
- multiple cautious measures - support PEIS - support PEIS - unprecedented harm, test first - sterilize first do not return
- test in situ or don’t return - test or sterilize first - unprecedented harm, return to space station
Those are 46 comments so far that would agree with Sagan.
Three more comments were very detailed with attachments making the same point.
Thomas Dehel quoting from an interview he did with Gill Levin, principal investigator for the first and only direct life detection experiment sent to Mars, who died shortly before the start of the PEIS process
"I believe people will realize, especially after the Covid-19 catastrophe, that even if there’s only a small chance that something could be contagious and pathogenic, coming from a foreign planet, I don’t think it’s worth taking that chance….you don’t take unnecessary chances where the risk-to-benefit ratio is almost infinite.”
(Dehel, 2022, Comment posted December 13th)
Barry DiGregorio quoted from an interview he did with Carl Woese when he was alive. Carl Woese is the biologist who used gene sequencing to identify the archaea, the third realm of life. As the botanist Otto Kandler put it: “He opened a door which nobody expected to exist” (Sapp et al., 2013, The singular quest for a universal tree of life).
“When the entire biosphere hangs in the balance, it is adventuristic to the extreme to bring Martian life here. Sure, there is a chance it would do no harm; but that is not the point. Unless you can rule out the chance that it might do harm, you should not embark on such a course”
(DiGregorio, 2022, Comment posted December 5th)
Then myself, quoting Carl Sagan. This means NASA prescreened Carl Sagan's quote as nonsubstantive too.
I have left out Chester Everline’s comment as a NASA employee as he doesn’t present his personal view on the topic or the view of any other scientist. However, he would certainly count as agreeing on the potential for unprecedented harm - and on the need to protect Earth in the case of uncertain risk of unprecedented harm unless the meteorite argument can be proven.
A possible consequence of unsuccessful containment is an ecological catastrophe. Although such an occurrence is unlikely, NASA should at least be clear regarding what level of risk it is willing to assume (for the biosphere of the entire planet)
…
A better statement of options should include the possibility of delaying the return of Mars samples until the risks associated with their return are better understood
(Everline, 2022, Comment posted December 20th)
So he agrees on the potential for large-scale harm and says we should do standard risk assurance which involves setting a target probability in advance. However he doesn't give a personal opinion on what the target probability should be but just asks NASA what its target is. As we saw, NASA said they don't have a target probability.
See above:
My own final comment ends:
Let's make this an even better mission and SAFE for Earth.
(Walker, 2022, Comment posted December 20th)
Even a fully telerobotic facility couldn't easily achieve the high bar of no appreciable risk
Sketch for a future ground facility that is future proof to achieve no appreciable risk even for mirror life
There is a possible solution to all this however that doesn't even need a Mars sample return study or a size limit or level of assurance review as it satisfies even the prohibitory version of the precautionary principle and it achieves no appreciable risk for all sizes with no minimum size limit.
I sketched out a proposal for a fully telerobotic terrestrial lab which achieves even the high threshold of no appreciable risk. This also is included in the attachment 8 that NASA excluded as "nonsubstantive".
There are likely to be lower cost and simpler ways to achieve even the "no appreciable risk" level of assurance, but it gives an idea of the issues involved and one possible solution. However it is likely to be excluded on grounds of cost and it would be at TRL 0 until it is worked out into a proposal that one can evaluate and price.
Then, there are many issues with a ground-based telerobotic facility too, such as
- accidents,
- criminal damage,
- we have no experience of operating such a facility.
- How do we replace or repair equipment, and would it really prevent a lab leak?
Also, it may be a requirement that
- the complete facility can be sterilized when it’s decommissioned, for instance if we find we have returned mirror life.
Then there's the time to build it, to train people to use it and so on.
As an exercise, based on these considerations, I sketched out an idea for a telerobotic facility that would deal with all these issues at a very high level of assurance:
The aim here is simply to demonstrate that it may be technologically feasible not to minimize cost. There are many disused nuclear bunkers so that's a simple way to keep it very safe from impacts by aircraft. It would be save even in a warzone. Then it is built inside an oven for sterilization when it's decommissioned. Then I looked at a way to have access to it with no risk of forwards or backwards contamination either way. This is what I came up with, a sketch of an idea.
For the oil sump we can use Pentane X2000, often used in space applications. It has a fire point of 335°C and flash point of 315°C (the flash point is when the vapour from the oil has a risk of igniting). Density 0.85, less dense than water, vapor pressure 10-12 torr (13- 16 millibars) so it can be used in vacuum conditions with almost no loss of oil through evaporation (Venier et al., 2003)
JUST TO TRY TO ESTABLISH FEASIBLITY FOR 100% PROTECTION OR VIRTUALLY 100% FOR EARTH'S BIOSPHERE - NO ATTEMPT TO MINIMIZE COSTS.
Text on graphic: Sketch for 100% containment of mirror nanobes, etc. Sump kept at 300°C filled with Pentane X2000 oil. Both airlocks and sump continuously radiated with X-rays and ionizing radiation and sterilized with CO2 snow. Both airlocks +ve pressure, inlets sealed during airlock cycles.
Shows the LAS fully robotic floor plan for a Mars sample receiving facility placed inside an oven for end of laboratory lifetime sterilization of the facility and accessed via two airlocks and a sump for 100% containment of even mirror life nanobes.
Sketch of telerobotic facility Credit NASA / LAS (Hsu, J., 2009, Keeping Mars Contained). Photo of Cultybraggan nuclear bunker (Clark, 2009, Cultybraggan nuclear bunker)
This is just a sketch of basic scientific ideas not an engineering proposal. It look as if this could be developed into a feasible solution. The sample could be returned to Earth in a titanium sphere since those survive re-entry undamaged (Daderot, 2017, Oregon Space Ball, probably from the equipment module of Gemini 3, 4, or 5 mission, titanium), and it could be covered in a Whipple shield to protect against micrometeorite impact which might be foam based (NASA, n.d., Shield Development) and double as the shielding used for a black box flight recorder built to withstand even a plane crash (ATSB, n.d., Black box flight recorders fact sheet), only opened inside the telerobotic facility.
I cover this in the attachment to my last comment, updated version here: (Walker, 2023, NASA and ESA are likely to be legally required to sterilize Mars samples to protect the environment until proven safe).
It's hard to say how feasible or otherwise this is, as I found no research into a telerobotic facility to the standard required by the ESF sample return study - but it seems likely to be far more expensive than a small satellite launched in the early 2030s to above GEO, and needs more upfront funding and research in the 2020s.
Once we have a permanent presence on the Moon it may be a better place to build a ground facility with no appreciable risk even for mirror life
If the aim is no appreciable risk of harm to humans or Earth's biosphere, it might be simpler to build a fully telerobotic receiving facility on the Moon further into the future, though not likely to have this capability in time to build it to be ready by 2033, though with the continued miniaturizing of technology we may find we can achieve the same capabilities in an expanding miniature lab above GEO
If we find that Martian life is mirror life or in some other way so hazardous for Earth’s biosphere that it has to be contained far above a BSL-4, we may later need something larger and better resourced than a miniature telerobotic lab above GEO.
That's for a future when we have a permanent human presence on the Moon. Most likely not until the 2040s. But we have technologies that could let us fly into space with Skylon and we have the Hoyt tether system that would allow very low cost transport to / from the Moon powered by the export of materials from the Moon back to Earth. With those capabilities we'd be able to do it far easier.
It's not likely in the 2030s and the NASA team ruled it out reasonably. We may have astronauts on the Moon permanently by then but it would likely be a small station like the ISS, perhaps several modules by then in a lunar village.
Perhaps we could build an inflatable telerobotic facility for them to operate but it's hard to see what the value would be of putting it on the moon rather than adding to above GEO.
To take advantage of being on the Moon we need a large scale presence there, humans or telerobots to construct things like particle accelerators for it to use.
It would be for experiments we can't do above GEO because they are physically too large and can't be miniaturized with current technology. They have to be very large experiments given the capability to send multiple tons to above GEO.
There isn't that much of that nature for biology any more. There's sample preparation for some studies.
The miniature instruments designed for in situ study already include the sample preparation steps needed for those particular experiments.
Then there's the analysis of isotope ratios. These can be done back on Earth by returning sterilized samples using particle accelerators on Earth as we saw above in:
.It would be convenient to have an accelerator in the facility which doesn't require transport of samples back to Earth each time they need to be analysed. This assumes we still need large particle accelerators by then for ultrasensitive isotope ratio analysis and haven't miniaturized them enough to send to orbital satellites.
See above:
By then we likely have a permanent presence on the Moon. This is a decision for the future but the best solution may be a telerobotically controlled laboratory on the Moon.
Caption: Hazardous Biology Facility on the Moon, telerobotically attended, surrounded by vacuum - Artist's impression, illustration by Madhu Thangavelu and Paul DiMare © (Schrunk et al., 2007, The Moon: Resources, Future Development and Settlement : : 145, 146).
This would be a step up from the orbital satellite while keeping it 100% contained more easily than on Earth.
Humans would still go nowhere near the facility but in a future where we have a permanent human presence on the Moon we could operate such a facility far more easily than one in GEO, for instance to install new robotic handlers, to add / remove materials, to service and maintain it etc., especially if by then we need a large facility with large multi-tone instruments. We could build a particle accelerator or accelerators on the lunar surface for such a facility to use.
Why protect even microbes, mosses and lichens from another planet? Potential billions of dollars commercial value, intrinsic value like a work of art or rare species, potential to add new dimensions to the life sciences, and potential benefits of bioactive substances for health
This is about appreciating the value of a totally alien biology for science, for understanding and for our civilization generally and indeed industry. Why might we want to use 100% sterile marscopters, and eventually drones and rovers when all we are likely to find are microbes or maybe primitive lichens or mosses or some such?
If we find a second genesis on Mars it will be independently evolved from the ground up. It is highly unlikely to use exactly the same chemical structures in exactly the same ways to do the same things.
The interior of a microbe is immensely complex. These molecular visualizations by WEHI give an idea of the complexity of our genetic machinery and how it operates. The idea of including these videos is just to give an overview of how intricate it is.
Video: DNA animation (2002-2014) by Drew Berry and Etsuko Uno
And this is the cytoskeleton which is what gives the cell its support for movement and also is used as a kind of trackway for internal processes.
Video: Cell Organelles 2 Cytoskeleton
Those, and many other intricate processes are going on right now in every cell of every terrestrial organism, and the processes are almost identical in them all using the same or almost identical chemical structures.
Even if martian life is based on DNA and RNA, it surely won’t be identical in all these intricate details unless it is related.
Indeed, it may be different in
- how it copies DNA,
- how it translates it to RNA,
- how it translates RNA to proteins (if it uses proteins),
- how it activates or inactivates DNA (gene expression)
- how it corrects for errors, and so on.
Even life with a common genesis which split off from terrestrial life before the last common ancestor may be of great value for this reason. It could differ in any of these details that are identical in all modern terrestrial cells.
Its cell cytoskeleton may differ in :
- what it is constructed from
- how it lets a cell move
- how it maintains its structure.
There may be many other differences.
To take one example, one study found nearly 4,000 biologically plausible amino acids Earth life could have used. See the supplementary data section:
Does Martian life use the same amino acids as terrestrial life? Does it use the same translation table to map nucleotide triples to amino acids?
Caption: Codon table shows how RNA triplets are translated into amino acids. You read it from the centre outwards, e.g. GUG, GYA, GUC and GUU all are translated into Valine (Mouagip, 2021, Amino acids table)
This has a lot of redundancy and could in principle code up to 62 different amino acids together with a start and stop codon.
Is this particular list of twenty amino acids in some way optimal or is it a historical accident, and other life does it differently?
Might life based on a different biochemistry which originated independently from Earth life have a different codon table for the same bases and amino acids? Might it use different bases and amino acids? Might it use the same amino acids but add more, or have fewer amino acids? Or not use amino acids at all?
The interior of a living cell is so complex with so many components interacting together. Suppose we discover a second genesis of life on Mars that works on a different basis, independently evolved life on Mars, even if only microbial, even if we find just one remaining species of this second genesis that now survives only in one small habitat on Mars. This novel microbe may be as complex as terrestrial life, but so different at the atomic level, that it is as different from terrestrial life as a coral reef is from a tropical jungle at the scale of animals, plants, corals, etc. It may have been living on Mars for billions of years, a relic species from an ancient second genesis, and then it goes extinct, undiscovered, a few years after one of our missions lands nearby.
We don't have anything as radically novel as alien biology on Earth. But we do have examples of unique forms of terrestrial biology.
Let's look at one of those examples. The two Australian species of gastric-brooding frogs had a unique adaptation, the female, frog incubated its young in its stomach. Normally when we eat food this triggers production of peptins and acid to digest the food and then it triggers the stomach to empty its contents into the small intestine. But the tadpoles secreted enzymes that switched off those processes so that they could live in their mother’s stomach.
At the time, scientists started studies to find out how they did this. This might have led to new treatments for human peptic ulcers which afflict 25 million people in the USA every year, but they had to stop because the frogs went extinct (Chivian et al., 2008, Sustaining life: how human health depends on biodiversity). The frogs probably went extinct because of the imported chytrid fungus which grew on their skins and made many amphibian species extinct. There was some hope that the northern species might still exist in the wild, but an expedition in 2021 didn’t find any traces of it, though it did find other rare frogs (Groves, 2021, A last-ditch attempt to find the Eungella gastric-brooding frog in the wild fails — but could they be cloned from extinction?)
Video: Meet the Gastric Brooding Frog (Rothschild, 2017, Meet the Gastric Brooding Frog)
We can get some idea of the potential value of extraterrestrial life from the study of extremophiles which led to enzymes that are widely used (Sarmiento, 2015, Cold and hot extremozymes: industrial relevance and current trends)
- Cold adapted enzymes for detergents. These work at lower temperatures which removes the need for heating and saves energy. The global market for these enzymes is valued at $1 billion
- Enzymes and microbes that work well at lower temperatures are used in the food industry, including proving the dough at lower temperatures for bread making, to clarify fruit juices, for lactose free foods, and syrups.
- In the textile, cement, cosmetic industries (antioxidants active down to 20°C)
- Heat adapted extremophiles are used for wood pulp and paper processing, and production of glucose from starch (e.g. from maize).
- in various research techniques for experts studying DNA and RNA
They are used to reduce costs, make the processes more eco friendly, reduce CO2 emissions, enable more efficient faster processing, etc.
The cold adapted enzymes are more active, so less of the enzyme is needed, and they can be used at lower temperatures, saving energy.
The heat adapted enzymes are active and efficient at high temperatures, extreme pH values, high concentrations of the substrate, and high pressures, resist organic solvents, easier to separate during purification and catalyze faster reactions.
Discoveries from study of a novel biochemistry could be far more radical than these enzymes, with implications for pharmaceuticals, understanding processes in medicine, agriculture, nanotechnology, and many other fields. They may form materials with novel properties too (many materials we use in everyday life are the results of biology).
These lifeforms if they exist, and any discoveries that would flow from them, are as much part of our natural heritage as human beings living in our solar system as the Gastric brooding frog and any of the other myriad species that we share our planet with. The questions that arise are similar.
Given that the public highly value species such as the gastric brooding frog it’s possible they may also place high value on conservation of microbes on Mars too.
Chris McKay has discussed an approach he calls planetary ecosynthesis. If we do find a second genesis of life on Mars, biologically different from terrestrial life - it could be that the life still lingers in isolated refugia, but is headed towards future extinction.
In this scenario, we might decide not just to preserve it but to enhance conditions for growth and make Mars more habitable for Martian life, to whatever extent we can. He suggests this could greatly benefit humans, and more than outweigh any utilitarian value from attempts to make Mars itself more habitable for terrestrial life (McKay, 2009, Planetary ecosynthesis on Mars: restoration ecology and environmental ethics)
Perhaps the most interesting and challenging case is that in which Mars has, or had, life and this life represents a distinct and second genesis.
I would argue that if there is a second genesis of life on Mars, its enormous potential for practical benefit to humans in terms of knowledge should motivate us to preserve it and to enhance conditions for its growth. Observations of Mars show that currently there is no global biosphere on that planet and if life is present it is in isolated refugia or dormant. It is possible that life present on Mars today is at risk of extinction if we do not alter the Martian environment so as to enhance its global habitability.
An appreciation for the potential utility and value of the restoration of a Martian biota does not depend on the assignment of intrinsic value to alternative lifeforms. The creation of a second biosphere using a second genesis of life could be of great utilitarian value for humans in terms of the knowledge derived ranging from basic biology to global ecology. And a case can be made that its’ value exceeds the opportunity cost of not establishing human settlements on Mars.
Profitiliotis et al. did a paper on an environmental economics approach to forward contamination in 2019 which outlines four main categories of economic harm from forward contamination (Profitiliotis et al., 2019, Planetary protection issues of private endeavours in research, exploration, and human access to space: An environmental economics approach to forward contamination :3).
Their four main categories of potential harm from forwards contamination are:
- harm to in situ research by other nations by polluting and contaminating the current state of the Martian surface, also harm to other human activities that may depend on preserving the current conditions on Mars
[we could add, also future research by the nation that introduces the contamination] - endangering and disrupting pristine niches of extraterrestrial biology
- inserting hardy terrestrial microbes into in situ resources, e.g. biofouling of potential water supplies
- accidentally introduced life could interfere with future attempts to terraform Mars through deliberate introduction of useful terrestrial lifeforms.
Profitiliotis et al also did a survey of expert opinion in 2023 (Profitiliotis et al., 2023, The Future of Space Activities and Preservation on Mars: a Preliminary Policy Delphi Study). Their objective was to support discussion of whether and how to set up planetary parks on Mars protected to some degree from human contamination. They asked experts with a very wide range of expertise. The highest numbers for those who responded in all three rounds were for astrobiology (4), space ethics (3), Planetary protection (3) and Environmental management (2) with one expert each in Physics, Biology, Environmental ecology, Innovation, Philosophy, Space Law, Archaeology, History and Languages.
They used a method for strategic foresight called the Delphi method developed by RAND researchers in the 1950s, originally to forecast the effect of technology in warfare but since then applied to assist policy makers in many areas of decision making (RAND, 2023. Delphic method). This is a method used to enhance planning processes by offering enhanced foresight from experts. It promotes structured dialogue and doesn’t attempt to find a consensus. Instead any consensus emerges naturally through the dialogue.
They found a diversity of views as expected. However a consensus did emerge on some topics indicating a stable consensus. None of their experts disagreed with their statement 10 (Profitiliotis et al., 2023, The Future of Space Activities and Preservation on Mars: a Preliminary Policy Delphi Study : 19-20).
Preservation plans for Mars should include more values than just the protection of astrobiological science from biological contamination
64% strongly agreed and 29% agreed, while only 7% of the experts surveyed who responded to this statement answered with “no judgement”.
Those who agreed gave as their reasons:
- planetary protection is insufficient should there be competing national or commercial interests at Mars;
- planetary protection is time-limited by the ‘period of biological exploration’, while preservation plans are timeless;
- non-astrobiological science and environmental/resource stewardship depend on other Mars characteristics
- dimensions that need to be protected other than potential biological ones, such as planetary data sources (including rare geologic evidence), planetary resources (including water), significant physical features;
- preservation includes more values than pure science (safeguarding historical, aesthetic, and other not just scientific planetary assets);
- current understanding of what should be protected stems from various 20th century discourses which offer only a limited insight into the range of things that humans are likely to value in the future;
- Mars should be seen not just as a resource for humans but as an entity in a solar system setting that has values in its own right.
The expert who refrained from judgement gave this view:
- it is difficult to balance political and economic ideologies and approaches with cultural and scientific values.
Also none of their experts disagreed with
Inclusive broad and global dialogue among governments, private industry, scientific communities, and diverse public groups regarding preservation plans for Mars should be initiated promptly
38% strongly agreed and 54% agreed, while only 8% of the experts surveyed who responded to this statement answered with “no judgement”.
The six reasons they gave for agreement included
- broad international consensus on preservation can only be achieved by the appropriate dialogue;
- irreparable environmental damage that could occur by crewed or robotic missions must be avoided;
- there is always ongoing dialogue, but it should be further promoted, especially in a multi-stakeholder approach that includes people whose activities need to be contained rather than encouraged
Those are pioneering papers. The topic of the potentially vast commercial and non economic benefits from living organisms from a second genesis has only been considered briefly from time to time in the planetary protection literature. It hasn’t had much detailed discussion.
The public needs to know extinction of alien biology can't be reversed with current technology even from a microbe that died the day before discovery
Another topic that needs more attention is the impossibility of reversing extinction of even extraterrestrial microbes. This is something the public needs to know to inform accurate decision making.
Even we find a new form of life with what may seem a minor difference at first. It still has 20 amino acids but 10 amino acids out of 20 amino acids it uses have slight differences from terrestrial biology, and it has a new codon table. This would greatly expand the vocabulary of life. We could make new life forms by changing / introducing any of those amino acids to terrestrial life or any of the terrestrial amino acids to the novel second genesis we found on Mars. The study of exobiology would immediately become an experimental multi-dimensional discipline, with numerous other extrapolated exobiologies that we could postulate for other planets - and then attempt to synthesize in the laboratory.
Suppose we find another scenario, independently evolved life which uses exactly the same vocabulary of amino acids, and all the structures and the codon table of independently evolved life are identical to terrestrial life. That would be extraordinary and we would want to find out why. We would be eager to find out what is different if anything.
Most likely there would be many differences to study.
If the life is extinct, we could only do it by studying dead life. With no examples of living cells, it would be far harder to know how it worked when alive, its life cycle, metabolism, how it moved, how it ate food, etc. It would be similar to trying to understand the gastric brooding frog from museum specimens. De-extincting it would be like the attempt to de-extinct the gastric brooding frog but likely to be far harder.
In another scenario, we find martian life, and don’t make it extinct immediately but it can no longer compete with terrestrial life on Mars. We might be able to study living microbes for a while, but it may be hard to get it to grow. This is plausible as it is often impossible to cultivate microbes in the laboratory even for terrestrial life. Depending on how widespread native Martian life is on Mars and how rapidly terrestrial life is overcoming it, we might find ourselves in a race against time to try to rescue species of Martian microbial life before it goes extinct.
To see how hard it would be to recreate life based on alien biologies, let’s look a the example of mirror life. It may seem simple. Don’t we “just” need to put together all the chemicals that make up a terrestrial cell but in their mirror form?
First, it’s an enormous challenge to synthesize mirror images of proteins or enzymes or other biomolecules from scratch. The first step is to synthesize a mirror image of the ribosome, the protein factory that converts messenger RNA into proteins. Then we need to synthesize the mirror images of the protein based enzymes that add amino acids to the chains. We can then build up mirror message mRNA step by step with the instructions to make a new protein, and then use mirror messenger RNA together with that mirror ribosome to make mirror proteins. But it’s a difficult job (Service, R. 2022, A big step towards mirror-image ribosomes)
Then, it’s not enough to have the biochemicals. Suppose we now have the mirror RNA, mirror ribosome and mirror proteins. If we tried to make a living mirror-life cell by mixing biochemicals in a solution and filling a membrane with them, they would immediately start to react with each other as we try to build the cell. We don’t know how to make a living cell from a dead cell.
Synthetic biologists who wish to synthesize mirror life plan instead is take a new living cell and slowly transform it one step at a time, all the way from normal life to finally, hopefully, mirror life. For this to work, we need intermediate functioning cells that consist partly of mirror and partly of normal biochemicals. Remarkably the synthetic biologists think that this is possible, though it is a major challenge and may take a decade or more to complete (Bohannon, 2010, Mirror-image cells could transform science-or kill us all) (Peplow, M., 2016, Mirror-image enzyme copies looking-glass DNA),
We can’t just mix together its component chemicals, because so much depends on everything else.
Text on graphic: Assembling a dead microbe from its chemicals even one hour after extinction is far harder than assembling a watch from its parts
As soon as we start to assemble the microbe, everything reacts with everything else and the components no longer exist that we need to build it.
De-extincting a microbe of alien life is impossible in the foreseeable future. We can't even make mirror life in this way, although we know its constituents exactly.
Graphic shows Omega 17 jewel hand-wind column wheel screwed balance chronograph movement with 30-minute and 12-hour register (Lin, 2010. Omega Cal. 321 Chronograph movement)
In short the ribosomes need ribosomes to make themselves. The mRNA needs mRNA and ribosomes to make the enzymes to translate the DNA into mRNA, and so on. As soon as you start to build your cell, before it is complete, everything will start reacting and we are left with a jumble of reacted chemicals.
To make ribosomes we need mRNA which needs DNA and the translation machinery which needs enzymes made by ribosomes. Then, the ribosomes themselves are enzymes that need to be made using mRNA and other ribosomes.
In short the ribosomes need ribosomes to make themselves. The mRNA needs mRNA and ribosomes to make the enzymes to translate the DNA into mRNA, and so on. As soon as you start to build your cell, before it is complete, everything will start reacting and you are left with a jumble of reacted chemicals.
Terrestrial life must have evolved originally from chemistry. However, this likely happened through a long chain of simpler living cells as ancestors that gradually got more and more complicated. We can’t do that (though some researchers are trying to duplicate it). The only way we know to make a living cell is to start with another living cell.
So, in our scenario of newly extinct Martian life, maybe even extinct a year or even a day before, we won’t be able to de-extinct it from non viable cells.
The most we could do is modify terrestrial life step by step until we get to something that resembles martian life, just as we may do for synthetic mirror life. But depending on what martian life is like, that might not be possible, there might not be intermediate steps from terrestrial life to martian life which are all viable living cells.
For example, would there be viable intermediate cells with a PNA backbone? Or with a different vocabulary of amino acids? Or a different way of coding / decoding even the same amino acids and same DNA? Or even the much simpler ribocells, although ribocells seem biologically feasible, researchers into RNA world cells haven't found a way to make RNA cells with ribozymes from modern life. If it is possible it would be a huge challenge.
So we might never succeed, for instance if what we find has a different genetic basis. If we succeeded the result would be similar to modern attempts to de-extinct mammoths. The aim is to de-extinct mammoth genes to get a cold adapted woolly elephant (likely with red fur). It would help us to restore the mammoth steppe which would also sequester more carbon than forestry and have a lower albedo, but not provide an exact copy of the mammoth which would require a viable mammoth egg.
Church: People tend to use the shorthand that we’re De-extincting mammoths: bringing them back to life after the last of them died about 4,000 years ago. We’re not. At least, not in the near future. We’re trying to de-extinct genes. The field has actually already done this with two genes that confer cold-resistant properties to organisms. The idea is to safely introduce these and other genes into present-day elephants so the elephants can comfortably live in and restore Arctic environments.
…
Our record for multiplexed editing in general now is 22,000 edits in a few days. The number of edits we’ll need to make in the elephant isn’t clear yet. It could be as few as 40, which is the number of changes we made in the pig genome (Perry, 2019, Pig organs for human patients: A challenge fit for CRISPR) to facilitate organ transplantation to humans, and it probably won’t be more than 500,000, which is the number of fixed genetic differences between mammoths and elephants.
(Pelley et al., 2019, Siberia's Pleistocene Park: Bringing back pieces of the Ice Age to combat climate change)
In the same way in the best case we could recover some of the capabilities of martian life that we've made extinct, if it is sufficiently close to us biologically to use the insights we get from studying it to modify terrestrial life in a useful way. But it would not be the original martian life. It would be engineered terrestrial life with some chemical reactions or structures that were derived from studying dead martian microbes. We would not be likely to be able to de-extinct martian life with present day technology.
The general public need to know how major the difference is between a discovery of present day life on Mars and a discovery of newly extinct microbial life on Mars that we ourselves made extinct or that we are unable to protect against extinction. Without this information it is impossible for the public to make properly informed policy decisions. Without this information they will not be fully aware of the potential consequences of our decisions.
Arthur C. Clarke’s story “Before Eden” exploring the theme of accidental extinction of extraterrestrial life in the forwards direction
Few science fiction authors tackled the theme of forward contamination of other parts of our solar system by Earth microbes, but there's one poignant sad story, by Arthur°C. Clarke, (Clarke, "Before Eden"), published in (Goldsmith, Amazing Stories, June 1961).
Back then, though they knew Venus was hot, scientists thought it was still possible that Venus could have water on its surface, perhaps at the top of its mountains in polar regions.
This is a poignant sad story about forward contamination of Venus, published in 1961 at a time when surface life there was still a remote scientific possibility. You can hear the complete story here:
Video: Before Eden by Arthur°C Clarke
These adventurers are exploring a completely dry Venus, or so they think. Up to then (in the story), everyone thought Venus had no water, and was sterile of life. That was a natural thought, because the temperatures they encountered were always above the boiling point of water. But the heroes of the story are stranded near the not quite so hot South pole, and find mountainous cliffs there. On those mountains they find a dried up waterfall - and then - a lake!
“Yet for all this, it was a miracle—the first free water that men had ever found on Venus. Hutchins was already on his knees, almost in an attitude of prayer. But he was only collecting drops of the precious liquid to examine through his pocket microscope. … He sealed a test tube and placed it in his collecting bag, as tenderly as any prospector who had just found a nugget laced with gold. It might be - it probably was - nothing more than plain water. But it might also be a universe of unknown, living creatures on the first stage of their billion-year journey to intelligence … ”
“ … What they were watching was a dark tide, a crawling carpet, sweeping slowly but inexorably toward them over the top of the ridge. The moment of sheer, unreasoning panic lasted, mercifully, no more than a few seconds. Garfield’s first terror began to fade as soon as he recognised its cause. … ”
“… But whatever this tide might be, it was moving too slowly to be a real danger, unless it cut off their line of retreat. Hutchins was staring at it intently through their only pair of binoculars; he was the biologist, and he was holding his ground. No point in making a fool of myself, thought Jerry, by running like a scalded cat, if it isn’t necessary. ‘For heaven’s sake,’ he said at last, when the moving carpet was only a hundred yards away and Hutchins had not uttered a word or stirred a muscle. ‘What is it?’ Hutchins slowly unfroze, like a statue coming to life. ‘Sorry,’ he said. ‘I’d forgotten all about you. It’s a plant, of course. At least, I suppose we’d better call it that.’ ‘But it’s moving! ’ ‘Why should that surprise you? So do terrestrial plants. Ever seen speeded-up movies of ivy in action?’ ‘That still stays in one place - it doesn’t crawl all over the landscape.’ ”
“‘Then what about the plankton plants of the sea? They can swim when they have to.’ Jerry gave up; in any case, the approaching wonder had robbed him of words. ”“ … ‘Let’s see how it reacts to light,’ said Hutchins. He switched on his chest lamp, and the green auroral glow was instantly banished by the flood of pure white radiance. Until Man had come to this planet, no white light had ever shone upon the surface of Venus, even by day. As in the seas of Earth, there was only a green twilight, deepening slowly to utter darkness. The transformation was so stunning that neither man could check a cry of astonishment. Gone in a flash was the deep, sombre black of the thickpiled velvet carpet at their feet. Instead, as far as their lights carried, lay a blazing pattern of glorious, vivid reds, laced with streaks of gold. No Persian prince could ever have commanded so opulent a tapestry from his weavers, yet this was the accidental product of biological forces. Indeed, until they had switched on their floods, these superb colours had not even existed, and they would vanish once more when the alien light of Earth ceased to conjure them into being … ”
“ … For the first time, as they relaxed inside their tiny plastic hemisphere, the true wonder and importance of the discovery forced itself upon their minds. This world around them was no longer the same; Venus was no longer dead - it had joined Earth and Mars. For life called to life, across the gulfs of space. Everything that grew or moved upon the face of any planet was a portent, a promise that Man was not alone in this universe of blazing suns and swirling nebulae. If as yet he had found no companions with whom he could speak, that was only to be expected, for the lightyears and the ages still stretched before him, waiting to be explored. Meanwhile, he must guard and cherish the life he found, whether it be upon Earth or Mars or Venus. So Graham Hutchins, the happiest biologist in the solar system, told himself as he helped Garfield collect their refuse and seal it into a plastic disposal bag. When they deflated the tent and started on the homeward journey, there was no sign of the creature they had been examining. That was just as well; they might have been tempted to linger for more experiments, and already it was getting uncomfortably close to their deadline. No matter; in a few months they would be back with a team of assistants, far more adequately equipped and with the eyes of the world upon them. Evolution had laboured for a billion years to make this meeting possible; it could wait a little longer.”
“ … For a while nothing moved in the greenly glimmering, fog-bound landscape; it was deserted by man and crimson carpet alike. Then, flowing over the wind-carved hills, the creature reappeared. Or perhaps it was another of the same strange species; no one would ever know. It flowed past the little cairn of stones where Hutchins and Garfield had buried their wastes. And then it stopped. It was not puzzled, for it had no mind. But the chemical urges that drove it relentlessly over the polar plateau were crying: Here, here! Somewhere close at hand was the most precious of all the foods it needed - phosphorous, the element without which the spark of life could never ignite …”
" … And then it feasted, on food more concentrated than any it had ever known. It absorbed the carbohydrates and the proteins and the phosphates, the nicotine from the cigarette ends, the cellulose from the paper cups and spoons. All these it broke down and assimilated into its strange body, without difficulty and without harm. Likewise it absorbed a whole microcosm of living creatures—the bacteria and viruses which, on an older planet, had evolved into a thousand deadly strains. Though only a very few could survive in this heat and this atmosphere, they were sufficient. As the carpet crawled back to the lake, it carried contagion to all its world. Even as the Morning Star set its course for her distant home, Venus was dying. The films and photographs and specimens that Hutchins was carrying in triumph were more precious even than he knew. They were the only record that would ever exist of life’s third attempt to gain a foothold in the solar system. Beneath the clouds of Venus, the story of Creation was ended.”Video transcript starts at 12:51 into the video
How sad it would be if future explorers on Mars get glimpses of early forms of life on Mars, and they go extinct soon after they are discovered. Or indeed, even before, maybe they are extinct before anyone finds them, and all we find are traces of the signs of past life right up to the present but gone within a decade or two at the start of the Martian 4ocene (if dust storms can spread terrestrial life throughout the planet).
The precautionary principle remastered for potential super positive assets to help clarify decision making
The precautionary principle was developed to help deal with new unprecedented challenges faced by humans.
If we contaminate Mars, Europa or Enceladus with Earth life, there is no risk of harm to human health or the environment of Earth. It’s more like missing out on an unexpected gift of treasure. We risk losing a potential future benefit of great value, something we never knew we had, such as discovery of living microbes from a second genesis of life in our solar system.
The general case is as a civilization we may risk losing something of tremendous value by some course of action, but it’s something we don’t have available to us yet and we can't prove this treasure exists.
Proposed definition: a "super positive asset" is an asset which if it exists is expected to have vast positive transformative effects on us, our children and all future generations and civilizations.
For example, some alternative form of life or early life on Mars could revolutionize biology, could potentially benefit medicine, agriculture, and indeed anything that we do that uses products of life, also nanotechnology. It could potentially, in the best case scenario be a hugely positive transformative discovery.
As with the precautionary principle the public don’t need to prove that the superpositive asset exists. Rather, it is for the proponents of the activity to show that they don’t risk destroying a superpositive asset.
There might be future discoveries waiting on Mars such as XNA life (life based on a different informational polymer from DNA) or insights into the early stages of prebiotic synthesis between life and non life. If we accidentally destroy such treasures in our solar system these might be discoveries future generations will only be able to make by traveling interstellar distances (and maybe not even then if there is something special about our solar system for evolution of life).
Let’s use the text of the Wingspread consensus statement on the precautionary principle, which seems a good starting point for developing a positive variant of it (Raffensperger, 1998, The Wingspread Consensus Statement on the Precautionary Principle)
When an activity raises threats of harm to human health or the environment, precautionary measures should be taken even if some cause and effect relationships are not fully established scientifically.
In this context the proponent of an activity, rather than the public, should bear the burden of proof.
The process of applying the Precautionary Principle must be open, informed and democratic and must include potentially affected parties. It must also involve an examination of the full range of alternatives, including no action.
Let’s replace "threats of harm to human health or the environment" by "super positive asset" in the precautionary principle
When an activity raises threats of harm to human health or the environment,
To:
When an activity [impacts on a potential super positive asset that may be of overwhelming positive and transformative value for humanity]
We can rephrase the rest of it accordingly in a positive way. We get something like this:
"When an activity impacts on a potential super positive asset that may be of overwhelming positive and transformative value for humanity, precautionary measures should be taken even if some cause and effect relationships are not fully established scientifically.
"In this context the proponent of an activity, rather than the public, should bear the burden of proof.
"The process of applying the precautionary principle for potential superpositive assets must be open, informed and democratic and must include potentially affected parties. It must also involve an examination of the full range of alternatives, including no action."
As with the precautionary principle, the burden of proof is on the proponents of the activity to show that we don’t risk losing a superpositive asset.
Until we can do a biological survey of Mars, there is no way to prove Earth microbes could make Mars life extinct, or to prove they will cause no harm. Also we have no way to decide how much of a benefit such a discovery can make to scientific knowledge, commerce, human health, etc.
This would be a motive to do such a survey to decide one way or another if we do risk losing a superpositive asset on Mars.
As with the precautionary principle there can be variants, such as best technology, prohibitory and so on, depending on how much importance we assign as a society to these superpositive assets.
The main point is not so much the detailed wording of the principle. It is a recognition that we need public involvement here. We have a decision to make as a civilization about how much we value potential but not yet discovered superpositive assets, and about what level of precautions we consider are necessary to protect them.
This isn’t a decision to be made by scientists. Nor is it a decision to be made by enthusiasts for a particular activity. Rather, it is a decision that concerns all of society. It also is a decision that impacts on the legacy we leave for future generations.
⸽ As humans become more independent of Earth we may settle the only moon with a thick atmosphere, Saturn's Titan, depending on its final planetary protection classification as we learn more
Saturn’s moon Titan for one with its thick atmosphere (Wohlforth et al., 2016, Let’s Colonize Titan) (Wohlforth, 2017, Beyond Earth: Our Path to a New Home in the Planets).The Titan dragonfly mission will take seven years to reach Titan, a quadcopter drone to explore Titan searching for signs of life (NASA, n.d., NASA's Dragonfly Will Fly Around Titan Looking for Origins, Signs of Life). However, that's because it needs three flybys of Earth and one flyby of Venus to boost its delta v.
From its final flyby of Earth to Titan the Dragonfly mission takes three years and three months from 9/3/2031 to 12/30/2034 (Scott et al., 2018, Preliminary interplanetary mission design and navigation for the Dragonfly New Frontiers mission concept : 7),
So, later this century, we can expect Titan will be only a little over three years travel away once we have more powerful launch capabilities, assuming conventional rockets. It can potentially be far less with unconventional rockets. It's still a long time for passengers to spend on the cruise, but so is the six months to Mars. Typical US nuclear submarine patrols are 77 days (SubPac, n.d., Ballistic Missile Submarines)
Caption: T-LEAF inflatable aerostat exploring Titan (Ross et al., 2015, Lifting Entry and Atmospheric Flight (LEAF) Applications at Various Solar System Bodies)
It’s colder than dry ice, but this makes dry suits more insulating. Add heaters for hands and feet, and you are ready for a Titan expedition.
Text on graphic: Later humans may colonize Titan - the only other location in our solar system with an atmosphere
- in the Saturn system
- thicker atmosphere than Earth (mainly nitrogen)
Titan's atmosphere is so thick:
- humans don't need spacesuits - just very thick diving suits and bottled oxygen
- habitats are as easy to construct as a terrestrial polytunnel
- cold is far easier to protect against than vacuum
- sources of energy from winds a few hundred meters up
- gravity so low humans can fly by flapping wings!
- sources for making plastics
- ice for water, and for fuel
- so very cold backward contamination is unlikely and forward contamination impossible unless it has liquid water in cryovolcanoes
(needs confirmation that there are no planetary protection issues)
(SpaceX, 2016, Interplanetary Transport System at Saturn)
We could also use a drysuit or wetsuit in an artificial impact lake on Jupiter’s moon Callisto. Venus cloud tops are similar in temperature and pressure to Earth. All we need in principle is an acid resistant hazmat suit with rebreather to venture outside our cloud tops aerostat.
The temperatures are extremely low but this needs only thermal protection, essentially, high tech drysuits with the thermal protection only 7.5 cm thick, and batteries to heat a visor and gloves (Nott, 2009, Titan: a distant but enticing destination for human visitors), due to its greater than Earth pressure atmosphere. Thermal protection is far easier to make and maintain than protection from vacuum conditions.
Titan has abundant wind and hydro power (Hendrix et al., 2017, Energy Options for Future Humans on Titan), organics for making plastics, a stable environment and complete protection from ionizing radiation and the smaller meteorites (as for Earth). Titan’s planetary protection status needs to be confirmed but forward and backward contamination of any native Titan life seems unlikely at such low temperatures. The main contamination risk would be of flows of liquid water (cryovolcanism). If liquid water is present, it would need careful study first, as this would provide a possible habitat for Earth life as well as a habitat that could have alien life that our astronauts and Earth need protection from (Wohlforth et al., 2016, Let’s Colonize Titan) (Wohlforth, 2017, Beyond Earth: Our Path to a New Home in the Planets).
In all scenarios self sustaining settlement in space likely happens after we have easy colonization of the shallow sea floor, and floating sea cities and farms
Whether any of these are easy places to live long term may depend on the gravity requirements for human health which are not yet known. However it is not yet known if the gravity on the Moon or Mars is suitable for human health long term either. It’s possible that they all need to be supplemented with the use of slow centrifuges spinning for artificial gravity during sleep, exercise, etc.
The closest analogue may be the seabed colonies that Jacques Cousteau and others attempted to found. None lasted for long (Kaji-O'Grady et al., 2005, Prototype cities in the sea). Titan, Callisto, or the Venusian clouds, like the seabed, are still “no place like home”. Earth remains the jewel we need to protect.
We are likely to have early experiments in space similar to those seabed colonies. But the history of seabed colonies may suggest that large-scale space settlement is for a time when it is rather easier to set up colony than today. A future when it is so easy to set up a colony in such harsh situations that we would find it very easy to live either seasteading floating on the sea or below the surface of the sea using only sunlight, the atmosphere, seawater and rocks to sustain ourselves.
We are close to that point. We may well be able to live almost anywhere on Earth with greatest of ease in the near future. Living on the sea floor or at sea or on remote ice covered islands is far easier than living in any of these space colonies, including the Moon and Mars. However our first experiments in space settlement on the Moon may well lead to advances that make it far easier to live almost anywhere on Earth too and then feed back to make it easy to live almost anywhere in space.
Our first habitats in space are likely to be very limited in space, with a small room of just a few cubic meters costing more and harder to maintain than a luxury mansion on Earth. Also we need space-suits to leave the "house" which costs more than a super car and are high maintenance needing to be replaced every few weeks.
But once we can build very large space habitats such as domed cities, or colonize the vast lunar caves, the colony gets economies of scale. The maintenance of the whole habitat is very low because of the large surface are to volume ratio. Inhabitants might spend most of their time inside the caves or city or in vehicles in transport, explore the surface remotely and only need spacesuits for safety reasons. It might then be similar in level of comfort and cost to a habitat on Earth.
At this stage it would also be very easy to set up home in the middle of the Pacific, on the sea floor and almost anywhere on Earth using the same technology but without the need for:
- Containing tons per square meter of even less than Earth normal atmosphere against a vacuum or the very low pressure of the Martian atmosphere
- Environmental control to make sure carbon dioxide levels remain low, oxygen levels high and gases like hydrogen sulfide, and methane are kept under control, as we can't just open a window in space to refresh the air
- A layer meters thick of regolith covering every habitat to keep out ionizing radiation or else the occupants have an increased life-long risk of cancer
- Need for protection from meteorites and micrometeorites
- Need to wear a space-suit whenever one leaves the habitat, high cost, high maintenance at present and need for frequent replacement with current technology
- Need for protection of the habitat from small meteorites and micrometeorites, and similarly for any vehicles used to travel between habitats or explore the surroundings
- Potentially a need for artificial gravity such as a centrifuge which may be needed for health, especially important for gestation, birth, child development, early settlers may well return to Earth to give birth
These are all solvable problems and these also would benefit from the economies of scale in space habitats, but aren't issues on Earth where the Earth's atmosphere protects us against ionizing radiation, we are adapted to Earth's gravity and know the gravity levels are suitable for human health, and we have a functioning biosphere and can just open windows for fresh air.
There are benefits to space habitats too, such as:
- Weather and environment completely under control, no hurricanes or tornadoes
- No risk of volcanoes or earthquakes
In that future it would likely also be very easy to do sea steading. We might still choose to live on land. However, if we wished to, using the highly efficient agriculture of our space settlements back on Earth, we could move most of our industry and our agriculture to floating sea settlements.
Even if we can never land humans on Mars, we can colonize our solar system with self sustaining habitats slowly spinning for artificial gravity to Pluto and beyond
Finally over the centuries, and millennia, with space habitats slowly spinning for artificial gravity and large thin film mirrors to focus sunlight, we could explore and settle the entire solar system to Pluto and beyond (Johnson et al., 1977, Space settlements: A design study: 175)
“At all distances out to the orbit of Pluto and beyond, it is possible to obtain Earth-normal solar intensity with a concentrating mirror whose mass is small compared to that of the habitat.”
[in space settlements spinning slowly for artificial gravity]
“At all distances out to the orbit of Pluto and beyond, it is possible to obtain Earth-normal solar intensity with a concentrating mirror whose mass is small compared to that of the habitat.”
Space settlements: A design study (1977)
[in space settlements spinning slowly for artificial gravity]
(NASA, 2017, Catalog Page for PIA21590)
Once we have fusion power we can also inhabit the Oort cloud and since the Oort clouds of nearby stars mingle, we would be well on our way to setting the galaxy. In the far future, a forward looking civilization would live in the galaxy sustainably and protecting other civilizations and species. There is enough by way of materials in our own home star system to support trillions, quadrillions or more space settlers. Meanwhile Earth's population is projected to level off by 2100. It's the most developed countries like Japan have the lowest birth rate [NEEDS CITE]. So we can expect our space settlements to have low birth rates naturally too.
I've explored this in a preprint, not yet submitted for publication. It uses Sagan's sustainability solution to the Fermi paradox combined with planetary protection extended to galaxy protection, the idea that just as we protect planets today, an advanced civilization would not just protect planets but also the galaxy.
In that future even though at some point we might gain the technological ability to harness all the sunlight from every star in the galaxy using self replicating starships able to dismantle planets and construct a Dyson sphere or swarm around every star, we may well choose to inhabit the galaxy with efficient ultra-low energy technology and we might have large numbers of settlers only around a few stars, perhaps with a few adventurous explorers crisscrossing the galaxy, in order to protect the galaxy and ourselves - just as in the near future we likely end all deforestation of tropical rainforests. If other extraterrestrials have already done this, we would see what looks to us like a pristine galaxy (Walker, 2021, Our pale blue dot-are there other homes for us in our galaxy?)
⸽ Mars is only habitable with space suits and colonists living in pressurized habitats, never needed on Earth
Mars is not habitable to humans in any ordinary sense of the word. The atmospheric pressure of 6 to 7 millibars is well below the Armstrong limit of 6.3% where water and body fluids boil at body temperature (Murray et al., 2013. Pathophysiology, prevention, and treatment of ebullism), so we would not be able to survive there even with bottled oxygen. The atmospheric pressure on Mars is too low for our lungs to function. We would go unconscious in seconds, and require a full body pressure suit to breathe.
Suppose we had a plateau on Earth, at a height of 45 kilometers (NASA, n.d., Atmosphere), five times higher than Mount Everest at 8,848.86 km (Dwyer, 2020, Everest Gets A Growth Spurt As China, Nepal Revise Official Elevation Upward). The pressure would be typical for Mars, but it would still be far more habitable than Mars.
We could survive on such a plateau simply by raising the pressure of the air inside habitats. On Mars colonists would have to generate all their own oxygen and scrub their habitats of the CO2 (possibly using plants or algae to do this). They would need to add some other gas such as nitrogen to the cabin atmosphere, as the CO2 in the Martian atmosphere raised to terrestrial pressures is lethal above 10% leading to rapid loss of consciousness and death, irrespective of how much oxygen there is (International Volcanic Hazard Network, n.d. Carbon Dioxide (CO2))
Colonists on Mars would also need extra protection from solar storms - even on such a high plateau on Earth we would have some protection due to Earth's magnetic field as for astronauts in the ISS. We also don't know what difference the lower Martian gravity makes to long term health, and then there's half the sunlight we get on Earth.
It would be far easier to colonize such a high plateau than Mars. Yet if such a plateau occurred on Earth, we would likely have few living there permanently except to extract resources or for scientific study. We don’t colonize most deserts, or the shallow continental shelves, which are far more habitable.
So we need to terraform Mars, a centuries long process, or we can paraterraform.
Paraterraforming is where we cover over large areas of the Martian surface, for instance, cover over part of the Valles Marineris, and enclose it to make habitats to live in. This is something we can also do on the Moon with domed cities and habitats in the lunar caves or at the lunar poles. Large habitats on the Moon need to allow for the two week long night except at the poles where the peaks of sunlight have only a few days of darkness a year. However domed habitats on Mars would also need to be able to cope with dust storms that can reduce the sunlight by 99% or more and UV by 97% for a few weeks at a time. The main difference is a settlement on the Moon has to deal with predictable periods of darkness that happen either every day or for a few days a year, while the ones on Mars happen unpredictably during dust storm seasons every decade or so. Both need to function for at lest days without solar power.
Then we have the problem of the dust. This is easier to deal with on the Moon than on Mars.
Earth as a jewel of a planet and we are evolved to live here
Look at this remote island with eyes of a space colonist:
(NASA, n.d., Bouvet island from the ISS) 1,600 kilometers from the nearest land, uninhabited, except by mosses, lichens, fur seals and penguins (Norwegian Polar Institute, 2016, Bouvetøya (Bouvet Island))
It has air for plants, and to breathe! Abundant ice! Seawater! Atmosphere to protect from solar storms, cosmic rays and small meteorites! It’s a resource filled paradise.
Some feel we should set up a colony on another planet to ensure survival. Yet we have solved many problems already. Seem with eyes of a space colonist, life is so easy here.
Jeff Bezos put it like this:
"We want to go into space to save Earth. I don't like the Plan B idea that we want to go into space so we have a backup planet, … Believe me, this is the best planet. There is no doubt this is the one you want to protect. This is the jewel. We evolved here, we're kind of made for this planet. It's gorgeous, and we can use space to protect it."
Video: Jeff Bezos discusses space flight and his vision for Blue Origin
The level of technology we need to terraform Mars is more than enough to protect Earth from any suggested future natural risk including the rare risk of a far future giant comet
It is not easy to devise a scenario that could make Earth less habitable than Mars, far less habitable than Bouvet island.
- There is no risk of runaway greenhouse warming to a hot house Venus. Earth has only a tenth of the carbon based fuel reserves needed (Goldblattet al., 2013. Low simulated radiation limit for runaway greenhouse climates).
Indeed, the IPCC showed in AR6 / WG1 that we can't have even a short term warming tipping point, virtually certain:“It is virtually certain that the net climate feedback is negative” (IPCC AR6 / WGI, 2021, Chapter 7: The Earth’s Energy Budget, Climate Feedbacks, and Climate Sensitivity : 73). Extra heat radiated away is counted as -ve feedback as it acts to prevent runaway. As the Earth warms it radiates away more heat (just as a warm radiator radiates away more heat). More heat is also trapped and more heat is absorbed but with each degree of warming far more heat is radiated away than absorbed or trapped. They found a total of -1.16 watts lost with each degree of warming, range -0.51 to -0.81 making it virtually certain we can't have a warming tipping point even taking account of all possible contributing factors and uncertainties. - Supernovae and gamma ray bursts are only possible for rare types of star. We know our neighbourhood well now. They can’t happen for thousands of years (Plait, 2010, No, a nearby supernova won't wipe us out - Bad Astronomy) (Gowanlock., 2016. Astrobiological effects of gamma-ray bursts in the Milky Way galaxy). Moreover humans on Earth have extra protection of the atmosphere equivalent to 10 meters of shielding by water, which is likely far more than for any potential Mars colony at least for surface domed cities.
Even the asteroid winter that ended the dinosaur era left Earth far more habitable than Mars is today and that is no longer a possible scenario for NEOs.
- Asteroids and comet NEOs - we now have a complete survey of the Near Earth objects of 10 km and larger, similar in size to the Chicxulub impact that ended the dinosaur era, we have found only four comets and four asteroids, none able to hit us in less than thousands of years. As of 2017 the largest size bin with an estimate of one asteroid left to find is 3.5 km (Stokes et al., 2017, Update to determine the feasibility of enhancing the search and characterization of NEOs : 26 and Table 2.1). So there is no risk for a NEO asteroid winter like the one that ended the dinosaur era. The lists are also almost complete down to one kilometer, a size of asteroid that hits only every few hundred thousand years and we will soon find almost all of the few dozen remaining.
The risk from long period comets is very remote.
- Long period comets - the risk is far lower than for NEOs at only 0.3% of the objects of 0.9 km and upwards (Stokes et al., 2017, Update to determine the feasibility of enhancing the search and characterization of NEOs : 14)
Based on the current population of 8 NEOs of 10 km and larger and the calculation method of (Stokes et al., 2017, Update to determine the feasibility of enhancing the search and characterization of NEOs : ii) we are hit by a 10 km NEO, very approximately, every 62.5 million years (500 million / 8). That's also our expected wait time since the expected distribution is memoryless. The past history of when we were last hit by such an asteroid is independent of our current risk.
Using a long period comet population of 0.3% of the NEO population, this makes the expected wait time for a long period comet of that size around 20 billion years (62.5 million * 100 / 0.3) with the current population of comets. There may be more comets after breakup of a Centaur (a large icy object orbiting between Jupiter and Neptune), or 1.3 million years from now during the flyby of Gliese 710 (Marcos et al., 2020, An Update on the Future Flyby of Gliese 710 to the Solar System Using Gaia EDR3: Slightly Closer and a Tad Later than Previous Estimates) but there is nothing in the next few millennia to increase the flux.
We do also have ways to divert at least small to medium sized comets with near future technology. The Breakthrough Starshot project plans to construct large multi-gigawatt lasers to propel tiny microspacehips to distant stars using thin film solar sail type materials. Philip Lubin looked into using these to deflect comets. He found that, if you spot a 5 km diameter comet two years before impact, it is easy to deflect it with a 100 gigawatt laser array with a few minutes a day if you have an orbital laser array - or else if you have arrays in both hemispheres on Earth. Most can be deflected by more than thirty times the diameter of Earth which is enough to deal with uncertainties in the path of the comet due to its own jets from the sun (Lubin, 2017, Comet deflection by directed energy: a finite element analysis) and (Zhang, 2017, Orbital simulations on deflecting near-earth objects by directed energy)
With the level of investment needed for terraforming Mars we can likely find ways to improve on those figures.
We don't know that it is possible to terraform Mars with near future technology. In a paper from 1991, McKay et al said:
Mars is believed to be lifeless, but it may be possible to transform it into a planet suitable for habitation by plants, and conceivably humans. The success of such an enterprise would depend on the abundance, distribution and form of materials on the planet that could provide carbon dioxide, water and nitrogen.
(McKay et al., 1991, Making Mars habitable)
So far we haven't found those large amounts of carbonates or nitrates (Jakosky et al., 2018. Inventory of CO2 available for terraforming Mars)
Wired reports Jakosky as saying:
“We were able to put together for the first time a reasonably clean inventory of the CO2 on Mars,. The bulk has been lost to space, a small amount to polar ice and shallow carbon-bearing minerals, and an unknown amount to deep carbonates.” Even adding in bits of CO2 stuck onto rocks—“adsorbed” onto their surfaces—and a little more locked into water-molecule cages called clathrates doesn’t help. “Even if you put it all back into the atmosphere, it doesn’t add up to enough to warm the planet,”
(Rogers, 2018, Sorry, Nerds: Terraforming Might Not Work on Mars)
Chris McKay finds the data inconclusive:
“The key question for terraforming is the amount of CO2, N2, and H2O on Mars. Unfortunately there is nothing new here to resolve this question,” McKay emails. Jakosky’s Maven results only show some of Mars’ ex-carbon dioxide leaving, not all of it. So maybe it’s still there, McKay says. “We are still highly uncertain as to the amount of CO2 below the surface. We don’t have good data and we need to drill deeply to get it.”
(Rogers, 2018, Sorry, Nerds: Terraforming Might Not Work on Mars)
NASA reports that the same team found that it isn't practical to supplement the atmosphere using redirected asteroids and comets.
Another idea is to import volatiles by redirecting comets and asteroids to hit Mars. However, the team’s calculations reveal that many thousands would be required; again, not very practical.
(NASA, n.d., Mars Terraforming Not Possible Using Present-Day Technology)
We may be able to solve all these problems and terraform Mars. But if we develop the high level of technology needed to terraform Mars, we most likely don't have to rely on a terrestrial "planet b" to save humanity. We can protect Earth directly.
We also have many ways to establish a presence elsewhere in the solar system and to create a backup of seeds, technology, knowledge in case there is harm to the backup seed vaults, libraries and so on that we have on Earth itself, or as time capsules for future terrestrial civilizations to find.
Moon as a backup, closer to Earth and able to preserve seeds at liquid nitrogen temperatures at the poles
Since there is no realistic possibility of a disaster to make Earth as uninhabitable as Mars, the purpose of a backup should be to restore the Earth not to move to another planet. This makes the Moon by far the best place to backup seeds.The moon's axis is only slightly tilted unlike Earth's and it has large areas of permanent darkness in the polar craters which are passively cooled to liquid nitrogen temperatures - many seeds would last centuries there without any attention stored a few meters below the surface.
William Burroughs and others proposed a seed bank on the Moon (Burrows, W., 2004, The survival imperative: using space to protect Earth). The eternal dark of polar craters is passively cooled to liquid nitrogen temperatures continuously below 110 K (-163°C ) (Hayne et al., 2015. Evidence for exposed water ice in the Moon’s south polar regions from Lunar Reconnaissance Orbiter ultraviolet albedo and temperature measurements). This increases lifetimes of many seeds tenfold (Walters et al., 2004. Longevity of cryogenically stored seeds). A time vault of life, it would be immune to political upheavals, climate change, all manner of disasters, and be there a thousand years later if needed.
Mars can't provide the same stable cold conditions as the lunar poles. The polar regions are cold in winter but those temperatures are seasonal as for Earth. Also the closer the backup is to Earth the more accessible it is.
Burroughs et al also suggest a backup library of digitized knowledge interrogated via radio. We could add librarians, who oversee the seed bank and grow plants such as mangoes, whose seeds can’t be cryopreserved.
We have also created many new sub-species of flowers, plants, animals, rare breeds of sheep, cows, fruit trees, etc. There is now a major conservation program of saving rare cultivated varieties of these things. That's the main focus of the Svalbard seed vault. The millennium seed bank in Surrey maintained by Kew Gardens preserves wild seeds (Kimbrough, 2020, Through war, wildfire and pandemic, the world’s seed vaults hold strong)
There are many seed banks in individual countries. These are backups of the seedbanks which in turn are backups of the agricultural and wild seeds. This would be an extra level, a backup in space which can be left passively cooled with no maintenance even for centuries.
Most plants can be preserved as seeds. There are exceptions, the "Exceptional species" which either don't produce seeds and need to be produced by propagation, or produce seeds rarely or that produce seeds that are hard to preserve (Zhang et al., 2021, Defining exceptional species—A conceptual framework to expand and advance ex situ conservation of plant diversity beyond conventional seed banking)
. A fair number of tropical fruits like mangoes can't be preserved in seed banks - the only way to preserve mangoes at present is to propagate them every year. They are propagated by grafts or from seeds depending on the type of mango (Slaven, n.d., Propagating mangoes). But mango seeds lose their viability a few days after they are removed from the fruit and don't survive long in the fruit either. There is some success using liquid nitrogen for some recalictrant seeds. (Walters et al., 2013, Preservation of Recalcitrant Seeds)
So there are exceptions but most of the world's crops and most of the wild plants outside of tropical rainforests can be preserved for a long time in seed vaults - many decades to centuries.
For the ecosystems themselves, later we can back those too, in lunar lava tube caves. In the low gravity theoretically these tubes are stable at up to five kilometers in diameter (Blair et al., 2017. The structural stability of lunar lava tubes) and by radar observations, perhaps over 100 km long (Sood et al., 2016. Detection of buried empty lunar lava tubes using GRAIL gravity data). We can do the same eventually in space habitats too, simulating any climate desired, and these could also backup extraterrestrial biologies from Mars, Europa, Enceladus, etc.,, spinning for artificial gravity to simulate the conditions on any planet or moon. This is technology that though advanced for us is far less than the level required for terraforming a planet.
⸽ How NASA's current PEIS fails many central NEPA requirements
This section summarizes the main issues mentioned in the paper. This book finds that NASA's PEIS (Programmatic Environmental Impact Statement) fails many central NEPA requirements. The issues aren't subtle gray area issues for lawyers. They are clear violations of many of the most central requirements of NEPA.
The main issues identified are that it:
- fails scientific integrity
- disciplines of preparers doesn't match the task
- doesn't identify the consensus opposing view to its Mars meteorite argument and its other three main planetary protection arguments
- doesn't discuss the majority view, also a consensus, that potential low risk worst case effects are global impacts on public health and environment
- narrow prescreening that omits alternatives if they fail to meet all the requirements of the mission plans
It seem appropriate to touch on theses issues briefly here then look at one way to resolve them all using the proposed change to NASA's plans in this book to return the samples to a miniature life detection lab above GEO.
All these issues potentially make NASA's plans vulnerable to legal action if anyone took them to the courts.
However they also deserve attention because there are reasons for these NEPA rules. They help protect the public and the agency itself from potentially harmful consequences. If an agency doesn't fulfill the requirements it doesn't just open it up to legal challenges in the present. It can also lead to real life issues in the future based on the reasons for the existence of the rules in the first place.
This section is not intended to support any legal case. Rather it is to reinforce the need for independent review of NASA's PEIS by an organization of the highest integrity, probably the Space Studies Board and National Academy of Sciences in the USA or the European Science Foundation in Europe, or both. It is also to reinforce the need to engage the public and scientists outside of NASA and encourage thorough scrutiny of NASA's plans from experts in the relevant disciplines globally.
Also this section might become a motivation for NASA to withdraw the PEIS voluntarily from their side if they agree that they have not complied with NEPA requirements as they thought they had.
Fails NEPA requirement for scientific integrity: for instance, should have said that the main cite for its Mars meteorite argument rebuts it
NEPA requires agencies to ensure scientific integrity.
"Agencies shall ensure the professional integrity, including scientific integrity, of the discussions and analyses in environmental documents"
§ 1502.23
NASA depend on the Mars meteorite argument but it is rebutted by its main cite.
NASA:
First, potential Mars microbes would be expected to survive ejection forces and pressure (National Academies of Sciences, Engineering, and Medicine and the European Science Foundation 2019) [CITE REBUTS SENTENCE], …”
(NASA, 2023. Mars Sample Return final PEIS : 3-3)
The cite:
National Academy of Sciences, 2019 (NASA's cite)The microbes may NOT be able to survive impact ejection and transport through space.”
(SSB, 2019, Planetary protection classification of sample return missions from the Martian moons : 45)
The issue of scientific integrity here is that NASA doesn't alert the reader to this discrepancy or discuss it. Indeed it nowhere mentions that any source has ever rebutted the Mars meteorite argument.
details see above:
Fails NEPA requirement for scientific integrity for the other three apparently plausible but invalid arguments
It is a similar situation for the other three invalid arguments. All fail scientific integrity. As we saw,
- NASA's argument that Mars is uninhabitable is rebutted by NASA's own plans to test the samples to see if they can get anything to grow from them.
See above: NASA's uninhabitable Mars finding rebutted by its own cite, and by NASA's own plans to test for life in its samples
The primary issue of integrity here is that NASA doesn't mention its plans to try to get martian life to grow from the samples in the PEIS. A subsidiary issue is that on the face of it, it seems impossible to reconcile the two statements which seem contradictory.
- near zero risk of terrestrial pathogens from Mars
see above: NASA's Biological Safety Report's finding of near zero risk that martian life can harm us rebutted by soil pathogens like tetanus and aspergillus fumigatus not adapted to any terrestrial host
Here the issue with scientific integrity is an inadequate literature search which would quickly turn up examples of human pathogens not adapted to an infectious lifestyle in any host.
- argument that a martian lifeform would not be able to live on Earth
See above: NASA's Biological Safety Report's finding that any martian microbe would not be viable on Earth rebutted by the only plausible Mars analogue in its cite: Planococcus Halocryophilus
Here the issue of scientific integrity is that the study didn't discuss the example organism in NASA's own cite which seems to provide a counterexample to their reasoning, or the many terrestrial organisms that have been tested in Mars simulation chambers.
Also NASA's team's literature search didn't turn up the rebuttal of all four of their main arguments by their first planetary protection officer in 2000 (it is inconceivable that they wouldn't mention this rebuttal if they knew about it):
Fails NEPA requirement to identify opposing views to its main arguments such as the Mars meteorite argument
This also fails another NEPA requirement to identify opposing views:
At appropriate points in the final statement, the agency shall discuss any responsible opposing view that was not adequately discussed in the draft statement and shall indicate the agency's response to the issues raised.
§ 1502.9
The PEIS doesn't mention the opposing view to its Mars meteorite argument in the very cites NASA uses. See:
The PEIS even presents the Mars meteorite argument as a scientific consensus. The opposing view is the consensus in the peer reviewed planetary protection literature.
On the last point of omissions of opposing views
An agency must address in an EIS “responsible opposing view[s].” Courts have interpreted this regulation as requiring agencies to address opposing scientific viewpoints. In recent years, courts have given an agency’s response to opposing scientific viewpoints deferential treatment, so long as the agency addressed the opposing statements and differing opinions in a meaningful way during the decision-making process.
(Feldman et al., n.d. NEPA’s Scientific and Information Standards—Taking the Harder Look)
So, for instance on the topic of environmental effects, it seems the courts would be able to pass it as a valid Environmental Impact Statement under NEPA based on NASA’s own statement that in their view there is no significant risk of environmental effects, that the Mars meteorite argument is valid, that Mars is uninhabitable, etc.,, so long as NASA alert the reader to the opposing views and so long as NASA address these differences of view in a meaningful way in the EIS.
Presumably NASA would also need to discuss the reasons their former planetary protection officers, the ESF and the NRC gave for their views, and explain why they came to a different view.
The issue here is that NASA doesn't identify these views anywhere in the EIS even though they are expressed in the cites it uses.
Fails NEPA requirement to discuss major points of view by not mentioning the view of a low risk of large-scale harm
NEPA also requires agencies to discuss all major points of view
At appropriate points in the draft statement, the agency shall discuss all major points of view on the environmental impacts of the alternatives including the proposed action.
§ 1502.9
The major point of view that there is a low but not zero risk of large-scale harm to human health and the environment is also a consensus in all the major peer reviewed studies of a Mars sample return.
This major view is not discussed, indeed there is no mention in the PEIS of even a low risk of large scale harm to human health by risk group 4 organisms, or of large scale changes to Earth's biosphere or ecosystems.
The consensus view in the Mars sample returned studies is that the potential affected area is global.
Once again they don't mention the opposing view of their own second planetary protection officer Cassie Conley that low risk potential health impacts are global, who in an official video still on NASA's YouTube channel express the same view as the consensus in the peer reviewed literature. See above:
Fails NEPA requirement to include reasonably foreseeable environmental effects in discussion of the affected environment
From the outset, NASA reduced the scope of the project to the Utah Test and Training Range. But that's not based on new scientific understanding. It is based on NASA's own non peer reviewed reasoning based on four invalid arguments and its invalid final deduction step from low risk of significant environmental effects to a judgement that any environmental effects would not be significant.
The PEIS never mentions the majority view that the worst case is global (Ammann et al., 2012, Mars Sample Return backward contamination–Strategic advice and requirements 20, 24, 58) (SSB, 2009, Assessment of planetary protection requirements for Mars sample return missions : 48).
This fails requirements on identifying the affected environment:
NEPA clarifies that reasonably foreseeable in section 1502 includes low likelihood events with catastrophic consequences, and a low likelihood of harm to the entire biosphere of Earth or to human health globally counts as catastrophic consequences§ 1502.15 Affected environment
The environmental impact statement shall succinctly describe the environment of the area(s) to be affected or created by the alternatives under consideration, including the reasonably foreseeable environmental trends and planned actions in the area(s).
(d) For the purposes of this section, “reasonably foreseeable” includes impacts that have catastrophic consequences, even if their probability of occurrence is low, provided that the analysis of the impacts is supported by credible scientific evidence, is not based on pure conjecture, and is within the rule of reason.
§ 1502.21 (c) and (d)
NASA in response to this as they clarified in their comment reply to me in the final PEIS is that they consider that this requirement was resolved by their reasoning to no significant effects in the PEIS.
Section 3.2 of the PEIS clearly outlines these requirements and addresses these issues.
This refers to their novel deduction of no significant impacts from the samples based only on its assessment of an unlikely risk of significant ecological impact. NASA are of the view that this fulfills the NEPA requirements. See:
Fails NEPA requirement on tiering by deferring the Mars Receiving Facility to tier 2 when it is identified as a major issue in the NRC and ESF studies
The Council on Environmental Quality explains that tiering is appropriate when it helps the lead agency to focus on the issues which are ripe for decision and exclude those that are not yet ripe for decision or already decided.
Tiering is appropriate when the sequence of statements or analyses is:
- From a program, plan, or policy environmental impact statement to a program, plan, or policy statement or analysis of lesser scope or to a site-specific statement or analysis.
- From an environmental impact statement on a specific action at an early stage (such as need and site selection) to a supplement (which is preferred) or a subsequent statement or analysis at a later stage (such as environmental mitigation). Tiering in such cases is appropriate when it helps the lead agency to focus on the issues which are ripe for decision and exclude from consideration issues already decided or not yet ripe.
(CEQ, 2007, A citizen’s guide to the NEPA: Having your voice heard : 49)
Issues to do with quarantine for lab leaks in a Mars Receiving Facility are ripe for decision at tier 1, because it affects whether such a facility can be built at all and whether a lab with human technicians can handle the sample or whether it needs telerobotics.
The ESF recommendation to contain 100% of all particles at 0.05 microns upwards is also ripe for decision in tier 1 because the technology to contain such particles doesn't exist yet.
Also potential effects of release from the Mars Receiving Facility are global, while the Utah sands retrieval handles the samples enclosed in a container that is unlikely to be breached and with a low risk of any Martian particles that gets to the outside of it. So the highest level of risk from potential impacts may well be from lab leaks from the Mars Receiving Facility after the capsule is opened, rather than the Utah sands.
So it wasn't appropriate to restrict tiering to the Utah test range and exclude from the Environmental Impact Statement all discussion of how to handle the samples later in a Mars Receiving Facility.
Misidentifying the affected location from the outset surely by itself makes this invalid under NEPA.
See above:
This tiering also lead to NASA only publicising the two rounds of public comments in local newspapers in the region of the Utah Test and Training Range (and Kennedy Space Center in Florida)
With an assessment of a potential global impact they would have needed to ensure a much wider audience was aware and involved.
Fails NEPA requirement to select preparers with disciplines appropriate to the Impact Statement's scope and issues
We conclude also from the Mars meteorite sentence, which has remained unchanged through to the final PEIS even when challenged by the public, that there is nobody in NASA's Mars Sample Return team is familiar enough with the planetary protection literature to know that the Mars meteorite argument you rely on was rebutted over 20 years ago, and nobody in NASA's team had enough interest in the topic to read the most important cite for planetary protection in the PEIS carefully as far as page 5.
That is another NEPA failing
The disciplines of the preparers shall be appropriate to the scope and issues identified in the scoping process
§ 1502.6
The scoping process refers to the first round of public comments (NASA, 2023, NEPA - Mars Sample Return Campaign). These include (NASA, 2022, The Safety of Mars Sample Return) and (NASA, 2022, Proposed action and no action alternative)
For an Environmental Impact Statement for samples returned from Mars one thing we can be sure is a requirement that preparers are familiar with the literature on a Mars sample return mission.
Also for a biosafety plan to protect Earth's biosphere and its inhabitants, there can't be much controversy about the need to have experts in risk assurance and public health.
Then from the need to build a sample return facility there's a clear need for experts on the design of biosafety labs.
It is clear from the PEIS itself that NASA have no experts amongst those tasked with preparing the PEIS in:
- risk assurance from their answers to Chester Everline, one of their experts on probabilistic risk assurance
See above: NASA's novel deduction that there would be NO significant ecological impacts, based only on its assessment that samples are UNLIKELY to pose a risk of significant ecological impact and
Also: NASA clarified that the Mars Sample Return mission has no campaign level probability target for containment but this is not mentioned in the text of the PEIS - public health because they would have immediately alerted NASA's MSR team to the presence of pathogens such as tetanus or Aspergillus fumigatus that are not adapted to an infectious lifestyle in any organism. It is clear none of NASA's team knew this from the Biological Safety Report conclusion and that nobody challenged it.
See above: NASA's Biological Safety Report's finding of near zero risk that martian life can harm us rebutted by soil pathogens like tetanus and aspergillus fumigatus not adapted to any terrestrial host - biosafety lab design as nobody on their team understands how HEPA filters work, Or they don't understand the ESF recommendation.
See above: Why more is needed than "NASA does not concur" for the issue of ultramicrobacteria containment - planetary protection for a Mars sample return mission, nobody on the team thoroughly familiar with the literature on the topic or they'd have immediately spotted that their main cites rebutted the Mars meteorite argument.
See above: How NASA's central finding that any harmful life can get here better protected in meteorites is rebutted by BOTH of its main cites for it
The peer reviewed literature describes the details for a Sample Receiving Facility as a major and very complex part of a sample return mission. It is not discussed in this PEIS.
For a longer list of some of disciplines that are needed for an adequate biosafety plan based on the issues found with NASA's PEIS in this book see above:
Based on this Programmatic Environmental Impact Statement (PEIS) it is clear that NASA is not an organization with the in-house expertise to run a biosafety lab suitable to protect Earth or devise a biosafety plan for Earth. It needs outside help from an interagency panel and experts of many other disciplines and it also has to involve internal experts in this project from area of expertise it does have such as risk assurance.
Since NASA wasn't able to get together a team capable of doing an adequate PEIS with the appropriate disciplines needed to prepare it, it also doesn't at present have the expertise needed to design and run the lab itself. It needs help from other agencies and experts of other disciplines.
Fails NEPA requirement for an interdisciplinary approach integrating the social sciences by not developing the tools needed to involve the public in risk governance
This is covered in:
Agencies shall prepare environmental impact statements using an interdisciplinary approach that will ensure the integrated use of the natural and social sciences and the environmental design arts (section 102(2)(A) of NEPA).
§ 1507.2
Together with the requirement on the disciplines of the preparers
The disciplines of the preparers shall be appropriate to the scope and issues identified in the scoping process
§ 1502.6
All the Mars sample return studies have talked about the need to work with the public on risk governance from an early stage needing experts in methods for working with the public.
Mars sample return studies emphasize the need to involve the public early on, not just in the USA, but through fora open to representatives from all countries globally because negative impacts could affect countries beyond the ones involved directly in the mission. This is from the most recent ESF study in 2012 which says this should be done as soon as possible and specifically mentioning civil society generally, and the scientific community.
It recommends tools are developed to effectively interact with individual groups and mechanisms and fora are set up dedicated to the ethical and social issues.
RECOMMENDATION 3
…, civil society, the key stakeholders, the scientific community and relevant agencies’ staff should be involved in the process of risk governance as soon as possible.
… Tools to effectively interact with individual groups should be developed (e.g. a risk map).
RECOMMENDATION 4
…mechanisms and fora dedicated to ethical and social issues of the risks and benefits raised by an MSR are set up at the international level and are open to representatives of all countries
(Ammann et al., 2012, Mars Sample Return backward contamination–Strategic advice and requirements : 59)
The interagency panel had at least four members with expertise in knowledgeable in one or more of the fields of bioethics, law, public attitudes and the communication of science, the Earth’s environment, or related fields, by its charter (SpaceRef editor, 2001, Planetary Protection Advisory Committee Charter)
However NASA lost that expertise when they closed down the panel in 2007. See above::
No such fora were ever set up and NASA have nobody on their team familiar with the social and design arts of involving the public in risk assurance. When asked about this they were of the view the requirement was satisfied by NASA's participation in various committees such as COSPAR which shows a lack of appreciation of the reason for the requirement. This issue is seen throughout NASA's responses to public comments, which are the responses of engineers not experts in communication with the public on risk.
It is clear from the public responses to the draft EIS that they didn't manage to communicate well with concerned members of the public, and this illustrates the issues the Mars Sample Return studies identified. See:
This is for a PEIS that was only advertised in local newspapers in communities close to the Utah Test and Training Range.
Fails NEPA requirements to consider reasonable alternatives that don't fully meet the agencies listed goals but better meet NASA and NEPA policies and requirements
The NEPA requirement here is that agencies have to evaluate reasonable alternatives and briefly discuss reasons for elimination of any alternatives eliminated from detailed study
§ 1502.14 Alternatives including the proposed action.
The alternatives section should present the environmental impacts of the proposed action and the alternatives in comparative form based on the information and analysis presented in the sections on the affected environment (§ 1502.15) and the environmental consequences (§ 1502.16). In this section, agencies shall:
(a) Evaluate reasonable alternatives to the proposed action, and, for alternatives that the agency eliminated from detailed study, briefly discuss the reasons for their elimination.
(b) Discuss each alternative considered in detail, including the proposed action, so that reviewers may evaluate their comparative merits. ~
§ 1502.14 -
This has to include a summary that identifies all the alternatives submitted by public commenters.
The final environmental impact statement shall include a summary that identifies all alternatives, information, and analyses submitted by State, Tribal, and local governments and other public commenters for consideration by the lead and cooperating agencies in developing the final environmental impact statement.
§ 1502.17
The Council on Environmental Quality says an agency has to consider alternatives that don't fully meet all its goals.
An agency also has to look at alternatives that don't meet the applicants listed goals but better meet the policies and requirements set forth in NEPA and the agency's statutory authority and goals
(CEQ, 2022, National Environmental Policy Act Implementing Regulations Revisions - a rule by the CEQ on 4/20/2022)
All three of these reasonable alternatives fit that description in one way or another.
- Sterilize all sample returned to Earth
- doesn't meet all the listed goals but better protects Earth's inhabitants and the biosphere so better meets policies and requirements of NEPA - Search for life in situ and defer return of the samples until we can do risk assurance better
- achieves the astrobiology goals in a different way and astrobiologists have said in many papers that their goals are better served by in situ searches than samples returned from Mars. So it likely achieves MORE science return for astrobiology than the mission plan. - Return samples to a miniature life detection lab above GEO
- The miniature lab above GEO also provides MORE science return though in a different way from the way envisioned in NASA's mission plan by adding those bonus samples in clean containers
- It retains virtually all the geology since the sample tubes are just sterilized and returned to Earth unopened and all, or almost all have already received - much more than a sterilizing dose of ionizing radiation on Mars
the main geological alterations of rock samples such as colour changes of halite crystals from clear to blue and quartz crystals from clear to brown and changes in thermoluminescent properties happen after 0.3 Mrad, only 3 million years of ionizing radiation (Allen et al., 1999. Biological sterilization of returned Mars samples) - Perseverance has no way to measure exposure ages and so is unlikely to return samples with a young enough exposure age to be affected noticeably
- the youngest exposure age measured by Curiosity is 80 million years (Pavlov et al., 2022. Rapid Radiolytic Degradation of Amino Acids in the Martian Shallow Subsurface: Implications for the Search for Extinct Life : 1100 - 1101)
A properly drafted purpose and need statement should lead to consideration of the reasonable alternatives to the proposed action, consistent with NEPA's requirements. See 42 U.S.C. 4332(2)(C), 4332(2)(E). CEQ disagrees with commenters assertions that consideration of alternatives that do not meet an applicant's goals or cannot be implemented by the applicant will always waste applicant or agency resources or result in delays. There may be times when an agency identifies a reasonable range of alternatives that includes alternatives—other than the no action alternative—that are beyond the goals of the applicant or outside the agency's jurisdiction because the agency concludes that they are useful for the agency decision maker and the public to make an informed decision.
Always tailoring the purpose and need to an applicant's goals when considering a request for an authorization could prevent an agency from considering alternatives that do not meet an applicant's stated goals, but better meet the policies and requirements set forth in NEPA and the agency's statutory authority and goals. The rule of reason continues to guide decision making in such contexts.
(CEQ, 2022, National Environmental Policy Act Implementing Regulations Revisions - a rule by the CEQ on 4/20/2022)
The revision clarifies that agencies have discretion to consider a variety of factors when assessing an application for an authorization, removing the requirement that an agency base the purpose and need on the goals of an applicant and the agency's statutory authority
However they can't restrict the purpose to exclude "truly" reasonable alternatives. They can't use this to make the PEIS incompatible with NEPA requirements.
It is contrary to NEPA for agencies to “contrive a purpose so slender as to define competing `reasonable alternatives' out of consideration (and even out of existence).” Simmons v. U.S. Army Corps of Engineers, 120 F.3d 664, 666 (7th Cir. 1997) (citing 42 U.S.C. 4332(2)(E)).
Constricting the definition of the project's purpose could exclude “truly” reasonable alternatives, making an EIS incompatible with NEPA's requirements. Id. See also, e.g., Nat'l Parks & Conservation Ass'n v. Bureau of Land Mgmt., 606 F.3d 1058, 1070 (9th Cir. 2010) (“Agencies enjoy `considerable discretion' to define the purpose and need of a project. However, `an agency cannot define its objectives in unreasonably narrow terms.'” (internal citations omitted)).'
(CEQ, 2022, National Environmental Policy Act Implementing Regulations Revisions - a rule by the CEQ on 4/20/2022)
The CEQ also clarified that the requirement to consider reasonable alternatives persists even after its narrowing of scope in its 2021 revision of NEPA (CEQ, 2020, National Environmental Policy Act Implementing Regulations Revisions - Supplementary information).
NASA required alternatives to definitively determine if it is safe to return unsterilized samples, even if all returned samples are sterilized, yet there will be no way even for terrestrial labs to prove the samples don't have small numbers of martian microbes
That's here, in this passage, the SSAP means [Sample Safety Assessment Protocol], a protocol to test the samples for the presence of Martian life. In this protocol, if none is found then they can be released form the labs, if Martian life is found the samples are never released because the effects can't be predicted from unknown novel life.
Given the needs above, Mars sample processing and analysis cannot be sufficiently conducted in situ, and any alternative associated with sample analysis under the MSR Campaign must be able to accommodate the processes and associated equipment required to conduct the level of analysis required to meet MSR Campaign objectives, including a comprehensive SSAP [Sample Safety Assessment Protocol]
[i.e. any alternative must be able to complete the sample safety assessment protocol to prove that it is safe to release unsterilized samples to the labs].
Additionally, given the constraints described above, there is no instrument or suite of tests that Perseverance can use on Mars or that the MSR Campaign could bring to Mars, to definitively determine if the samples collected are of sufficiently low risk so as to alter the "Restricted Earth Return" mission planetary protection designation and being treated as if they are potentially hazardous.
[excludes in situ searches because they can't be used to prove that the samples can be returned to Earth with no restrictions as for the Moon]
(NASA, 2023. Mars Sample Return final PEIS : 2-25)
This improperly removes from consideration all three of those reasonable alternatives
No safety testing is needed for samples returned sterilized or unsterilized samples studied on Mars or unsterilized samples studied above GEO.In addition we see from that passage that their prescreening requirement is that the alternatives must "definitively determine if the samples collected are of sufficiently low risk so as to alter the "Restricted Earth Return" mission planetary protection designation"
It turns out that NASA's own mission plan is guaranteed to fail that prescreening requirement, so, strictly interpreted, they should eliminate their current plan from consideration too by that same prescreening criterion.
The reason is that NASA's biosafety testing is guaranteed to fail with all samples going to hold and critical review indefinitely because of
- the high levels of terrestrial contamination,
- the impossibility of an adequate inventory of terrestrial life
- and the potential for ultra low levels of present day life, even just a few viable microbes in the entire collection,
So NASA's Needs and purpose section not only improperly rules out all three of these alternatives submitted by the public by setting a requirement that any alternative has to be able to prove that it is safe to return the samples to Earth unsterilized
This requirement is also one that NASA's own mission plan can't fulfill as there is no way that NASA will be able to prove it is safe to release the samples unsterilized with even very weak levels of assurance.
For details see:above in: Why the COSPAR Sample Safety Assessment Framework can't be used to prove safety of unsterilized Perseverance samples
NASA also required all alternatives to test unsterilized samples for present day and past life in a terrestrial laboratory based on out of date information about in situ capabilities
"Given the needs above, Mars sample processing and analysis cannot be sufficiently conducted in situ … "
(NASA, 2023. Mars Sample Return final PEIS : 2-25)
NASA's summary of the capabilities of in situ instruments is based on a limited literature search that only turned up information 14 years out of date
The International Mars Architecture for the Return of Samples Working Group, in 2008, evaluated the overall goals and objectives of Mars exploration and determined that, given the scope of what is realistically achievable via in situ exploration technology, a significant fraction of these investigations could not be meaningfully advanced without returned samples for the following reasons (iMARS Working Group 2008, Meyer et al. 2022):
(NASA, 2023. Mars Sample Return final PEIS : 3–3)
Their main 2008 cite (MARS Working Group, 2008. Preliminary Planning for an International Mars Sample Return Mission : 9) predates all the more modern in situ instrument designs. Their 2022 cite (Meyer et al, 2022, Final Report of the Mars Sample Return Science Planning Group 2 (MSPG2)) doesn't seem to have any discussion of in situ instruments.
The Mars sample return team were not aware of the survey from 2016 of in situ instrument capabilities for their own agency's Europa lander mission proposal (Hand et al., 2017, Report of the Europa Lander Science Definition Team).
I gave a list of many example instruments we could send to above GEO with present day technology or near future technology, based on their own Europa team report and other sources in my attachment 8 of the final comment but NASA prescreened this attachment as "nonsubstantive" and so didn't see that list.
See:
- NASA’s screening process for alternatives based on life detection in situ or in a robotic satellite lab was outdated and missed the in situ life detection instruments listed in its own 2016 Europa lander proposal
- Modern miniature life detection instruments that could fly to above GEO, or to Mars
This issue of inappropriate prescreening also impacted on their assessment of Chester Everline's suggestion of a deferred sample return
⸽ How reasonable alternatives to NASA's plans can resolve these issues with NEPA by removing the need to prepare a comprehensive biosafety plan for unsterilized samples returned to Earth
So let's look at how NASA could resolve these issues based on the conclusions of this book (see above):
NASA can resolve nearly all these NEPA issues by pre-sterilizing samples that return to Earth. It then
- Doesn't need "in house" expertise in human health, quarantine, epidemiology or design of biosafety labs if NASA no longer plans a terrestrial biosafety lab, though NASA should still restore the interagency panel to get their input.
- Still needs expertise in risk assurance but with a much easier remit
- Does need expertise in planetary protection, but again with a much easier remit
No matter what NASA does it clearly does need experts with familiarity with the planetary protection literature for a Mars sample return mission.Even if the aim is to return unsterilized samples to above GEO and sterilize all samples returned to Earth NASA needs experts who have familiarity with the literature on a Mars sample return mission.
I hope that after reviewing this book, at some point NASA will agree it should NEVER AGAIN find itself in a situation where it prepares a PEIS for samples returned from Mars where the preparers haven't read as far as page 5 of its most important planetary protection cite.
NASA no longer has this expertise or it couldn't produce a PEIS like this. It can restore this expertise if it follows the advice of the Space Studies board to urgently need to restore its independent planetary protection office.
Also it's clear that NASA's planetary protection office needed the extra full time equivalent civil servant NASA's planetary protection office called for in 2016 just before NASA closed it down. It also needs those for private space and for forward protection for its other missions.
PPS issued a recommendation that NASA assign an additional full-time equivalent (FTE) civil servant to the PPO, reasoning that a one person office at Headquarters is inadequate given the growing number of missions that have planetary protection consequences. PPS notes that there is increasing load on PPO that is not accompanied by concomitant funding
(Peterson, 2016, National Advisory Council Science Committee Meeting Report, July 25-27, 2016 : 9)
The issues with this PEIS wouldn't have arisen if NASA had kept its planetary protection office and listened to their officers.
However the task for the preparers of a PEIS will be far easier with a pre-sterilized samples.
The main issue to resolve is
- the level of sterilization needed to protect Earth which needs widespread public involvement and expertise from many disciplines including synthetic biology.
NASA does need to
- set up public fora and engage better with the broader public and not just with enthusiasts for humans to Mars for success for this mission.
Even though the presterilized sample return keeps Earth 100% safe with no appreciable risk, this is something that needs to be done with broad consultation with the public so those fora and risk communication and other requirements mentioned in the Mars sample return studies are still needed.
However again the tasks are greatly simplified if all samples returned to Earth are sterilized.
The potential scope needs to be set to global from the start and then the PEIS can explain how sterilizing samples returned to Earth achieves biosafety.
Looking further ahead the Congress can do a lot to make planetary protection easier and more effective. See:
- NASA world leading on planetary protection until it closed down its office at early stages of Mars sample return planning
- Need to avoid a trade-off between planetary protection and NASA's obligations to minimize costs
- An independent body to safeguard Earth and Mars: avoiding perverse incentives to reduce safety by ensuring planetary protection costs don't come from NASA's budget
Some mechanism is also needed to ensure private space has adequate planetary protection, and given the public good of protecting Earth, and protecting the value of Mars for science, it seems appropriate to have a similar approach with private space that up to 10% (or more) of the cost of a mission can be supplied as a grant for planetary protection
If NASA still wishes to return unsterilized samples far more is needed. It needs the relevant expertise on its team:
- NASA's team must include experts in public health, environmental issues, biosafety design and risk assurance
- NASA needs to restore the interagency panel to get access to its expertise
- NASA's also needs to address the systemic issues that led to its engineers and mission planners not listening to the advice of the interagency panel and its planetary protection experts
NASA needs to be up to date on the scientific research that's been done since 2009 and do an updated level of assurance and size limit review
- NASA needs to do an updated Mars Sample Return study
- NASA needs an updated size limit and level of assurance review
- NASA needs to initiate a technology review and assessment of the technology needed for a fully telerobotic facility to contain the samples within the recommended size limit and level of assurance or some other plan that is able to respond to lab leaks by containing any alien or distantly related biology found, even mirror life or a novel genus of fungus.
- NASA then needs to develop a roadmap for that technology and estimate the timescale needed to achieve it and have a Mars Receiving Facility ready to receive the samples and evaluate costs
The technology doesn't exist yet but it seems plausible that we can develop the technology to return unsterilized samples. However, the conclusion of this book is that it is likely to cost significantly more and have a much longer timeline with far higher risk of cost overruns and timeline overruns since most of the enabling technologies are at TRL 1 or not even reached the TRL system as they haven't been given enough attention yet to have a fully worked out suggestion even in principle to achieve 100% containment of ultramicrobacteria (which may be turned into 100% containment of ribocells on review).
See:
Also alternatives that return only sterilized samples to above GEO have the additional benefit of no appreciable risk. Whether or not that becomes a requirement after a size limit and level of assurance review, it would certainly be seen by the public as a benefit of these alternatives.
Covered above in
By the presidential directive NSC-25 (possible large-scale adverse environmental effects), NASA should draw their PEIS to the attention of the Director of OSTP which can lead to the chair of the CEQ ordering further studies
Presidential directive NSC-25 requires "a review of large scale effects that could be reasonably expected to result in allegations of major or protracted effects"
The process begins with the head of the agency, in this case the head of NASA. They should recommend such a review even if the agency feels confident such allegations are unfounded
It should be understood that experiments which by their nature could reasonably be expected to result in domestic or foreign allegations that they might have major and protracted effects on the physical or biological environment, or other areas of public or private interest, are to be included under this policy even though the sponsoring agency feels confident that such allegations would in fact prove to be unfounded.
(White House, 1977, Presidential Directive NSC-25: Scientific or Technological Experiments with Possible Large-Scale Adverse Environmental Effects and Launch of Nuclear Systems into Space).
The PEIS does mention this directive.
For missions returning samples from planetary bodies that might have major and protracted effects on the physical or biological environment, NASA is required to address Presidential Directive/National Security Council-25, Scientific or Technological Experiments with Possible Large-Scale Adverse Environmental Effects and Launch of Nuclear Systems into Space, by presenting detailed information regarding the importance and potential environmental effects of the mission in this PEIS.
(NASA, 2023. Mars Sample Return final PEIS : 1-5)
However that is all it says. It then goes on as we saw to reason that any environmental effects would not be significant and that any public health effects negligible. But those are the views of the agency.
In NASA's summary of the directive, it misses out the word "allegations" which is a significant omission. It may have led them to believe they fulfilled the requirement by giving their own novel reasoning in the PEIS to the effect that the allegations would prove to be unfounded.
However in the public comments in response to the many members of the public concerned about large-scale harm, NASA do NOT say that large-scale harm is impossible. Instead they say the risk of large-scale harm can't be demonstrated to be zero.
Even in response to a question from a member of the public asking if a possible consequence is a mass extinction or that humans might have to live in biomes on Earth for the rest of time as a result of this sample return, NASA say that the risk of thesis can't be demonstrated to be zero:
So although this isn't covered in the main body of the PEIS NASA is on record in the public comments section of the PEIS as saying that it is impossible to demonstrate zero risk of even unprecedented harm that could significantly change the habitability of Earth for humans for all future time, which is not a consideration for a normal biosafety laboratory.
We also saw all the major peer reviewed studies of a Mars sample return concur on a likely low but non zero risk of large-scale harm.
Assuming the head of NASA agrees that there is potential for concern about large-scale effects they then call their proposals to the attention of the Director of the Office of Science and Technology Policy, the Director then consults with the Chairman of the Council on Environmental Quality.
It has to be done even if the agency feels confident such allegations are false
Together they review the proposals and the supporting materials and can order further studies if they don't feel the potential allegations have been addressed adequately.
An impact statement that recognizes a low risk potential for large scale harm to the environment and public health has to consider impacts on the Great Lakes, or oceans, or invasive species, and many executive orders are triggered
We saw that NASA's PEIS is the outlier with its conclusion that any environmental effects would not be significant.
The relatively low probability of an inadvertent reentry combined with the assessment that samples are unlikely to pose a risk of significant ecological impact or other significant harmful effects support the judgement that the potential environmental impacts would not be significant.
(NASA, 2023. Mars Sample Return final PEIS : 3-16)
See above:
An impact statement that concurs with the conclusions of the NRC and the ESF will recognize a low risk potential for large scale harm.
The Study Group also concurs with another conclusion from the NRC reports (1997, 2009) that the potential for large-scale effects on the Earth’s biosphere by a returned Mars life form appears to be low, but is not demonstrably zero.
(Ammann et al., 2012, Mars Sample Return backward contamination–Strategic advice and requirements 20)
Previous studies said numerous laws to protect Earth’s environment would be triggered by a Mars sample return and that other agencies would be involved in evaluating the effects such as the CDC, Department of Agriculture.
The most recent of these is co-authored by NASA's second planetary protection officer:Cassie Conley: (Uhran et al., 2019, Updating Planetary Protection Considerations and Policies for Mars Sample Return) and the earlier one is by Margaret Race for COSPAR (Race, 1995, Planetary Protection, Legal Ambiguity, and the Decision Making Process for Mars Sample Return)
Here are some relevant executive orders and federal laws NASA must consider in this PEIS once they say in it that there is a potential for large-scale effects on the environment and on human health (NASA, 2012, NASA Facilities Design Guide).
- 13112 Invasive Species Federal agencies are to prevent the introduction of invasive species, to provide for their control, and to minimize the economic, ecological, and human health impacts that invasive species cause
- 13158 Marine Protected Areas Federal agency actions are to avoid harm to the natural and cultural resources that have been designated a Marine Protected Area (MPA).
- 13186 Responsibilities of Federal Agencies to Protect Migratory Birds In their land management and environmental quality planning, Agencies are to avoid/minimize adverse impacts on and to prevent/abate pollution or detrimental alteration of the environment of migratory bird resources.
[migratory birds could be affected by environmental changes from introduction of mirror life, or by novel diseases such as a species from a novel fungal genus] - 13547 Stewardship of the Ocean, Our Coasts, and the Great Lakes Federal Agencies are to exercise stewardship of oceans, coasts, and the Great Lakes by protecting, maintaining, and restoring their health and biological diversity.
- Endangered Species Act Through federal action and by encouraging the establishment of state programs, the 1973 Endangered Species Act provided for the conservation of ecosystems upon which threatened and endangered species of fish, wildlife, and plants depend
[effects of introduced microbes from Mars on ecosystems would not be possible to predict] - 13486 Strengthening Laboratory Biosecurity in the United States Facilities that possess biological select agents and toxins are to have appropriate security and personnel assurance practices to protect against theft, misuse, or diversion to unlawful activity of such agents and toxins.
Also representative federal laws that apply::
- Migratory Bird Treaty Act Act for the protection of migratory birds.
- Safe Drinking Water Act This Act regulates drinking water systems
[microbial life from Mars could potentially have effects on drinking water]
This would seem to be necessary from NASA's own responses to the general public in the comments under:
But there is nothing in the PEIS relating to these executive orders. However the other agencies haven't challenged the PEIS.
Apart from the possibility of legal challenges I haven't been able to find out what the implications are of not complying with these executive orders.
Obligations for ESA countries based on the conclusion of the ESF study from 2012 on low risk of large-scale harm
I have not found a paper on the legal process for a Mars sample return for ESA member states. So these are only preliminary observations. The initial sample receiving facility will be in the USA with the current plans. However, ESA, and so, ESA member states are directly involved in returning the samples from Mars to the USA. ESA's cooperation is also essential in the sense that NASA would need to find a new source of funding to launch the Sample Retrieval Lander or the Earth Return Orbiter with its Earth Return System this decade, if ESA was to pull out of the mission. (NASA, n.d., Mars Sample Return Mission)
Directive 2001/42/EC would apply Directive 2001/42/EC of the European Parliament and of the Council of 27 June 2001 on the assessment of the effects of certain plans and programmes on the environment. This is similar to NEPA, including the need for a draft report, public comments and so on. This is an example of how it is implemented in the UK, which still retains the law though no longer in the EU. (Strategic Environmental Assessment Directive: guidance - Practical guidance on applying European Directive 2001/42/EC)
Also the EU, UK and many other member states of ESA are parties to the Espoo convention (UN, n.d., Convention on Environmental Impact Assessment in a Transboundary Context). Under that convention they need to consult with each other on all major projects that can have an effect outside of their own boundaries (UNECE, n.d., Environmental Assessment - Espoo Convention), which would certainly apply to the low risk worst case of a Mars sample return irreversible release of mirror life to our biosphere.
Then, though the USA is not part of the Convention on Biological Diversity, all the participating nations of ESA are, indeed all UN nations except the USA which leads to obligations to prevent introduction of alien species that threaten ecosystems, habitats or species:
"Each Contracting Party shall, as far as possible and as appropriate:
… (h) Prevent the introduction of, control or eradicate those alien species which threaten ecosystems, habitats or species."
Convention on Biological Diversity, 1997, Legal text
Any Mars sample return mission also has implications internationally e.g. for the WHO, FAO, etc
There are many other relevant conventions and treaties and once the US says there is potential for large scale effects, it would likely involve:
- international organizations like the WHO, FAO, etc
- international treaties
No matter which country is involved in planning a Mars sample return mission, at some stage, international agencies like the Food and Agriculture Organization may get involved, because of potential impact on agriculture and fisheries and global food supplies, and the World Health Organization because of effects on human health globally if a new organism is returned that can be spread to other countries.
They might not have much by way of authority but they would affect public opinion in the originating country and globally and governments of other countries might well get involved.
There are many international implications. See:(Uhran et al., 2019, Updating Planetary Protection Considerations and Policies for Mars Sample Return) and (Race, 1995, Planetary Protection, Legal Ambiguity, and the Decision Making Process for Mars Sample Return)
Potential for private individuals in the USA to litigate the government on violation of the Outer Space Treaty
It's not necessarily Congress or the president that might halt the mission in the USA. Unusually there may be a potential for private American citizens to litigate their own government on international treaties.
Chris McKay writes:
Importantly, in the US private individuals can also act though the courts to stop missions if that mission does not comply with US treaty obligations.
Thus, there is the specter of astronauts on Mars prohibited from returning to Earth based on a successful lawsuit claiming a lack of due diligence in the question of Martian life as required by the Outer Space Treaty.
(McKay, 2019,. Prerequisites to Human Activity on Mars: Scientific and Ethical Aspects : 319)
This seems to be the central point in the argument for those interested in the legal details :
The supremacy clause and the British Rule Article VI of the Constitution provides:
This constitution and the Laws of the United States which shall be made in Pursuance thereof; and all Treaties made, or which shall be made,under the Authority of the United States, shall be the Supreme Law of the Land; and the Judges in every State shall be bound thereby, any Thing in the Constitution or Laws of any State to the Contrary notwithstanding,
The bare text of this clause establishes that treaties are to be given effect by judges. It strongly suggests that treaties are to be applied by judges in the same circumstances as federal statutes and (leaving aside the question of hierarchy) the Constitution.
The clause's reference to judges contradicts the claim that treaties, being contracts between independent nations, "depend[] for the enforcement of [their] provisions on the interest and the honor of the governments which are parties to [them]" rather than on lawsuits. Although treaties may depend on "interest and honor" on the international plane, under the Constitution, the treaty obligations of the United States are also enforceable in courts
(Vázquez, 2008. Treaties as Law of the Land: The Supremacy Clause and the Judicial Enforcement of Treaties)
Another legal expert, Stephan argues that there are limitations here and that private citizens can only take out cases of treaty violations with the court if the violation has direct consequences for the litigants
The alternative is to consider the deciding of cases as an instrumental matter. By this I mean that outcomes have direct consequences for litigants and indirect but significant implications for persons who look at decided cases as evidence of the rule that may be applied to their activities
(Stephan, 2007. Private remedies for treaty violations after Sanchez- Llamas)
That is a matter that would need to be decided by the Supreme Court.
However return of extraterrestrial life that could impact on Earth's biosphere does seem to fit that criterion at least so long as it is acceptable to consider potential outcomes as for NSC-25.
If NASA's case is not securely grounded, it seems most likely that the mission would be stopped in Congress or by a presidential directive or executive order. But there may be potential for private litigation to stop it too.
⸽ My background: I was working on my first astrobiological paper when the NEPA comments process started, about NASA's Mars sample return mission, not yet submitted to journals
Continues from:
I commented on both rounds of public comments and so have legal standing. This means NASA are legally required under NEPA to respond to serious issues I raised and to consider any reasonable suggestions I make.
My background is I was working on my first astrobiology paper, on NASA's very mission at the time of the PEIS. However, NASA's team are unfamiliar with the planetary protection literature as we see already from the main Mars meteorite cite, and numerous other details in the PEIS and replies to public comments. They can't see any issues with their PEIS and are about to finalize it or may have finalized it already.
In more detail, I was finishing what was going to be my first paper on astrobiology, on planetary protection for NASA's mission when the Environmental Impact Statement process started. Amongst other things my preprint covered
- what Sagan called the "vexing issue of latency" for quarantine of technicians after lab leaks, which doesn't seem to be discussed in modern planetary protection literature though there is a fair bit about it from the Apollo era. It is a serious issue that needs to be looked at, since quarantine is essential to plans to contain a lab leak of extraterrestrial life in a lab run by human technicians
See: Even the most well prepared labs can have lab leaks - Sagan's insoluble "vexing question" of the quarantine period for a novel alien pathogen - legal issues
- novel planetary protection scenarios such as mirror life to encourage space agencies and private space to take planetary protection seriously
See: Mirror life as a potential astrobiological surprise like the unexpected carbon dioxide geysers for geology - the ESF recommendation to contain 100% of all particles from 0.05 microns upwards
- issues with achieving the ESF recommendation with present day air filter technology
I focused on the ESF recommendation in the first sentence of my first comment under NEPA, as in my work on my preprint I had found no mention of any work on the technology needed to achieve this level of containment (Walker, 2022, Comment posted on May 16th).
Sadly NASA's team ignored my comment for the draft PEIS. They finally commented on the final round of public comments that they didn't concur that this was an issue. They didn't contest the recommendation and it seems their reason for leaving it out is that they were under the mistaken belief that a HEPA filter already has this capability.
- Why NASA can't rely on HEPA filters for the ESF recommendation to contain 100% of ultramicrobacteria
They labeled my preprint with these issues in it as nonsubstantive so didn't see the sections about issues with quarantine.
In my preprint I found the issue of quarantine after lab leaks, and the ESF recommendation seemed to have no solutions with currently available technology except possibly a first of its kind fully telerobotic laboratory.
My preprint included a suggestion to solve these issues in a simpler less costly way with a miniature life detection lab above GEO. I found this alternative would also achieve a higher level of assurance to keep Earth 100% safe (no appreciable risk) and that it was future-proof for any revision of the size limit or level of assurance.
Although my suggestion was not yet peer reviewed it was complete enough to suggest as a reasonable alternative for NASA to consider, which I did in the first round of public comments. Sadly NASA prescreened this suggestion out of consideration as nonsubstantive based on an out of date cite from 2008 that said we couldn't do adequate in situ life detection on Mars or in space.
NASA's team was unaware of its own Europa Lander mission, from 2016 to do life detection as far away as Jupiter. This was the main basis for my reasonable alternative and it cited it as one of its main sources but they never got to see that cite because they prescreened my paper without reading it.
When NASA published their draft PEIS I was astonished to see they had nobody on the team familiar with the planetary protection literature, which was immediately clear. For instance all four of their main arguments had already been refuted in 2000. My preprint covered this rebuttal under:
Zubrin's arguments in: "Contamination from Mars: No Threat" - not likely to be decisive in legal process - response of planetary protection experts in "No Threat? No Way"
(Walker, 2022, NASA and ESA are likely to be legally required to sterilize Mars samples to protect the environment until proven safe : 136)
I would never have guessed that NASA themselves would present these same four arguments in their own PEIS in 2022, as new arguments, original to them. This was not based on new research but based on many failings of scientific integrity, as we already saw for the Mars meteorite argument and for the other three, see above:
NASA's team showed no awareness in the PEIS that the Mars meteorite argument was rebutted even in their own cites.
I commented on the draft PEIS pointing out numerous very clear and serious flaws (Walker, 2022, Comment posted December 20th). Most are already covered in the attachment to my response during the first round of public comments. (Walker, 2022, Comment posted on May 16th) but I later found NASA labeled this as nonsubstantive and didn't read it.
My summary of these issues is in my attachment 7 for the second round of public comments, and is the only one NASA treated as substantive and responded to.
- (Walker, 2022, So many serious mistakes in NASA's Mars Samples Environmental Impact Statement it needs a clean restart) (docx))
I did minor updates after the PEIS was over. The latest preprint is here:
- (Walker, 2023. So many serious mistakes in NASA's Mars Samples Environmental Impact Statement it needs a clean restart) (docx))
NASA did read my attachment 7 and quote from it in their final PEIS but their team couldn't see that any of the issues I raised needed to be mentioned in the PEIS. From this it was clear there is nobody left on NASA's team familiar enough with the planetary protection literature any more to discuss this, so some other approach is needed.
Chester Everline, a co-author of NASA's handbook on probabilistic risk assurance (NASA, 2011, Probabilistic risk assessment procedures guide for NASA managers and practitioners) unusually commented on the last day of public comments (about the same time as my last comment). He found the PEIS didn’t state clearly what level of risk NASA is prepared to take for Earth’s biosphere and raised many other issues from the perspective of risk assurance, finally recommending that if NASA can't establish the Mars meteorite argument it should consider a deferred return until it has the information needed to assess risks better. See above:
I sent an email to Chester Everline to ask if we could liaise - combining his expertise in probabilistic risk assurance and my familiarity with the planetary protection literature. However, I was not surprised when he responded saying as a NASA employee he couldn’t engage.
I also contacted NASA's first planetary protection officer John Rummel. He is principal author, co-author, or contributor to nearly all the major studies on a Mars sample return. He just said he has retired and I should contact the planetary protection office (which has not replied). However John Rummel did also reassure me that someone would listen
I encourage you, however, to respond to NASA’s draft, highlighting the weaknesses that you have found. Somebody will listen - of that I am convinced - but I no longer work for NASA on this or any other project.
So there seems no potential for progress at this point through talking to the NASA team or others at NASA. If any do have issues with the PEIS they are likely to be in the same situation as Everline that as NASA employees they can't engage with me on the topic.
However, I hope somebody at NASA will listen at some point.
Making this book maximally accessible to neurodivergent people such as autistic people
As part of my voluntary work helping scared people over the internet, I am used to working with scared people, many of them autistic. I have learnt how to use simple and self contained sentence structures, that even quite severely autistic people can understand quickly when in the middle of a panic attack.
This is a “win win” situation as I find this approach usually makes the sentences shorter, with fewer words. It also seems to make the text easier for everyone to parse quickly. You may not notice much difference. The most obvious change may be that sentences and clauses within sentences tend to be a little shorter than for most academic papers. Also the use of short summary text on images and emphasis of occasional important points with bolding and red text. I have also done this page with pastel backgrounds for autistic people and I have worked on making it easier to navigate which helps everyone including autistic people.
I did a blog post on the difference between how autistic and non autistic people preferentially parse sentences which may help the reader understand the choices I make in sentence structure (Walker, R., n.d., Are you an orang utan or a chimpanzee? Common misunderstandings when talking about doomsday fears with people who are autistic, Aspergers, or very empathic and imaginative)
For some of the principles we can use to make text more accessible for neurodivergent people and those with various learning difficulties, see for instance (Advonet, 2021, Making information accessible for neurodivergent people) and (Change, n.d., How To Make Information Accessible). The language in this book is not simplified as much as for those examples, but I use the same principles, tested daily in communications with autistic people online..
[Supplementary information for the section: Note on use of language – this book is designed to be maximally accessible – by careful use of vocabulary and grammatical structures, but never with loss of precision in the meaning of the text]
My main strength is interdisciplinary
I am not associated with any institution. My training is in maths with a first class honours degree from York University in the 1970s, and I studied for a second undergraduate degree in philosophy also in the 1970s and did postgraduate study in the foundations of maths at Oxford under Robin Gandy (Wolfson College, 2019. Wolfson College salutes Robin Gandy on his centenary) in the 1980s.. Robin Gandy suggested I publish it as papers and after those papers were accepted for publication, to submit them to the university for a DSc, because of issues that arose to do with submitting my research as a thesis. That would have taken a fair bit of extra work. I haven't yet found the time to do that and so never completed my doctorate.
I have kept a keen interest in science, following the latest developments in science, astronomy and space exploration for half a century.
After my time at university, I worked as a self employed software developer selling my own software over the internet and I am now retired. However I wrote blog posts and I did answers on the Q/A site Quora which lead to some interest amongst astrobiologists. This lead to me being invited to a small astrobiology conference in Oxford in 2015 (Walker, 2015, "Super Positive" Outcomes For Search for Life In Enceladus and Europa Oceans). Also independently, an astrobiologist friend suggested I write an astrobiology paper for publication. That's when I started work on those various preprints.
My main strength in this topic is interdisciplinary. I can bring together results from the very wide range of disciplines relevant to planetary protection and sometimes find new connections between them that weren't noticed before in this vast field. A couple of examples:
- the work on the issues with lab leaks and quarantine for samples from a potentially alien biosphere is based on bringing together materials from public health experts, the history of the Apollo mission, synthetic biology (for mirror life) and many other disciplines.
- the work on the ESF size limit is a result of looking at biosafety lab requirements, air filter technology, the capabilities of filters, methods of maintaining and testing filters, and comparing those with the recommendation in the ESF report.
Much of this book is applied ethics, applied philosophy. There my philosophical background helps, also my religious background. My parents were both ordained missionaries in Madagascar (my mother belonged to a denomination that ordained female ministers and had female missionaries decades ago). As a child the house was full of books of theology and I read many of the as I was keen on the topic myself, and particularly interested in the cosmic optimism of the works of the Jesuit priest and anthropologist Teilhard de Chardin.
Into this combination of excitement and fear of annihilation, many people were looking for hope. One Catholic theologian who presented an optimistic view of the future was the Jesuit Scientist Teilhard de Chardin. Chardin presented a hopeful view of the technological progress that was exploding around him. Rather than dwelling on the dangers provided by nuclear power and space exploration, Chardin drew on his Christian faith to say that space travel was the final stage in the evolution of the universe towards greater consciousness. While Chardin had many critics, including the Magisterium of the Catholic Church, his views came to influence many in the West, including many Catholics.
(St Lawrence Institute, n.d., Jesus.... and Aliens? Part 1: The Question Arises)
Whether that happens or not, on the timescale of decades, I think it's clear we are headed towards more sustainability, respect for human rights, biorespect, etc. But likely many different faiths, philosophies and ideologies, rather than converging on one faith somehow. Planetary protection is also part of that I believe and part of a progression towards a biorespect of other lifeforms and other civilizations in our galaxy and eventually galaxy protection, biological protection and sustainable exploration of the entire galaxy.
I've also got a preprint related to the Fermi paradox, a sustainability solution following Sagan's approach, combined with planetary protection extended to galaxy protection, which as far as I know hasn't yet been used as a solution for the Fermi paradox (Robert Walker, 2021. Our pale blue dot - are there other homes for us in our galaxy?))
This preprint reasons that our universe is exactly what you'd expect if aliens start with planetary protection for their home planet, then their star system and then for the galaxy and realize they have to colonize a galaxy sustainably for their own protection as well as out of respect for biodiversity of the galaxy. I reason that this is how alien civilizations would likely mature. They would have the ability to have a major impact but they choose not to, just as we have the ability to use all the wood in the Amazon rainforest until it's gone - but choose not to and are moving to a sustainable future. This would explain why we see no large scale structures or radically altered galaxies anywhere - it would predict a minimal impact on the galaxy and mean they are hard to spot.
While any extraterrestrials that don't find a sustainable approach respecting biodiversity - and planetary protection - likely meet various major issues that prevent them spreading in space until eventually they do develop those capabilities - or else of course that they are unadventurous, -and not expansive. Many terrestrial animals are specialized., An extra -terrestrial civilization of rainforest frogs, say, might have little or no interest in trying to colonize a galaxy so long as their preferred habitat is secure. This is based on the sustainability solutions which go back to Carl Sagan's work on the Fermi paradox. It adds galaxy protection (including protecting themselves from their descendants)and planetary protection as an extra motive for sustainability.
In my final year of my philosophy degree I did an undergraduate thesis on the philosophy and theology of Albert Schweitzer, and his ideas of "Reverence for life", the philosopher, theologian and physician who worked in West Africa. (Barsam, 2008, Reverence for Life: Albert Schweitzer's Great Contribution to Ethical Thought).
For the last 40 years I have been a practicing Buddhist, in the Tibetan Nyingmapa tradition. So I also have a background in Buddhist ethics, not from books but from applying it in my life and going to many teachings by Buddhist teachings. We are encouraged to listen to teachings from any tradition so I have also listened to many teachings from other traditions when I was in Oxford, Buddhist and non Buddhist.
So I have a sound background in philosophy, ethics, I have a basic understanding of theology and Buddhism, and I have a strong background in maths and especially in the logical foundations of maths. That involves looking at logical reasoning at a level of detail that not even most mathematicians do. For instance most mathematicians doing geometry would be content with Euclid's axioms and not know about the axioms he left out such as the axiom that if a line enters a triangle through an edge or vertex it also has to leave it through another edge or vertex. Intuitively it follows from the other axioms but logically it doesn't. It is possible to construct a model of the other axioms where the axiom is invalid.
For an engineer or scientist to do practical applied ethics in a rigorous fashion, without training, is as impossible as for a philosopher to do science without training.
We all do intuitive ethics. To take a deliberately unrealistic example, if someone says they want to jump out of a plane at 10,000 feet (without a parachute) to see if they land uninjured, you can tell them it is foolish. You can give them the statistics that it is exceptionally unusual for anyone to survive a fall from 10,000 feet. You can raise ethical issues of unacceptable levels of risk. But you can't use science to prove that they shouldn't do it. In the end it is an ethical decision.
Hume referred to this as the is ought argument, that an 'ought' cannot be derived from an 'is'. This is a matter of a great deal of philosophical debate. This is how the Stanford Encyclopedia of Philosophy introduces it:
David Hume seemed to have these points in mind when he observed that an ‘ought’ cannot be derived from an ‘is. There is substantial debate about just what Hume meant, and similarly substantial debate as well about whether he was right. But at least part of Hume’s concern seems to have been that no set of claims about plain matters of fact (‘is’ claims) entail any evaluative claims (‘ought’ claims). He seems to have thought that one can infer the latter from the former only if, in addition to premises concerning plain matters of fact, one has on hand as well at least one evaluative premise. If, for instance, one infers from the fact that someone is feeling pain that something bad is happening, one is at least presupposing that pain is bad. That presupposition, in turn, is not entailed by any claims concerned solely with plain matters of fact. If Hume is right, every valid argument for an evaluative conclusion either includes or presupposes some evaluative premise. And, as a result, there is no value-neutral argument for an evaluative conclusion.
Coming at the same issues from a different direction, G. E. Moore argued (at the beginning of the twentieth century) that no naturalist account of morality could do justice to what we are actually thinking and claiming when we make moral judgments. Moore had in mind a variety of naturalist views that, at the time, had come to be seen as, on balance, the most attractive accounts of the nature of morality. While these views differed among themselves as to what goodness, rightness, virtue, and justice might consist in, they shared a commitment to seeing morality as a wholly natural phenomenon and they all saw moral judgment as a matter of thinking that actions, institutions, or characters had some particular natural property or other. According to these views, moral properties are to be identified with some natural property or other (e.g., with what is pleasant, or what satisfies someone’s desire, or what conforms to social rules that are in force).
(Stanford Encyclopedia of Philosophy, 2007, Metaethics : 4. Is/Ought and the Open Question Argument)
Hume's early 'is ought' distinction has lead to widespread philosophical debate about whether and to what extent ethics can be derived from natural properties of the world and other data such as social conventions, essentially derived using the scientific method for the natural sciences combined with the social sciences, or whether it is independent, cognitive, something that one has to develop through thinking through the ethics by oneself.
Whatever ones philosophical view, in practice ethics is something we can certainly not derive from the methods of science alone from physical properties and there is a lot of individual variation, as we see for instance in the variety of views on the acceptability of taking on a very low risk of unprecedented harm for Earth's entire biosphere, in the case of a Mars sample return.
In this way we all do practical ethics. What a philosophical background and logical background brings here is extra rigour in looking at this ethical reasoning in a kind of applied philosophy. In practice it is certainly not possible to derive ethical decisions in any absolute way, but it is possible to greatly increase the clarity of ethical debates and discussions and to firmly ground it in an evidence based approach to the scientific basis for any decision.
Then I have a sound background in scientific thinking. That's due to a keen interest in understanding not just how to do science but the basis of it, how it works. Although I didn't do a thesis in philosophy of science it was closely related to my thesis topic and a special interest of mine, and I did attend a postgraduate course in philosophy of science at Oxford university.
Then I have also had a keen interest in astronomy and science and have followed some of these topics since their beginnings, I've had a keen interest in astronomy since long before the Apollo moon landings.
The range of topics here is so vast, ethics, applied philosophy, astrobiology, maths, origins of life, air filter technology, microbial pathogens, synthetic biology, physiological responses to artificial gravity, medicine, public health.
Clearly I am not expert in any of these topics. What grounds this book is careful citing and checking of the cites. It is more than citing individual sentences. I also look at the context, looking at higher level, review level whenever it is available, and looking at the context and other papers on the topic and expert assessments of the reliability of the methods used to derive the conclusions.
So I am not expert in any of this. However, I rely on expert sources and am good at identifying reliable sources and summarizing / understanding what they say. I also frequently get feedback that I got it right when I contact experts. This will be checked of course with peer review. It will be quite a challenge for an academic publisher to find reviewers for this book assuming someone does accept the entire book for publication, because of the inevitable multidisciplinary approach used.
Citations list
[Citations list to go here]